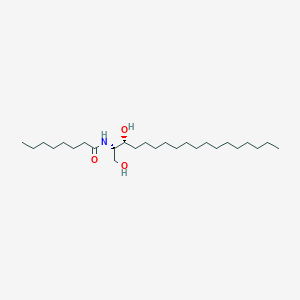
DIHIDROCERAMIDA C8
Descripción general
Descripción
N-[(2S,3R)-1,3-dihydroxyoctadecan-2-yl]octanamide is a ceramide, a type of lipid molecule that plays a crucial role in cellular signaling and structure. Ceramides are found in high concentrations within the cell membrane of eukaryotic cells and are involved in various cellular processes, including apoptosis (programmed cell death), cell differentiation, and proliferation .
Aplicaciones Científicas De Investigación
Cancer Therapeutics
Mechanism of Action:
C8 dihydroceramide has been shown to induce apoptosis in cancer cells, particularly in non-small-cell lung cancer (NSCLC) models. A study demonstrated that C8-ceramide treatment resulted in increased levels of reactive oxygen species (ROS), leading to cell cycle arrest and apoptosis in H1299 lung cancer cells. The compound modulates the expression of superoxide dismutases (SOD1 and SOD2), influencing cell survival pathways and promoting anti-proliferative effects .
Case Study:
In a specific experiment, H1299 cells treated with C8 dihydroceramide exhibited a dose-dependent decrease in proliferation rates, with an IC50 value of 22.9 µM. Flow cytometry analysis indicated that higher concentrations caused significant accumulation of cells in the G1 phase and increased apoptotic markers such as cleaved caspase-3 .
Metabolic Disorders
Role in Cardiometabolic Health:
Recent research has identified a link between dihydroceramides, including C8 dihydroceramide, and the risk of developing type 2 diabetes (T2D) and cardiovascular diseases (CVD). A profiling study indicated that certain dihydroceramides are associated with increased risks for these conditions. Specifically, C8 dihydroceramide was implicated in metabolic alterations that precede disease onset, suggesting its potential as a biomarker for assessing cardiometabolic risk .
Data Table: Dihydroceramide Associations with T2D and CVD Risk
Dihydroceramide | Hazard Ratio (HR) for T2D | Hazard Ratio (HR) for CVD |
---|---|---|
dhCer20:0 | 1.32 (1.08, 1.63) | – |
dhCer22:2 | 1.32 (1.07, 1.62) | 1.55 (1.23, 1.94) |
This table summarizes significant findings from the EPIC-Potsdam cohort study regarding the association of specific dihydroceramides with T2D and CVD risk .
Immunological Functions
Immunomodulatory Effects:
C8 dihydroceramide has been shown to enhance immune responses by stimulating dendritic cells during viral infections. This property suggests its potential use in developing immunotherapies or vaccines . Additionally, it has been observed to mimic the effects of gamma-tocopherol on endoplasmic reticulum (ER) stress induction and downregulation of pro-inflammatory pathways involving NF-κB .
Biophysical Properties
Membrane Dynamics:
Research indicates that dihydroceramides like C8 can influence membrane stability and dynamics due to their structural properties. They facilitate the formation of rigid domains within biological membranes, potentially altering cellular signaling pathways and membrane interactions . This characteristic could be leveraged in drug delivery systems where membrane integrity is crucial.
Mecanismo De Acción
C8 Dihydroceramide, also known as n-octanoyldihydrosphingosine, N-[(2S,3R)-1,3-dihydroxyoctadecan-2-yl]octanamide, or N-OCTANOYLSPHINGANINE, is a type of sphingolipid that has been gaining attention for its emerging physiological roles and functions .
Target of Action
C8 Dihydroceramide primarily targets the de novo sphingolipid synthesis pathway . This pathway is an evolutionarily conserved process that takes place in the endoplasmic reticulum (ER) and is responsible for the production of sphingolipids, a major class of membrane lipids that also function as essential signaling molecules .
Mode of Action
C8 Dihydroceramide is a metabolic intermediate in the de novo sphingolipid synthesis pathway . It is converted into ceramides (Cers) with the addition of a double bond . This conversion is catalyzed by two distinct dihydroceramide desaturases (DES1 and DES2), with DES1 accounting for ceramide synthesis in most tissues .
Biochemical Pathways
C8 Dihydroceramide and the product ceramide are substrates for the generation of more complex sphingolipids in the Golgi apparatus . These include dihydrosphingomyelin (DhSM) or dihydroglucosylceramides/dihydrogangliosides and sphingomyelin (SM) or glucosylceramides/gangliosides .
