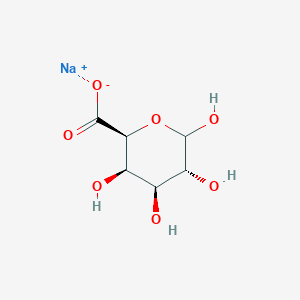
Sodium alginate
Descripción general
Descripción
Sodium alginate (SA) is a linear polysaccharide derived from brown seaweed (Phaeophyta), composed of β-D-mannuronic acid (M) and α-L-guluronic acid (G) residues linked by 1→4 glycosidic bonds . It is water-soluble, biocompatible, biodegradable, and exhibits excellent gel-forming capabilities via ionic crosslinking with divalent cations like Ca²⁺ . SA is widely used in:
- Food Industry: As a thickener, stabilizer, and gelling agent in ice cream, yogurt, and meat products .
- Pharmaceuticals: For controlled drug delivery, wound dressings, and tissue engineering due to its extracellular matrix-like structure .
- Environmental Science: In wastewater treatment for adsorbing heavy metals and phosphates .
Key properties include high viscosity, thermal stability, and tunable mechanical strength depending on the M/G ratio .
Métodos De Preparación
Extraction from Natural Sources
The extraction of sodium alginate from brown seaweed or kelp involves a series of steps to isolate and purify the polysaccharide from raw biomass. Traditional methods rely on chemical treatments, but recent advances incorporate enzymatic preprocessing to enhance yield and quality .
Pretreatment and Enzymatic Hydrolysis
Raw seaweed is washed to remove salts and debris, followed by soaking in formaldehyde to fix pigments. The material is then treated with a complex enzyme solution containing cellulase, pectinase, and protease (0.5–2% w/w) at 40–50°C for 2–4 hours . Enzymatic hydrolysis breaks down cell walls, releasing alginate into solution while minimizing degradation of its polymer chains. This step increases extraction efficiency by 15–20% compared to non-enzymatic methods .
Alkaline Digestion and Precipitation
The enzymatically treated seaweed is digested in sodium carbonate solution (2–4% w/v) at 60–70°C for 2–3 hours to convert insoluble alginic acid salts into soluble this compound . The filtrate is acidified with hydrochloric acid to pH 2–3, precipitating alginic acid, which is then neutralized with sodium hydroxide to yield this compound. Ethanol precipitation is employed to further purify the product, resulting in a final viscosity of 500–800 mPa·s and a yield of 25–30% .
Chemical Synthesis Methods
Chemical synthesis focuses on purifying alginic acid derived from natural sources or synthetic pathways. A patented method highlights the neutralization of alginic acid with sodium hydroxide (5–50% concentration) to produce high-purity this compound .
Neutralization and Impurity Removal
Alginic acid is dissolved in sodium hydroxide under controlled pH conditions (7.0–7.5). Activated carbon (1:6 ratio relative to alginic acid) is added to adsorb impurities such as proteins and pigments for 5–20 minutes . Vacuum filtration (−0.04 to −0.1 MPa pressure) removes the carbon-alginate mixture, yielding a translucent filtrate.
Ethanol Precipitation and Drying
Ethanol is added to the filtrate to precipitate this compound, which is then vacuum-dried at 30–100°C for 0.3–4 hours . This method achieves a purity exceeding 98% with minimal residual ash content (<0.5%), making it suitable for pharmaceutical applications .
Microfluidic Production Techniques
Microfluidic systems enable precise control over this compound particle size and morphology, particularly for drug delivery and cell encapsulation .
Droplet Generation and Gelation
Alginate solution is introduced into a microfluidic chip alongside a calcium ion source (e.g., CaCl₂ or Ca-EDTA complexes). At the junction of aqueous and oil phases, droplets form via shear forces, with sizes tunable from 10 µm to 1 mm . A novel approach employs calcium-EDTA chelates, where acetic acid triggers ion release post-droplet formation, ensuring homogeneous crosslinking and preventing channel clogging .
Monodisperse Particle Fabrication
Utech et al. demonstrated a method generating monodisperse microparticles (CV < 5%) using capillary-based devices . By separating droplet formation and gelation, this technique achieves structural uniformity and encapsulation efficiencies exceeding 90% for mammalian cells .
