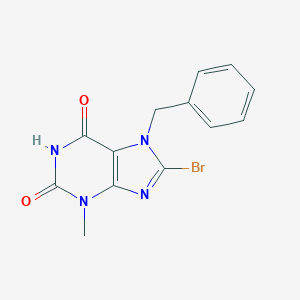
7-Benzyl-8-bromo-3-methylxanthine
Descripción general
Descripción
7-Benzyl-8-bromo-3-methylxanthine is a synthetic xanthine derivative characterized by substitutions at positions 3, 7, and 8 of the xanthine core. The 3-methyl group enhances metabolic stability, while the 7-benzyl and 8-bromo substituents modulate electronic and steric properties, influencing reactivity and biological interactions.
Métodos De Preparación
Bromination of 3-Methylxanthine to 8-Bromo-3-Methylxanthine
The synthesis of 7-benzyl-8-bromo-3-methylxanthine begins with the regioselective bromination of 3-methylxanthine at the 8-position. This step is critical for directing subsequent substitutions and ensuring structural fidelity. Two primary methodologies dominate this stage:
Classical Bromination Using Elemental Bromine
In a traditional approach, 3-methylxanthine is dissolved in glacial acetic acid and treated with bromine (Br) at 60–70°C for 4–6 hours . The reaction proceeds via electrophilic aromatic substitution, with the electron-rich 8-position of the xanthine core preferentially targeted. After quenching with ice water, the precipitate is filtered and recrystallized from ethanol to yield 8-bromo-3-methylxanthine as a light yellow solid (melting point: ~300°C, yield: 80–85%) .
Modern Bromination with N-Bromosuccinimide (NBS)
To enhance safety and selectivity, NBS in chloroform or dichloromethane has emerged as a preferred brominating agent . Under reflux conditions (40–50°C, 8–12 hours), this method achieves comparable yields (85–90%) while minimizing side reactions such as dibromination. The reaction mechanism involves radical intermediates, ensuring precise monobromination .
Table 1: Comparative Bromination Methods
Brominating Agent | Solvent | Temperature (°C) | Time (h) | Yield (%) |
---|---|---|---|---|
Br | Acetic acid | 60–70 | 4–6 | 80–85 |
NBS | Chloroform | 40–50 | 8–12 | 85–90 |
The introduction of the benzyl group at the 7-position of 8-bromo-3-methylxanthine is achieved through nucleophilic alkylation. This step exploits the reactivity of the deprotonated N7 nitrogen, which attacks the electrophilic benzyl chloride in the presence of a base.
Alkylation with Benzyl Chloride
A mixture of 8-bromo-3-methylxanthine, benzyl chloride, and potassium carbonate in DMAc is heated to 75–85°C for 6–8 hours . The polar aprotic solvent facilitates deprotonation, while the elevated temperature accelerates the SN mechanism. Post-reaction, the mixture is diluted with water, and the product is isolated via suction filtration. Recrystallization from ethanol/methyl tert-butyl ether (1:10 v/v) yields this compound as a white crystalline solid (melting point: 215–218°C, yield: 78–82%) .
Solvent and Base Optimization
Alternative solvents such as N,N-dimethylformamide (DMF) and N-methylpyrrolidinone (NMP) have been explored, but DMAc consistently delivers higher yields due to its superior solvation of intermediates . Similarly, substituting potassium carbonate with sodium bicarbonate or sodium carbonate reduces reactivity, underscoring the necessity of strong bases for efficient deprotonation .
Table 2: Benzylation Reaction Parameters
Base | Solvent | Temperature (°C) | Time (h) | Yield (%) |
---|---|---|---|---|
KCO | DMAc | 75–85 | 6–8 | 78–82 |
NaCO | DMF | 75–85 | 6–8 | 65–70 |
NaHCO | NMP | 75–85 | 6–8 | 55–60 |
Analytical Characterization and Quality Control
Nuclear Magnetic Resonance (NMR) Spectroscopy
-NMR analysis (DMSO-d, 400 MHz) confirms the structure of this compound:
-
δ 7.35–7.28 (m, 5H, benzyl aromatic protons)
-
δ 5.42 (s, 2H, N7–CH–Ph)
-
δ 3.45 (s, 3H, N3–CH)
High-Performance Liquid Chromatography (HPLC)
HPLC purity assessments using a C18 column (acetonitrile/water gradient) reveal a principal peak at 12.3 minutes with 99.2–99.5% purity, confirming the absence of unreacted starting materials or byproducts .