Pharmacokinetics
It is known that dihydroceramides accumulate to a far greater extent in tissues than previously thought .
Result of Action
C8 Dihydroceramide has been implicated in various biological processes distinct from those involving ceramides . These processes include cellular stress responses and autophagy, cell growth, pro-death or pro-survival pathways, hypoxia, and immune responses . In addition, their plasma concentration has been related to metabolic diseases and shown as a long-term predictor of type 2 diabetes onset .
Action Environment
The action of C8 Dihydroceramide can be influenced by various environmental factors. For instance, the inhibition of DES1 activity elevates DhCer and DhSph levels, leading to cellular stress, autophagy, and early apoptosis
Análisis Bioquímico
Biochemical Properties
C8 Dihydroceramide is a metabolic intermediate of the de novo sphingolipid synthesis pathway . It interacts with ceramide synthase (CerS), which gives rise to dihydroceramide with varying acyl chain lengths . These interactions are crucial for the production of more complex sphingolipids .
Cellular Effects
C8 Dihydroceramide has been found to play roles in cellular stress responses, autophagy, cell growth, pro-death or pro-survival pathways, hypoxia, and immune responses . Its plasma concentration has been related to metabolic diseases and shown as a long-term predictor of type 2 diabetes onset .
Molecular Mechanism
The molecular mechanism of C8 Dihydroceramide involves the insertion of a double bond into dihydroceramide to convert it to ceramide . This process is catalyzed by two distinct dihydroceramide desaturases (DES1 and DES2) . The inhibition of DES1 activity elevates C8 Dihydroceramide levels, leading to cellular stress, autophagy, and early apoptosis .
Metabolic Pathways
C8 Dihydroceramide is involved in the de novo sphingolipid biosynthesis pathway . It’s converted into ceramides with the addition of a double bond, a process that takes place in the endoplasmic reticulum (ER) . The ceramides are then substrates for the generation of more complex sphingolipids .
Subcellular Localization
The de novo sphingolipid biosynthesis, which involves the production of C8 Dihydroceramide, takes place in the endoplasmic reticulum (ER)
Métodos De Preparación
Synthetic Routes and Reaction Conditions
The synthesis of N-[(2S,3R)-1,3-dihydroxyoctadecan-2-yl]octanamide typically involves the reaction of sphinganine with octanoyl chloride in the presence of a base such as pyridine. The reaction is carried out under anhydrous conditions to prevent hydrolysis of the acyl chloride. The product is then purified using column chromatography.
Industrial Production Methods
Industrial production of N-[(2S,3R)-1,3-dihydroxyoctadecan-2-yl]octanamide involves large-scale synthesis using similar methods as in the laboratory but optimized for higher yields and purity. This includes the use of automated reactors and continuous flow systems to ensure consistent reaction conditions and efficient purification processes.
Análisis De Reacciones Químicas
Types of Reactions
N-[(2S,3R)-1,3-dihydroxyoctadecan-2-yl]octanamide undergoes various chemical reactions, including:
Oxidation: The hydroxyl groups can be oxidized to ketones or carboxylic acids using oxidizing agents such as potassium permanganate or chromium trioxide.
Reduction: The amide group can be reduced to an amine using reducing agents like lithium aluminum hydride.
Substitution: The hydroxyl groups can be substituted with other functional groups using reagents like tosyl chloride or mesyl chloride.
Common Reagents and Conditions
Oxidation: Potassium permanganate in acidic or basic conditions.
Reduction: Lithium aluminum hydride in anhydrous ether.
Substitution: Tosyl chloride or mesyl chloride in the presence of a base such as triethylamine.
Major Products Formed
Oxidation: Ketones or carboxylic acids.
Reduction: Amines.
Substitution: Tosylates or mesylates.
Comparación Con Compuestos Similares
Similar Compounds
- N-[(2S,3R)-1,3-dihydroxyoctadecan-2-yl]pentacosanamide
- N-[(2S,3R)-1,3-dihydroxyoctadecan-2-yl]butanamide
- N-[(2S,3R)-1,3-dihydroxyoctadecan-2-yl]formamide
Uniqueness
N-[(2S,3R)-1,3-dihydroxyoctadecan-2-yl]octanamide is unique due to its specific fatty acid chain length and hydroxyl group configuration, which influence its physical properties and biological activity. Compared to other ceramides, it has distinct effects on cellular signaling pathways and membrane structure, making it a valuable compound for research and industrial applications .