Comparative Analysis of Preparation Methods
The table below summarizes key parameters of this compound production techniques:
Method | Yield | Purity | Viscosity (mPa·s) | Scalability | Cost |
---|---|---|---|---|---|
Enzymatic Extraction | 25–30% | 85–90% | 500–800 | Moderate | Medium |
Chemical Synthesis | >98% | >98% | 200–400 | High | Low |
Microfluidics | 70–80% | 95–98% | Customizable | Low | High |
Yield and Purity Trade-offs
Enzymatic extraction sacrifices purity for higher molecular weight and viscosity, ideal for food additives . Chemical synthesis prioritizes purity but may reduce viscosity due to polymer degradation during neutralization . Microfluidics balances both but faces scalability challenges .
Industrial Applicability
Chemical synthesis dominates industrial production due to low cost and high throughput . Microfluidic methods remain niche, reserved for high-value applications like targeted drug delivery .
Recent Advances in Preparation Methods
Hybrid Enzymatic-Chemical Processes
Combining enzymatic pretreatment with ethanol precipitation reduces organic solvent use by 30% while maintaining yields above 25% .
3D-Printed Microfluidic Chips
Customizable 3D-printed chips enable rapid prototyping of alginate microgels with complex geometries (e.g., Janus particles) . These systems reduce production time from hours to minutes but require significant upfront investment .
Análisis De Reacciones Químicas
Cross-Linking with Divalent Cations
The most characteristic reaction involves ionic cross-linking with Ca²⁺ ions through the "egg-box" model. Studies demonstrate:
Parameter | Calcium Alginate Gel | Sodium Alginate Solution |
---|---|---|
Bond type | Ionic (Ca²⁺-COO⁻) | Electrostatic (Na⁺-COO⁻) |
Solubility | Insoluble | Water-soluble |
Gel strength | 50-200 kPa | N/A |
Source 3 reveals gel formation occurs within 50-200 ms upon contact with CaCl₂ solutions, influenced by:
-
M/G ratio : Laminaria species (M/G = 2.26) form weaker gels than Sargassum (M/G = 0.8-1.5) due to reduced G-block availability
-
Concentration : 2% this compound solutions achieve optimal cross-link density with 0.5 M CaCl₂
Oxidation Reactions
Periodate oxidation introduces aldehyde groups via C2-C3 bond cleavage (Scheme 1):
Table 1 : Oxidation efficiency with NaIO₄ (24h reaction)
NaIO₄ (mol%) | Oxidation Yield (%) | Mw Reduction (%) |
---|---|---|
5 | 98 | 42 |
25 | 99 | 78 |
50 | 98 | 92 |
Key findings:
Acid-Base Reactions
Protonation of carboxyl groups alters solubility:
DSC analysis shows endothermic peak at 120°C for alginic acid vs. 85°C for this compound, indicating stronger hydrogen bonding in protonated form .
Redox Reactions in Alginate Matrices
Encapsulated redox systems demonstrate unique kinetics:
-
(acidic activation)
-
(decolorization in 8 min)
Comproportionation reaction :
(blue coloration in 30-60 s)
Chemical Modifications
-
Reactant ratio: H₃PO₄:urea = 1:70
-
Results: 2-4× Mw reduction, exclusive G-unit modification
-
SO₃·Py complex reaction
-
Forms C2/C3 sulfate esters
-
Mw decreases from 100 kDa → 10 kDa
Enzymatic and Thermal Degradation
Physical Interactions
Molecular dynamics simulations reveal :
-
Na⁺ coordination distance: 2.3 Å (O-Na)
-
H-bond network density: 3.2 bonds/nm³ in alginic acid vs. 1.8 bonds/nm³ in this compound
These reactions enable tailored material properties for biomedical, food, and environmental applications. Recent advances focus on controlling reaction kinetics through M/G ratio manipulation (source 1) and oxidation degree optimization (source 5), opening new frontiers in smart hydrogel development.
Aplicaciones Científicas De Investigación
Food Industry
Thickening and Gelling Agent
Sodium alginate is widely used in the food industry as a thickening agent, gelling agent, emulsifier, stabilizer, and texture improver. Its ability to form gels in the presence of calcium ions makes it ideal for creating various food products such as jellies, sauces, and dairy items .