Industrial Applications and Derivative Synthesis
This compound serves as a pivotal intermediate in the synthesis of linagliptin, a dipeptidyl peptidase-4 (DPP-4) inhibitor . Subsequent reactions with (R)-3-Boc-aminopiperidine under Mitsunobu conditions yield advanced intermediates, which are deprotected to form the final active pharmaceutical ingredient (API) .
Análisis De Reacciones Químicas
Types of Reactions: 7-Benzyl-8-bromo-3-methylxanthine can undergo various chemical reactions, including:
Substitution Reactions: The bromine atom can be substituted with other nucleophiles under appropriate conditions.
Oxidation and Reduction: The xanthine core can be oxidized or reduced, although these reactions are less common for this specific derivative.
Common Reagents and Conditions:
Substitution: Common reagents include nucleophiles like amines or thiols, often in the presence of a catalyst or under basic conditions.
Oxidation: Strong oxidizing agents like potassium permanganate can be used, although care must be taken to avoid over-oxidation.
Reduction: Reducing agents like lithium aluminum hydride can be employed, but these reactions are typically more challenging due to the stability of the xanthine core.
Major Products:
Substitution Products: Depending on the nucleophile used, various substituted derivatives can be formed.
Oxidation and Reduction Products: These reactions can lead to changes in the oxidation state of the xanthine core, potentially forming new derivatives with different biological activities.
Aplicaciones Científicas De Investigación
Pharmacological Research
1.1 Adenosine Receptor Modulation
7-Benzyl-8-bromo-3-methylxanthine has been studied for its ability to modulate adenosine receptors, which play a crucial role in various physiological processes. Research indicates that this compound acts as an antagonist at the A1 and A2A adenosine receptor subtypes, making it a valuable tool in studying the effects of adenosine signaling in different biological contexts .
1.2 Development of DPP-4 Inhibitors
This compound is also investigated as a synthetic intermediate for developing Dipeptidyl Peptidase-4 (DPP-4) inhibitors, which are significant in treating type 2 diabetes. DPP-4 inhibitors enhance incretin levels, leading to improved insulin secretion and reduced blood glucose levels . The synthesis of derivatives based on this compound has shown promising results in enhancing the potency and selectivity of these inhibitors.
Cancer Research
2.1 Tyrosine Kinase Inhibition
Research has highlighted the potential of this compound as a tyrosine kinase inhibitor. Tyrosine kinases are critical in cancer cell proliferation and survival. Studies demonstrate that derivatives of this compound can selectively inhibit specific tyrosine kinases, thereby inducing apoptosis in cancer cells . This application opens avenues for developing targeted cancer therapies.
Neuropharmacology
3.1 Treatment of Neurodegenerative Diseases
The compound's role in neuropharmacology is under investigation, particularly concerning its ability to cross the blood-brain barrier and influence neuroprotective pathways. Its derivatives have been linked to potential treatments for neurodegenerative diseases by modulating neurotransmitter systems and reducing oxidative stress .
Synthesis and Derivatives
The synthesis of this compound involves several chemical reactions that yield various derivatives with enhanced biological activities. For instance, modifications at different positions on the xanthine scaffold can lead to compounds with improved receptor binding affinities or altered pharmacokinetic profiles .
Case Studies and Research Findings
Several studies have documented the efficacy of this compound and its derivatives:
Mecanismo De Acción
The mechanism of action of 7-Benzyl-8-bromo-3-methylxanthine involves its interaction with specific molecular targets, such as enzymes and receptors. The compound can act as an inhibitor or modulator of these targets, affecting various biochemical pathways. For example, xanthine derivatives are known to inhibit phosphodiesterase enzymes, leading to increased levels of cyclic AMP and subsequent physiological effects.
Comparación Con Compuestos Similares
Comparison with Structurally Related Compounds
Key Observations :
- Synthetic Accessibility: 7-Benzyl derivatives are synthesized efficiently in aqueous dioxane with hydrazine hydrate, yielding ~70–80% purity . In contrast, bulkier 7-phenoxypropyl derivatives require longer reaction times and specialized conditions due to steric hindrance .
- Isomerism : 8-thioacetic acid derivatives (e.g., 7-ethyl-8-thioacetic acid) exhibit E/Z isomerism (2:1 ratio), confirmed via NMR spectroscopy, unlike brominated analogs .
Physicochemical and Spectral Properties
Key Observations :
- Solubility : Brominated derivatives (e.g., 7-benzyl-8-bromo) exhibit low water solubility due to hydrophobic substituents, whereas thioacetic acid analogs show improved solubility .
- Thermal Stability : Higher melting points in brominated compounds (e.g., 215°C for 7-benzyl-8-bromo) suggest greater crystalline stability compared to thio derivatives (~175°C) .
