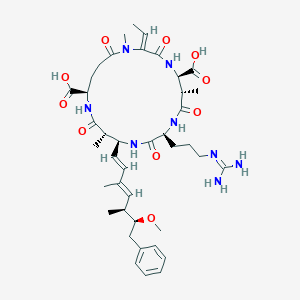
Nodularina
Descripción general
Descripción
La nodularina es una potente hepatotoxina producida por la cianobacteria Nodularia spumigena . Este compuesto es un pentapéptido cíclico, conocido por su estabilidad y resistencia a la degradación por la luz, la temperatura y las microondas . La this compound se encuentra principalmente en cuerpos de agua salobres y es conocida por causar graves daños hepáticos en humanos y animales .
Mecanismo De Acción
La nodularina se dirige principalmente al hígado, donde causa daño citoesquelético, necrosis y rápida formación de ampollas en los hepatocitos . El compuesto inhibe las proteínas fosfatasas 1 y 2A de serina/treonina (PP1 y PP2A), lo que lleva a un aumento de la fosforilación de proteínas y a la interrupción de las funciones celulares . Esta inhibición provoca la muerte celular y el daño a los vasos sanguíneos más finos del hígado .
Aplicaciones Científicas De Investigación
Nodularin has several scientific research applications, particularly in the fields of chemistry, biology, and medicine . Some of the key applications include:
Chemistry: Nodularin is used as a model compound to study the synthesis and stability of cyclic peptides.
Biology: It serves as a tool to investigate the effects of cyanobacterial toxins on aquatic ecosystems and their impact on water quality.
Medicine: Nodularin is studied for its hepatotoxic effects, providing insights into liver diseases and potential therapeutic interventions.
Análisis Bioquímico
Biochemical Properties
Nodularin-R interacts with various enzymes and proteins in biochemical reactions. It is a potent inhibitor of protein serine/threonine phosphatase 1 and 2A (PP1 and PP2A) with IC50 values of 1.8 nM and 0.026 nM, respectively . The biochemical reactions of Nodularin-R are similar to those of microcystin-LR due to their structural similarity, inhibiting specific okadaic acid binding, PP1 and PP2A, and increasing protein phosphorylation in the cells by protein kinases .
Cellular Effects
Nodularin-R has significant effects on various types of cells and cellular processes. It primarily targets the liver, though it also accumulates in the blood, intestines, and kidneys . In the liver, Nodularin-R leads to cytoskeletal damage, necrosis, and rapid blistering of the hepatocytes . Cell death and rapid blistering also destroy the finer blood vessels of the liver .
Molecular Mechanism
Nodularin-R exerts its effects at the molecular level through several mechanisms. It binds to biomolecules, inhibits enzymes, and changes gene expression. During digestion, Nodularin-R diffuses from the small intestine into the liver due to active uptake by an unspecific organic anion transporter in the bile acid carrier transport system .
Temporal Effects in Laboratory Settings
Nodularin-R is a relatively stable compound: light, temperature, and microwaves do little to degrade it . It breaks down slowly at temperatures greater than 104F, pH less than 1, and pH greater than 9 .
Dosage Effects in Animal Models
In animal models, the effects of Nodularin-R vary with different dosages. Fish exposed to Nodularin-R were negatively impacted in every studied ontogenic stage . These cyanotoxins can accumulate in various organs of fish, leading to deleterious effects on the physiology .
Metabolic Pathways
Nodularin-R is involved in several metabolic pathways. The number of metabolic pathways predicted revealed a vast and distinctive yield of potential natural products in the genomes of the genus Nodularia .
Transport and Distribution
Nodularin-R is transported and distributed within cells and tissues. During digestion, Nodularin-R diffuses from the small intestine into the liver due to active uptake by an unspecific organic anion transporter in the bile acid carrier transport system .
Métodos De Preparación
Rutas sintéticas y condiciones de reacción: La nodularina se sintetiza de forma no ribosomal por la cianobacteria Nodularia spumigena . La biosíntesis implica un complejo ensamblaje de aminoácidos no proteicos, incluyendo el ácido N-metil-didehidroaminobutírico y el β-aminoácido ADDA . La ruta sintética es intrincada, involucrando múltiples pasos enzimáticos que aseguran la formación de la estructura cíclica.