Encapsulation
In food processing, this compound is utilized for encapsulating flavors, nutrients, and probiotics. This encapsulation helps in protecting sensitive ingredients from degradation while allowing for controlled release during digestion .
Biomedical Applications
Drug Delivery Systems
this compound-based hydrogels have emerged as promising candidates for drug delivery systems. They can encapsulate proteins and other therapeutic agents under mild conditions that minimize denaturation. The hydrogels protect these agents from degradation until their release in the body .
Tissue Engineering
Alginate gels serve as scaffolds in tissue engineering due to their biocompatibility and ability to support cell growth. They can be tailored for both 2D and 3D cell culture systems, allowing researchers to study cellular behaviors in physiologically relevant conditions .
Submucosal Injection Material
this compound has been investigated as a submucosal injection material for endoscopic procedures. Studies have shown that it can create a durable submucosal cushion that enhances lesion-lifting properties during endoscopic resections .
Environmental Applications
Water Treatment
this compound's biodegradable nature makes it suitable for use in water treatment processes. It can be formulated into hydrogels and films that effectively remove contaminants from water while being environmentally friendly .
Soil Stabilization
Recent research has highlighted this compound's potential as an eco-friendly soil stabilizer. When mixed with soil, it enhances shear strength and reduces erosion by forming a matrix within the soil pores .
Case Studies
Mecanismo De Acción
Sodium alginate exerts its effects primarily through its ability to form gels and interact with various ions. When ingested, it can form a gel-like raft in the stomach, which helps to suppress gastric reflux by creating a physical barrier . In biomedical applications, its gel-forming ability is utilized for controlled drug release and tissue engineering .
Comparación Con Compuestos Similares
Calcium Alginate
- Solubility : Insoluble in water but forms hydrogels via Ca²⁺ crosslinking .
- Gelation Mechanism : Calcium ions create an "egg-box" structure with G-blocks, resulting in rigid, heat-stable gels .
- Applications :
- Mechanical Properties : Higher gel strength compared to SA, but brittle due to dense crosslinking .
Potassium Alginate
- Solubility : Water-soluble, similar to SA .
- Gelation : Requires divalent cations (e.g., Ca²⁺) but less commonly used than SA .
- Applications: Food thickener in low-sodium diets . Limited pharmaceutical use due to SA's dominance.
Ammonium Alginate
- Solubility : Water-soluble .
- Gelation : Forms gels with Ca²⁺ but less studied than SA or calcium alginate.
- Applications : Primarily industrial, such as textile printing and paper coatings .
Alginic Acid
- Solubility : Insoluble in water but swells in acidic conditions (pH < 3.5) .
- Gelation : Forms acid gels at low pH, used in antacid formulations .
- Applications :
Data Tables
Table 1: Comparative Properties of Alginate Salts
Property | Sodium Alginate | Calcium Alginate | Potassium Alginate | Alginic Acid |
---|---|---|---|---|
Solubility | Water-soluble | Insoluble | Water-soluble | Swells in acid |
Gelation Trigger | Ca²⁺, Ba²⁺ | Ca²⁺ | Ca²⁺ | Low pH (<3.5) |
Gel Strength | Moderate | High (rigid) | Low | Moderate |
Primary Applications | Food, pharma, adsorpt | Wound care, encapsulation | Food thickener | Supplements, antacids |
Cost | Low | Moderate | Moderate | Low |
Table 2: Impact of M/G Ratio on Alginate Properties
M/G Ratio | Chain Flexibility | Gel Strength | Preferred Application |
---|---|---|---|
High M | High | Low | Flexible films, drug delivery |
High G | Low | High | Rigid gels, wound dressings |
Research Findings
Structural and Mechanical Differences
- SA vs. Calcium Alginate : Molecular dynamics simulations show calcium alginate forms denser aggregates due to stronger ionic interactions, whereas SA remains soluble until crosslinked . Guluronate-rich SA (e.g., from Laminaria) produces stiffer gels than mannuronate-rich SA (e.g., from Fucus) .