Key Observations :
- Antimicrobial Potency : Thioacetic acid derivatives (e.g., 7-ethyl-8-thioacetic acid) demonstrate superior activity (MIC 10–25 µg/mL) compared to brominated analogs .
- Structure-Activity Relationship (SAR): Bulkier 7-substituents (e.g., phenoxypropyl) may reduce bioavailability despite favorable spectral properties .
Actividad Biológica
7-Benzyl-8-bromo-3-methylxanthine (7-BBrMX) is a xanthine derivative that has garnered attention for its potential therapeutic applications, particularly due to its interaction with phosphodiesterase 9A (PDE9A). This compound plays a significant role in modulating cellular signaling pathways, particularly those involving cyclic guanosine monophosphate (cGMP).
Target Enzyme: Phosphodiesterase 9A (PDE9A)
7-BBrMX primarily targets PDE9A, an enzyme responsible for the degradation of cGMP. By inhibiting this enzyme, 7-BBrMX prevents the breakdown of cGMP, leading to increased intracellular levels of this second messenger. The elevation of cGMP activates protein kinase G (PKG), which in turn phosphorylates various target proteins, influencing multiple cellular processes such as:
- Cell proliferation
- Ion channel conductance
- Smooth muscle contraction and relaxation
This mechanism highlights the compound's potential in therapeutic contexts where modulation of cGMP signaling is beneficial, such as cardiovascular diseases and neurodegenerative disorders.
Biochemical Pathways Affected
The inhibition of PDE9A by 7-BBrMX enhances cGMP-dependent signaling pathways. The resultant increase in cGMP levels can lead to:
- Vasodilation : Enhanced relaxation of vascular smooth muscle.
- Neuroprotection : Potential protective effects against neuronal damage through modulation of signaling pathways involved in apoptosis.
- Cognitive Enhancement : Possible implications for improving cognitive function through modulation of neurotransmitter systems influenced by cGMP.
Research Findings and Case Studies
Several studies have explored the biological effects and potential therapeutic applications of 7-BBrMX:
- Cardiovascular Research : In a study examining the effects of PDE9A inhibitors on heart function, 7-BBrMX demonstrated significant improvements in cardiac contractility and relaxation, suggesting its potential use in heart failure management.
- Neuropharmacology : Research indicated that 7-BBrMX could enhance memory retention in animal models by modulating cGMP levels within the hippocampus, a brain region critical for learning and memory .
- Anti-inflammatory Effects : Another study found that 7-BBrMX exhibited anti-inflammatory properties by reducing cytokine production in activated macrophages, indicating its potential role in treating inflammatory diseases .
The synthesis of this compound has been well-documented. It involves the reaction of xanthine derivatives with various substituents under controlled conditions to yield compounds with specific biological activities. The physical-chemical properties have been characterized using methods such as NMR spectroscopy and elemental analysis, confirming the compound's structure and purity .
Applications in Medicine and Industry
The unique properties of 7-BBrMX make it a valuable compound not only in research but also in potential clinical applications:
- Drug Development : Its ability to selectively inhibit PDE9A positions it as a candidate for developing drugs targeting cognitive decline and cardiovascular diseases.
- Biochemical Assays : In laboratory settings, it serves as a tool for studying xanthine derivatives' effects on various biological pathways.
Q & A
Basic Research Questions
Q. How can researchers optimize the synthesis of 7-Benzyl-8-bromo-3-methylxanthine to improve yield and purity?
Q. What spectroscopic methods are most reliable for confirming the structure of this compound?
Methodological Answer:
- PMR spectroscopy : Key proton signals include:
- Aromatic protons (7.46–7.18 ppm, multiplet) for the benzyl group.
- N3-CH3 singlet at 3.38–3.26 ppm.
- Secondary alcohol protons (5.78–5.45 ppm, doublet) if hydroxyl-containing intermediates are present .
Advanced Research Questions
Q. How can researchers resolve contradictions between solubility data and observed biological activity in this compound derivatives?
Methodological Answer:
- Apply theoretical frameworks to link solubility (e.g., poor aqueous solubility but solubility in DMF/DMSO) to activity. For example:
- Use Hansch analysis to correlate logP values with antimicrobial efficacy .
- Test derivatives with polar substituents (e.g., hydroxyl or amino groups) to improve solubility while retaining activity .
- Address discrepancies by replicating experiments under standardized conditions (e.g., pH 7.4 buffer for solubility assays) and validating via HPLC purity checks .
Q. What strategies are effective for establishing structure-activity relationships (SAR) in brominated xanthines targeting microbial pathogens?
Methodological Answer:
- Systematic substitution : Synthesize analogs with:
- Varied benzyl substituents (e.g., 4-bromo vs. 3-methylbenzyl) to assess halogen and alkyl group impacts .