Métodos de producción industrial: La producción industrial de this compound no es común debido a su naturaleza tóxica. Para fines de investigación, la this compound puede aislarse de floraciones de cianobacterias. El proceso de aislamiento implica la recolección de las cianobacterias, seguida de la extracción y purificación mediante técnicas como la cromatografía líquida de alto rendimiento (HPLC) y la espectrometría de masas .
Análisis De Reacciones Químicas
Tipos de reacciones: La nodularina experimenta diversas reacciones químicas, incluyendo oxidación, reducción y sustitución. Estas reacciones son cruciales para comprender su estabilidad e interacciones con otros compuestos.
Reactivos y condiciones comunes:
Oxidación: La this compound puede oxidarse utilizando reactivos como el peróxido de hidrógeno en condiciones controladas.
Reducción: Las reacciones de reducción pueden llevarse a cabo utilizando agentes reductores como el borohidruro de sodio.
Sustitución: Las reacciones de sustitución a menudo implican reactivos nucleofílicos que se dirigen a grupos funcionales específicos dentro de la molécula de this compound.
Productos principales formados: Los productos principales formados a partir de estas reacciones dependen de las condiciones específicas y los reactivos utilizados. Por ejemplo, la oxidación puede conducir a la formación de derivados hidroxilados, mientras que la reducción puede resultar en la formación de análogos de this compound reducidos.
4. Aplicaciones de la investigación científica
La this compound tiene varias aplicaciones de investigación científica, particularmente en los campos de la química, la biología y la medicina . Algunas de las aplicaciones clave incluyen:
Química: La this compound se utiliza como compuesto modelo para estudiar la síntesis y estabilidad de los péptidos cíclicos.
Biología: Sirve como herramienta para investigar los efectos de las toxinas de cianobacterias en los ecosistemas acuáticos y su impacto en la calidad del agua.
Medicina: La this compound se estudia por sus efectos hepatoxicos, proporcionando información sobre las enfermedades hepáticas y posibles intervenciones terapéuticas.
Comparación Con Compuestos Similares
La nodularina es estructuralmente similar a las microcistinas, otro grupo de toxinas de cianobacterias . Ambos compuestos son péptidos cíclicos y comparten mecanismos de acción similares, incluida la inhibición de las proteínas fosfatasas . La this compound es un pentapéptido, mientras que las microcistinas son heptapéptidos . Esta diferencia estructural contribuye a variaciones en su toxicidad y estabilidad.
Compuestos similares:
Microcistina-LR: Un heptapéptido con potentes efectos hepatotoxicos.
Ácido okadaico: Una toxina de poliéter que también inhibe las proteínas fosfatasas.
Anatoxina-a: Una neurotoxina producida por cianobacterias, que afecta al sistema nervioso.
La estructura única de la this compound y su potente hepatotoxicidad la convierten en un compuesto significativo para la investigación científica y el monitoreo ambiental.