- Reinforcement with Nanoparticles: Adding graphene oxide (GO) at 0.1% w/w increases SA’s compressive strength by 40% . Titanium dioxide (TiO₂) enhances SA’s dielectric constant (ε′ = 5.2 at 5 wt%) for electronic applications .
Functional Performance
Actividad Biológica
Sodium alginate is a natural polysaccharide derived from the cell walls of brown seaweeds, primarily belonging to the family Phaeophyceae. It has garnered significant attention in various fields, including medicine, food science, and agriculture, due to its unique biological activities and properties. This article delves into the biological activities of this compound, highlighting its therapeutic potentials, mechanisms of action, and applications based on diverse research findings.
Overview of this compound
This compound is a linear copolymer composed of two types of uronic acid residues: mannuronic acid (M) and guluronic acid (G) . The ratio of these residues (M/G ratio) influences its physicochemical properties and biological activities. This compound is known for its ability to form gels in the presence of divalent cations like calcium, which is crucial for its applications in drug delivery systems, tissue engineering, and as a food additive.
Biological Activities
This compound exhibits a wide range of biological activities:
- Anti-tumor Activity : Research indicates that this compound and its oligosaccharides can inhibit tumor growth. A study showed that high-viscosity alginate significantly suppressed the growth of sarcoma 180 cells in mice compared to low-viscosity alginate . The anti-tumor effects are believed to be linked to the modulation of immune responses.
- Immunomodulatory Effects : this compound has been shown to enhance immune responses by inducing cytokine production in macrophages. For instance, alginate oligosaccharides can stimulate the synthesis of pro-inflammatory cytokines like TNF-α and IL-6 . This immunomodulatory effect is crucial for its potential use in cancer therapies and vaccine adjuvants.
- Antioxidant Properties : this compound demonstrates antioxidant activity by scavenging free radicals, which can help mitigate oxidative stress-related diseases .
- Gastroprotective Effects : this compound is widely used in treating gastroesophageal reflux disease (GERD) due to its ability to form a viscous gel that protects the gastric mucosa from acid damage. Clinical studies have shown that this compound significantly reduces symptoms like epigastric pain and acid regurgitation following endoscopic procedures .
- Antibacterial Activity : this compound exhibits antibacterial properties against various pathogens, making it a candidate for applications in wound healing and infection control .
- Low Molecular Weight Alginate : Recent studies have highlighted the benefits of low molecular weight this compound (VLVG) in reducing liver inflammation and improving survival rates in animal models undergoing surgical procedures . This suggests that different molecular weights can have distinct biological effects.
The biological activities of this compound are mediated through several mechanisms:
- Cytokine Induction : Alginate oligosaccharides activate macrophages through the NF-κB signaling pathway, leading to increased cytokine production .
- Gel Formation : The gel-forming ability of this compound not only provides physical protection but also creates a sustained release system for drugs or bioactive compounds.
- Interaction with Cell Membranes : this compound can interact with cell membranes, enhancing cellular uptake of therapeutic agents.
Case Study 1: Immunomodulation in Cancer Therapy
A study investigated the effects of this compound on RAW264.7 macrophages, revealing that it induced significant cytokine production (e.g., IL-12, TNF-α) at specific concentrations (3 mg/mL). This suggests its potential as an immunotherapeutic agent in cancer treatment .
Case Study 2: Gastroprotective Effects
In a randomized clinical trial involving patients undergoing endoscopic biopsy, those treated with this compound reported significant reductions in post-procedural discomfort compared to controls. This highlights its efficacy as a gastroprotective agent .
Applications
The diverse biological activities of this compound enable its use across various industries:
Application Area | Description |
---|---|
Pharmaceuticals | Used as a drug delivery system due to its gel-forming properties. |
Food Industry | Acts as a thickening agent and stabilizer; enhances texture and shelf life. |
Agriculture | Used as a soil conditioner and plant growth regulator due to its water retention capabilities. |
Cosmetics | Incorporated into formulations for its moisturizing properties. |
Medical Devices | Utilized in wound dressings for its biocompatibility and antibacterial effects. |
Q & A
Q. What are the standard methods for characterizing sodium alginate in experimental studies?
Level: Basic
Answer: Characterization should include molecular weight analysis (via gel permeation chromatography), monomer composition (mannuronate/guluronate ratio via NMR or FTIR), viscosity measurements, and purity assessment (e.g., ash content, heavy metal testing). For publication, journals like Carbohydrate Polymers require detailed specifications such as degree of esterification or cross-linking . Structural confirmation through techniques like XRD or SEM is also recommended for modified alginate materials .