Actividad Biológica
C8 Dihydroceramide (C8-dhCer) is a sphingolipid that has garnered attention in the field of cell biology due to its role as a substrate for dihydroceramide desaturase (DES), an enzyme that converts dihydroceramides into ceramides. This article provides a detailed overview of the biological activity of C8 Dihydroceramide, including its metabolic pathways, implications in disease, and experimental findings from recent studies.
Overview of Dihydroceramides
Dihydroceramides are precursors to ceramides, which are crucial components of cell membranes and play significant roles in cellular signaling, apoptosis, and cell differentiation. While ceramides are often recognized for their bioactive properties, dihydroceramides like C8-dhCer have traditionally been considered biologically inactive. However, recent research suggests that they may have indirect effects on cellular processes through their metabolic conversion to ceramides.
Metabolism and Enzymatic Activity
C8 Dihydroceramide serves as a substrate for dihydroceramide desaturase (DES), which catalyzes the introduction of a double bond into the sphingolipid backbone. The enzymatic conversion of C8-dhCer to ceramide involves several key parameters:
- Kinetic Parameters :
- for C8-dhCer: 1.92 ± 0.36 μM
- : 3.16 ± 0.24 nmol/min/g protein
These values indicate the efficiency of DES in metabolizing C8-dhCer, highlighting its potential role in regulating ceramide levels in cells .
Role in Disease
Emerging evidence links dihydroceramides to metabolic disorders such as Type 2 Diabetes (T2D) and cardiovascular diseases (CVD). A study utilizing genome-wide association analyses found that certain dihydroceramides were associated with increased risks for these conditions. Specifically:
- Dihydroceramide Associations :
- Higher T2D Risk : dhCer20:0 and dhCer22:2
- Higher CVD Risk : dhCer22:2
These findings suggest that dihydroceramides may mediate the effects of dietary habits on disease risk, indicating a complex interplay between lipid metabolism and health outcomes .
In Vitro Studies
- Desaturase Activity Assays :
- Cell Viability and Function :
Case Studies
Several case studies have illustrated the implications of dihydroceramide metabolism in cancer biology and metabolic disorders:
- Case Study 1 : In human neuroblastoma cells treated with fenretinide, an inhibitor of DES, researchers observed altered desaturation rates of dihydroceramides, leading to decreased ceramide levels and subsequent effects on cell cycle progression .
- Case Study 2 : A cohort study linked plasma levels of specific dihydroceramides to T2D risk factors, demonstrating how dietary intake influences lipid profiles and disease outcomes .
Propiedades
IUPAC Name |
N-[(2S,3R)-1,3-dihydroxyoctadecan-2-yl]octanamide | |
---|---|---|
Source | PubChem | |
URL | https://pubchem.ncbi.nlm.nih.gov | |
Description | Data deposited in or computed by PubChem | |
InChI |
InChI=1S/C26H53NO3/c1-3-5-7-9-10-11-12-13-14-15-16-18-19-21-25(29)24(23-28)27-26(30)22-20-17-8-6-4-2/h24-25,28-29H,3-23H2,1-2H3,(H,27,30)/t24-,25+/m0/s1 | |
Source | PubChem | |
URL | https://pubchem.ncbi.nlm.nih.gov | |
Description | Data deposited in or computed by PubChem | |
InChI Key |
LGOFBZUQIUVJFS-LOSJGSFVSA-N | |
Source | PubChem | |
URL | https://pubchem.ncbi.nlm.nih.gov | |
Description | Data deposited in or computed by PubChem | |
Canonical SMILES |
CCCCCCCCCCCCCCCC(C(CO)NC(=O)CCCCCCC)O | |
Source | PubChem | |
URL | https://pubchem.ncbi.nlm.nih.gov | |
Description | Data deposited in or computed by PubChem | |
Isomeric SMILES |
CCCCCCCCCCCCCCC[C@H]([C@H](CO)NC(=O)CCCCCCC)O | |
Source | PubChem | |
URL | https://pubchem.ncbi.nlm.nih.gov | |
Description | Data deposited in or computed by PubChem | |
Molecular Formula |
C26H53NO3 | |
Source | PubChem | |
URL | https://pubchem.ncbi.nlm.nih.gov | |
Description | Data deposited in or computed by PubChem | |
DSSTOX Substance ID |
DTXSID40425011 | |
Record name | C8 DIHYDROCERAMIDE | |
Source | EPA DSSTox | |
URL | https://comptox.epa.gov/dashboard/DTXSID40425011 | |
Description | DSSTox provides a high quality public chemistry resource for supporting improved predictive toxicology. | |
Molecular Weight |
427.7 g/mol | |
Source | PubChem | |
URL | https://pubchem.ncbi.nlm.nih.gov | |
Description | Data deposited in or computed by PubChem | |
CAS No. |
145774-33-0 | |
Record name | C8 DIHYDROCERAMIDE | |
Source | EPA DSSTox | |
URL | https://comptox.epa.gov/dashboard/DTXSID40425011 | |
Description | DSSTox provides a high quality public chemistry resource for supporting improved predictive toxicology. | |
Retrosynthesis Analysis
AI-Powered Synthesis Planning: Our tool employs the Template_relevance Pistachio, Template_relevance Bkms_metabolic, Template_relevance Pistachio_ringbreaker, Template_relevance Reaxys, Template_relevance Reaxys_biocatalysis model, leveraging a vast database of chemical reactions to predict feasible synthetic routes.