Propiedades
IUPAC Name |
(2Z,5R,6S,9S,12S,13S,16R)-9-[3-(diaminomethylideneamino)propyl]-2-ethylidene-12-[(1E,3E,5S,6S)-6-methoxy-3,5-dimethyl-7-phenylhepta-1,3-dienyl]-1,6,13-trimethyl-3,7,10,14,19-pentaoxo-1,4,8,11,15-pentazacyclononadecane-5,16-dicarboxylic acid | |
---|---|---|
Source | PubChem | |
URL | https://pubchem.ncbi.nlm.nih.gov | |
Description | Data deposited in or computed by PubChem | |
InChI |
InChI=1S/C41H60N8O10/c1-8-31-38(54)48-34(40(57)58)26(5)36(52)46-29(15-12-20-44-41(42)43)37(53)45-28(25(4)35(51)47-30(39(55)56)18-19-33(50)49(31)6)17-16-23(2)21-24(3)32(59-7)22-27-13-10-9-11-14-27/h8-11,13-14,16-17,21,24-26,28-30,32,34H,12,15,18-20,22H2,1-7H3,(H,45,53)(H,46,52)(H,47,51)(H,48,54)(H,55,56)(H,57,58)(H4,42,43,44)/b17-16+,23-21+,31-8-/t24-,25-,26-,28-,29-,30+,32-,34+/m0/s1 | |
Source | PubChem | |
URL | https://pubchem.ncbi.nlm.nih.gov | |
Description | Data deposited in or computed by PubChem | |
InChI Key |
IXBQSRWSVIBXNC-HSKGSTCASA-N | |
Source | PubChem | |
URL | https://pubchem.ncbi.nlm.nih.gov | |
Description | Data deposited in or computed by PubChem | |
Canonical SMILES |
CC=C1C(=O)NC(C(C(=O)NC(C(=O)NC(C(C(=O)NC(CCC(=O)N1C)C(=O)O)C)C=CC(=CC(C)C(CC2=CC=CC=C2)OC)C)CCCN=C(N)N)C)C(=O)O | |
Source | PubChem | |
URL | https://pubchem.ncbi.nlm.nih.gov | |
Description | Data deposited in or computed by PubChem | |
Isomeric SMILES |
C/C=C\1/C(=O)N[C@H]([C@@H](C(=O)N[C@H](C(=O)N[C@H]([C@@H](C(=O)N[C@H](CCC(=O)N1C)C(=O)O)C)/C=C/C(=C/[C@H](C)[C@H](CC2=CC=CC=C2)OC)/C)CCCN=C(N)N)C)C(=O)O | |
Source | PubChem | |
URL | https://pubchem.ncbi.nlm.nih.gov | |
Description | Data deposited in or computed by PubChem | |
Molecular Formula |
C41H60N8O10 | |
Source | PubChem | |
URL | https://pubchem.ncbi.nlm.nih.gov | |
Description | Data deposited in or computed by PubChem | |
DSSTOX Substance ID |
DTXSID60880022 | |
Record name | Nodularin | |
Source | EPA DSSTox | |
URL | https://comptox.epa.gov/dashboard/DTXSID60880022 | |
Description | DSSTox provides a high quality public chemistry resource for supporting improved predictive toxicology. | |
Molecular Weight |
825.0 g/mol | |
Source | PubChem | |
URL | https://pubchem.ncbi.nlm.nih.gov | |
Description | Data deposited in or computed by PubChem | |
Solubility |
2 mg/mL in methanol | |
Record name | Nodularin | |
Source | Hazardous Substances Data Bank (HSDB) | |
URL | https://pubchem.ncbi.nlm.nih.gov/source/hsdb/7749 | |
Description | The Hazardous Substances Data Bank (HSDB) is a toxicology database that focuses on the toxicology of potentially hazardous chemicals. It provides information on human exposure, industrial hygiene, emergency handling procedures, environmental fate, regulatory requirements, nanomaterials, and related areas. The information in HSDB has been assessed by a Scientific Review Panel. | |
Mechanism of Action |
Direct addition of nodularin to B6C3F1 mouse splenocyte cultures produced a concentration-dependent inhibition of the lymphoproliferative response to concanavalin A stimulation. Nodularin inhibited PMA plus ionomycin (Io)-induced IL-2 mRNA expression in murine splenocytes and thymocytes as determined by quantitative/competitive RT-PCR. To further characterize the mechanism for the transcriptional regulation of IL-2, the binding activity of transcription factors, NF-AT, AP-1, NF-kappaB, and Oct, was evaluated by electrophoretic mobility shift assays in mouse splenocytes. Nodularin reduced the NF-AT binding activity in PMA/Io-induced splenocytes, but no significant effect was observed on AP-1, NF-kappaB, or Oct binding activity. Nodularin also inhibited IL-4 mRNA expression in PMA/Io-stimulated murine splenocytes. These results suggest that T lymphocyte is a possible cellular target of nodularin, and the inhibitory effect of nodularin on T-cell specific transcription factor NF-AT induces T-cell dysfunction, which leads to a diminution in IL-2 and IL-4 gene transcription., Treatment of three microcystin (MC) isotypes, MC-LR, MC-YR and nodularin, on B6C3F1 mouse splenocytes produced dose-dependent inhibition of in vitro polyclonal antibody response and lymphoproliferation to LPS. ConA-induced lymphoproliferative response was decreased by MC-YR and nodularin, but no significant effect was observed in the MC-LR treatment. Intraperitoneal administration of nodularin into