Q. How can response surface methodology (RSM) be applied to optimize this compound-based formulations?
Level: Advanced
Answer: RSM, particularly Box-Behnken or central composite designs, allows efficient optimization of variables (e.g., polymer concentration, cross-linker ratio). For example, in film formulation, independent variables like this compound concentration and CaCl₂ immersion time can be modeled against responses such as water vapor permeability or gel strength . Software tools (Design Expert®) enable polynomial equation fitting to predict optimal conditions while minimizing experimental runs .
Q. What experimental designs are suitable for studying this compound in drug delivery systems?
Level: Advanced
Answer: Factorial designs (e.g., simplex lattice) are effective for multi-variable systems. For ion-activated gels, variables like this compound, gellan gum, and HPMC concentrations can be tested against drug release profiles and rheological properties . Replicated center points in designs (e.g., Box-Behnken) improve reliability by accounting for variability .
Q. What statistical approaches are recommended for analyzing this compound concentration-response data?
Level: Basic
Answer: Linear regression models are suitable for dose-response trends (e.g., encapsulation effects on plant growth ). For non-normal data, Shapiro-Wilk tests followed by non-parametric analyses (Kruskal-Wallis) are advised. ANOVA with post-hoc Tukey tests can compare means across concentrations, while multivariate analysis handles interactions between variables (e.g., alginate-CMC blends) .
Q. How to address contradictions in studies on this compound's biological effects?
Level: Advanced
Answer: Contradictions (e.g., growth inhibition vs. enhancement) may arise from species-specific responses or concentration thresholds. Meta-analysis of variables like alginate molecular weight, exposure duration, and environmental conditions (pH, ionic strength) is critical. For example, A. latus fish studies showed growth benefits at specific doses, while sugarcane bud chips exhibited inhibition at higher concentrations . Replication under standardized protocols is essential .
Q. What methodologies are effective for synthesizing stable silver nanoparticles (AgNPs) using this compound?
Level: Advanced
Answer: this compound acts as a dual reducing/stabilizing agent. Key parameters include alginate concentration (0.5–2% w/v), AgNO₃ molarity, and reaction time. UV-Vis spectroscopy monitors plasmon resonance (∼400 nm), while TEM confirms nanoparticle size (10–50 nm). Cross-linking with Ca²⁺ enhances colloidal stability, and antibacterial efficacy can be validated via MIC assays .
Q. What are key considerations for in vivo studies evaluating this compound's biocompatibility?
Level: Basic
Answer: Follow ethical guidelines (e.g., Basel Declaration ). Control variables include alginate purity (endotoxin levels), administration route (oral vs. injectable), and degradation kinetics. Histopathological analysis and immune markers (e.g., cytokine levels) should assess acute/chronic toxicity. Studies on A. latus combined growth metrics with mucus immunity profiling .
Q. How to enhance mechanical properties of this compound hydrogels for biomedical applications?
Level: Advanced
Answer: Cross-linking with Ca²⁺ forms an "egg-box" structure, improving tensile strength . Blending with synthetic polymers (e.g., PVA) or nanomaterials (cellulose nanocrystals) enhances elasticity . Advanced methods like click chemistry or graft copolymerization can introduce covalent bonds for long-term stability .
Q. What strategies mitigate batch-to-batch variability in this compound sources?
Level: Advanced
Answer: Source alginate from consistent algal species (e.g., Laminaria hyperborea) and standardize extraction protocols. Pre-screening via viscosity and monomer ratio analysis ensures uniformity . Collaborative databases documenting material properties (e.g., CAS 9005-38-3 specifications) aid reproducibility .
Q. What are best practices for documenting this compound modifications in publications?
Level: Basic
Answer: Report modification methods (e.g., esterification, amidation) with reaction conditions (temperature, pH, catalysts). Include spectral data (FTIR/NMR) for new derivatives and mechanical testing (rheometry, tensile tests) for hydrogels . Journals like Beilstein Journal of Organic Chemistry require explicit experimental details for replication .