One-Step Synthesis Focus: Specifically designed for one-step synthesis, it provides concise and direct routes for your target compounds, streamlining the synthesis process.
Accurate Predictions: Utilizing the extensive PISTACHIO, BKMS_METABOLIC, PISTACHIO_RINGBREAKER, REAXYS, REAXYS_BIOCATALYSIS database, our tool offers high-accuracy predictions, reflecting the latest in chemical research and data.
Strategy Settings
Precursor scoring | Relevance Heuristic |
---|---|
Min. plausibility | 0.01 |
Model | Template_relevance |
Template Set | Pistachio/Bkms_metabolic/Pistachio_ringbreaker/Reaxys/Reaxys_biocatalysis |
Top-N result to add to graph | 6 |
Feasible Synthetic Routes
Q1: How does N-Octanoylsphinganine interact with dihydroceramide desaturase, and what are the downstream effects of this interaction?
A1: N-Octanoylsphinganine serves as a substrate for dihydroceramide desaturase (Des2), a key enzyme responsible for converting dihydroceramides to ceramides []. This enzymatic step is crucial in the de novo synthesis of ceramides, bioactive sphingolipids involved in various cellular processes like apoptosis, inflammation, and cell signaling. Inhibiting Des2 with compounds like GT11 analogs can disrupt ceramide levels and influence these cellular functions [].
Q2: What is the role of N-Octanoylsphinganine in understanding the mechanism of dihydroceramide:sphinganine C-4-hydroxylation?
A2: Research has shown that N-Octanoylsphinganine is a suitable substrate for studying the C-4-hydroxylation activity of Des2 []. By utilizing purified Des2 and N-Octanoylsphinganine in an in vitro assay, scientists were able to determine the enzyme's kinetic parameters (Km and Vmax) and demonstrate the requirement for cytochrome b5 in the hydroxylation process []. This highlights the compound's utility in dissecting the molecular mechanisms of ceramide synthesis.
Q3: Has N-Octanoylsphinganine been investigated as a potential biomarker for any health conditions?
A5: Although not N-Octanoylsphinganine itself, a related compound, Cer 40:0; O2, has been identified as a potential serum biomarker for premature rupture of fetal membranes (PROM) in the first trimester of pregnancy []. This points towards the potential of analyzing specific sphingolipid profiles, which may include N-Octanoylsphinganine and its metabolites, as a strategy for understanding and diagnosing pregnancy-related complications.
Q4: Can dietary components like γ-tocotrienol influence cellular processes by modulating sphingolipids, including dihydroceramides like N-Octanoylsphinganine?
A6: Yes, research suggests that γ-tocotrienol (γTE), a natural form of vitamin E, can influence the cellular inflammatory response by modulating sphingolipid metabolism, including increasing intracellular dihydroceramides [, ]. γTE was shown to upregulate A20, an inhibitor of NF-κB, a key regulator of inflammation. The study suggests that this upregulation is linked to γTE's ability to enhance dihydroceramide levels, potentially impacting cellular processes regulated by this class of sphingolipids [, ].
Descargo de responsabilidad e información sobre productos de investigación in vitro
Tenga en cuenta que todos los artículos e información de productos presentados en BenchChem están destinados únicamente con fines informativos. Los productos disponibles para la compra en BenchChem están diseñados específicamente para estudios in vitro, que se realizan fuera de organismos vivos. Los estudios in vitro, derivados del término latino "in vidrio", involucran experimentos realizados en entornos de laboratorio controlados utilizando células o tejidos. Es importante tener en cuenta que estos productos no se clasifican como medicamentos y no han recibido la aprobación de la FDA para la prevención, tratamiento o cura de ninguna condición médica, dolencia o enfermedad. Debemos enfatizar que cualquier forma de introducción corporal de estos productos en humanos o animales está estrictamente prohibida por ley. Es esencial adherirse a estas pautas para garantizar el cumplimiento de los estándares legales y éticos en la investigación y experimentación.