B6C3F1 mice decreased humoral immune responses to sheep red blood cell (sRBC), and the inhibitory effect became severe when hepatic uptake of nodularin was blocked by rifampicin. Each MC 1 uM suppressed phorbol 12-myristate 13-acetate (PMA) plus ionomycin-induced IL-2 mRNA expression in splenocytes and thymocytes, but not in EL-4 mouse thymoma cells. To further characterize the mechanism for the reduced IL-2 mRNA level, IL-2 mRNA stability was measured using RT-PCR. Deprivation of PMA/ionomycin stimuli from activated splenocytes and blockade of new transcription resulted in destabilization of IL-2 mRNA, which was accelerated by MC treatment. These results demonstrated that MC down-regulated lymphocyte functions and the immunosuppression was mediated, at least in part, through decreased IL-2 mRNA stability., Okadaic acid is functionally a potent tumor promoter working through inhibition of protein phosphatases 1 and 2A (PP1 and PP2A), resulting in sustained phosphorylation of proteins in cells. The mechanism of tumor promotion with okadaic acid is thus completely different from that of the classic tumor promoter phorbol ester. Other potent inhibitors of PP1 and PP2A - such as dinophysistoxin-1, calyculins A-H, microcystin-LR and its derivatives, and nodularin - were isolated from marine organisms, and their structural features including the crystal structure of the PP1-inhibitor complex, tumor promoting activities, and biochemical and biological effects, are reviewed. The compounds induced tumor promoting activity in three different organs, including mouse skin, rat glandular stomach and rat liver, initiated with three different carcinogens. The results indicate that inhibition of PP1 and PP2A is a general mechanism of tumor promotion applicable to various organs..., A common mechanism in the toxicity of microcystins and nodularin is the specific inhibition of Ser/Thr protein phosphatases 1 and 2A at picomolar concentrations in the cytoplasm and the nucleus in vitro. This inhibition results in hyperphosphorylation of intracellular proteins, which is shown by the rapid disaggregation of intermediate filaments (cytokeratins) that form the cellular scaffold in human and rodent hepatocytes. Microfilaments become detached from the cytoplasmic membrane, which results in cell cytoskeletal deformation and bleb formation. Cell lysis and apoptosis follow, depending on the dose. Death results from dissolution of the liver structure and intrahepatic pooling of blood, which lead to overall haemorrhagic shock. Doses that are not immediately lethal can result in death from liver failure in large animals and humans several months after the initial exposure to microcystin. | |
Record name | Nodularin | |
Source | Hazardous Substances Data Bank (HSDB) | |
URL | https://pubchem.ncbi.nlm.nih.gov/source/hsdb/7749 | |
Description | The Hazardous Substances Data Bank (HSDB) is a toxicology database that focuses on the toxicology of potentially hazardous chemicals. It provides information on human exposure, industrial hygiene, emergency handling procedures, environmental fate, regulatory requirements, nanomaterials, and related areas. The information in HSDB has been assessed by a Scientific Review Panel. | |
CAS No. |
118399-22-7 | |
Record name | Nodularin | |
Source | ChemIDplus | |
URL | https://pubchem.ncbi.nlm.nih.gov/substance/?source=chemidplus&sourceid=0118399227 | |
Description | ChemIDplus is a free, web search system that provides access to the structure and nomenclature authority files used for the identification of chemical substances cited in National Library of Medicine (NLM) databases, including the TOXNET system. | |
Record name | Nodularin | |
Source | EPA DSSTox | |
URL | https://comptox.epa.gov/dashboard/DTXSID60880022 | |
Description | DSSTox provides a high quality public chemistry resource for supporting improved predictive toxicology. | |
Record name | 118399-22-7 | |
Source | European Chemicals Agency (ECHA) | |
URL | https://echa.europa.eu/information-on-chemicals | |
Description | The European Chemicals Agency (ECHA) is an agency of the European Union which is the driving force among regulatory authorities in implementing the EU's groundbreaking chemicals legislation for the benefit of human health and the environment as well as for innovation and competitiveness. | |