Propiedades
Número CAS |
9005-38-3 |
---|---|
Fórmula molecular |
C6H9NaO7 |
Peso molecular |
216.12 g/mol |
Nombre IUPAC |
sodium;(2S,3R,4S,5R)-3,4,5,6-tetrahydroxyoxane-2-carboxylate |
InChI |
InChI=1S/C6H10O7.Na/c7-1-2(8)4(5(10)11)13-6(12)3(1)9;/h1-4,6-9,12H,(H,10,11);/q;+1/p-1/t1-,2+,3+,4-,6?;/m0./s1 |
Clave InChI |
MSXHSNHNTORCAW-KSSASCOMSA-M |
SMILES |
C1(C(C(OC(C1O)O)C(=O)[O-])O)O.[Na+] |
SMILES isomérico |
[C@@H]1([C@H]([C@H](OC([C@@H]1O)O)C(=O)[O-])O)O.[Na+] |
SMILES canónico |
C1(C(C(OC(C1O)O)C(=O)[O-])O)O.[Na+] |
Color/Form |
Cream-colored powder Filamentous or granular solid or powde |
Descripción física |
Dry Powder Cream-colored solid; [Merck Index] Yellow-brown powder; [MSDSonline] |
Solubilidad |
Insol in alc, chloroform, ether, aq acid soln below ph 3, and in hydro-alcoholic soln greater than 30% wt/wt Sol in water forming viscous, colloidal soln |
Sinónimos |
alginate Alginate, Barium Alginate, Calcium Alginate, Copper Alginate, Potassium Alginate, Sodium Alginate, Sodium Calcium Alginates alginic acid, barium salt alginic acid, calcium salt alginic acid, copper salt alginic acid, potassium salt alginic acid, sodium salt Alloid G barium alginate calcium alginate Calcium Alginate, Sodium calginat copper alginate Kalrostat Kalrostat 2 Kaltostat poly(mannuronic acid), sodium salt potassium alginate sodium alginate sodium calcium alginate Vocoloid Xantalgin |
Origen del producto |
United States |
Retrosynthesis Analysis
AI-Powered Synthesis Planning: Our tool employs the Template_relevance Pistachio, Template_relevance Bkms_metabolic, Template_relevance Pistachio_ringbreaker, Template_relevance Reaxys, Template_relevance Reaxys_biocatalysis model, leveraging a vast database of chemical reactions to predict feasible synthetic routes.
One-Step Synthesis Focus: Specifically designed for one-step synthesis, it provides concise and direct routes for your target compounds, streamlining the synthesis process.
Accurate Predictions: Utilizing the extensive PISTACHIO, BKMS_METABOLIC, PISTACHIO_RINGBREAKER, REAXYS, REAXYS_BIOCATALYSIS database, our tool offers high-accuracy predictions, reflecting the latest in chemical research and data.
Strategy Settings
Precursor scoring | Relevance Heuristic |
---|---|
Min. plausibility | 0.01 |
Model | Template_relevance |
Template Set | Pistachio/Bkms_metabolic/Pistachio_ringbreaker/Reaxys/Reaxys_biocatalysis |
Top-N result to add to graph | 6 |
Feasible Synthetic Routes
Descargo de responsabilidad e información sobre productos de investigación in vitro
Tenga en cuenta que todos los artículos e información de productos presentados en BenchChem están destinados únicamente con fines informativos. Los productos disponibles para la compra en BenchChem están diseñados específicamente para estudios in vitro, que se realizan fuera de organismos vivos. Los estudios in vitro, derivados del término latino "in vidrio", involucran experimentos realizados en entornos de laboratorio controlados utilizando células o tejidos. Es importante tener en cuenta que estos productos no se clasifican como medicamentos y no han recibido la aprobación de la FDA para la prevención, tratamiento o cura de ninguna condición médica, dolencia o enfermedad. Debemos enfatizar que cualquier forma de introducción corporal de estos productos en humanos o animales está estrictamente prohibida por ley. Es esencial adherirse a estas pautas para garantizar el cumplimiento de los estándares legales y éticos en la investigación y experimentación.