Explanation | Use of the information, documents and data from the ECHA website is subject to the terms and conditions of this Legal Notice, and subject to other binding limitations provided for under applicable law, the information, documents and data made available on the ECHA website may be reproduced, distributed and/or used, totally or in part, for non-commercial purposes provided that ECHA is acknowledged as the source: "Source: European Chemicals Agency, http://echa.europa.eu/". Such acknowledgement must be included in each copy of the material. ECHA permits and encourages organisations and individuals to create links to the ECHA website under the following cumulative conditions: Links can only be made to webpages that provide a link to the Legal Notice page. | |
Record name | NODULARIN | |
Source | FDA Global Substance Registration System (GSRS) | |
URL | https://gsrs.ncats.nih.gov/ginas/app/beta/substances/0979BIK2QU | |
Description | The FDA Global Substance Registration System (GSRS) enables the efficient and accurate exchange of information on what substances are in regulated products. Instead of relying on names, which vary across regulatory domains, countries, and regions, the GSRS knowledge base makes it possible for substances to be defined by standardized, scientific descriptions. | |
Explanation | Unless otherwise noted, the contents of the FDA website (www.fda.gov), both text and graphics, are not copyrighted. They are in the public domain and may be republished, reprinted and otherwise used freely by anyone without the need to obtain permission from FDA. Credit to the U.S. Food and Drug Administration as the source is appreciated but not required. | |
Record name | Nodularin | |
Source | Hazardous Substances Data Bank (HSDB) | |
URL | https://pubchem.ncbi.nlm.nih.gov/source/hsdb/7749 | |
Description | The Hazardous Substances Data Bank (HSDB) is a toxicology database that focuses on the toxicology of potentially hazardous chemicals. It provides information on human exposure, industrial hygiene, emergency handling procedures, environmental fate, regulatory requirements, nanomaterials, and related areas. The information in HSDB has been assessed by a Scientific Review Panel. | |
Retrosynthesis Analysis
AI-Powered Synthesis Planning: Our tool employs the Template_relevance Pistachio, Template_relevance Bkms_metabolic, Template_relevance Pistachio_ringbreaker, Template_relevance Reaxys, Template_relevance Reaxys_biocatalysis model, leveraging a vast database of chemical reactions to predict feasible synthetic routes.
One-Step Synthesis Focus: Specifically designed for one-step synthesis, it provides concise and direct routes for your target compounds, streamlining the synthesis process.
Accurate Predictions: Utilizing the extensive PISTACHIO, BKMS_METABOLIC, PISTACHIO_RINGBREAKER, REAXYS, REAXYS_BIOCATALYSIS database, our tool offers high-accuracy predictions, reflecting the latest in chemical research and data.
Strategy Settings
Precursor scoring | Relevance Heuristic |
---|---|
Min. plausibility | 0.01 |
Model | Template_relevance |
Template Set | Pistachio/Bkms_metabolic/Pistachio_ringbreaker/Reaxys/Reaxys_biocatalysis |
Top-N result to add to graph | 6 |
Feasible Synthetic Routes
Descargo de responsabilidad e información sobre productos de investigación in vitro
Tenga en cuenta que todos los artículos e información de productos presentados en BenchChem están destinados únicamente con fines informativos. Los productos disponibles para la compra en BenchChem están diseñados específicamente para estudios in vitro, que se realizan fuera de organismos vivos. Los estudios in vitro, derivados del término latino "in vidrio", involucran experimentos realizados en entornos de laboratorio controlados utilizando células o tejidos. Es importante tener en cuenta que estos productos no se clasifican como medicamentos y no han recibido la aprobación de la FDA para la prevención, tratamiento o cura de ninguna condición médica, dolencia o enfermedad. Debemos enfatizar que cualquier forma de introducción corporal de estos productos en humanos o animales está estrictamente prohibida por ley. Es esencial adherirse a estas pautas para garantizar el cumplimiento de los estándares legales y éticos en la investigación y experimentación.