- Modified bromine positions (e.g., 8-bromo vs. 6-bromo) to study steric and electronic effects .
- Biological testing : Use MIC (Minimum Inhibitory Concentration) assays against Gram-positive bacteria (e.g., S. aureus) and fungi (e.g., C. albicans). Prioritize compounds showing activity at ≤50 µg/mL .
Q. How should researchers design experiments to investigate the mechanism of action of this compound in enzyme inhibition?
Methodological Answer:
- Kinetic assays : Measure inhibition constants (Ki) for xanthine oxidase or phosphodiesterase isoforms using fluorogenic substrates (e.g., Amplex Red for H₂O₂ detection) .
- Molecular docking : Use crystallographic data from related xanthines (e.g., theobromine, PDB ID 1WYG) to model binding interactions with target enzymes .
- Competitive binding studies : Compare IC₅₀ values with known inhibitors (e.g., theophylline) to identify competitive vs. allosteric effects .
Q. What advanced analytical techniques can quantify trace impurities in this compound batches?
Methodological Answer:
- High-resolution LC-MS : Detect impurities at ≤0.1% levels using C18 columns and acetonitrile/water gradients .
- X-ray crystallography : Resolve structural ambiguities (e.g., bromine positioning) in purified crystals .
- Elemental analysis : Validate Br and N content (±0.3% tolerance) to confirm stoichiometry .
Q. Methodological Design & Data Analysis
Q. How can researchers leverage NMR data to study dynamic molecular interactions of this compound in solution?
Methodological Answer:
- Perform NOESY experiments to detect spatial proximity between the benzyl group and the xanthine core, confirming intramolecular stacking .
- Analyze temperature-dependent NMR shifts to assess hydrogen bonding strength (e.g., NH protons at 10.98–10.54 ppm) .
Q. What experimental approaches are recommended for synthesizing and testing novel 8-substituted analogs of this compound?
Methodological Answer:
- Protecting group strategies : Use tert-butyldimethylsilyl (TBDMS) groups to shield reactive sites during bromination or alkylation .
- Parallel synthesis : Employ robotic liquid handlers to generate libraries of 8-amino or 8-thio derivatives for high-throughput screening .
- Data normalization : Use Z-score analysis to rank biological activity across analogs, minimizing batch-to-batch variability .
Propiedades
IUPAC Name |
7-benzyl-8-bromo-3-methylpurine-2,6-dione | |
---|---|---|
Source | PubChem | |
URL | https://pubchem.ncbi.nlm.nih.gov | |
Description | Data deposited in or computed by PubChem | |
InChI |
InChI=1S/C13H11BrN4O2/c1-17-10-9(11(19)16-13(17)20)18(12(14)15-10)7-8-5-3-2-4-6-8/h2-6H,7H2,1H3,(H,16,19,20) | |
Source | PubChem | |
URL | https://pubchem.ncbi.nlm.nih.gov | |
Description | Data deposited in or computed by PubChem | |
InChI Key |
SFIWIEFIJQKWQZ-UHFFFAOYSA-N | |
Source | PubChem | |
URL | https://pubchem.ncbi.nlm.nih.gov | |
Description | Data deposited in or computed by PubChem | |
Canonical SMILES |
CN1C2=C(C(=O)NC1=O)N(C(=N2)Br)CC3=CC=CC=C3 | |
Source | PubChem | |
URL | https://pubchem.ncbi.nlm.nih.gov | |
Description | Data deposited in or computed by PubChem | |
Molecular Formula |
C13H11BrN4O2 | |
Source | PubChem | |
URL | https://pubchem.ncbi.nlm.nih.gov | |
Description | Data deposited in or computed by PubChem | |
DSSTOX Substance ID |
DTXSID70356889 | |
Record name | 7-benzyl-8-bromo-3-methylxanthine | |
Source | EPA DSSTox | |
URL | https://comptox.epa.gov/dashboard/DTXSID70356889 | |
Description | DSSTox provides a high quality public chemistry resource for supporting improved predictive toxicology. | |
Molecular Weight |
335.16 g/mol | |
Source | PubChem | |
URL | https://pubchem.ncbi.nlm.nih.gov | |
Description | Data deposited in or computed by PubChem | |
Solubility |
42.7 [ug/mL] (The mean of the results at pH 7.4) | |
Record name | SID47204368 | |
Source | Burnham Center for Chemical Genomics | |
URL | https://pubchem.ncbi.nlm.nih.gov/bioassay/1996#section=Data-Table | |
Description | Aqueous solubility in buffer at pH 7.4 | |
CAS No. |
93703-26-5 | |
Record name | 8-Bromo-3,7-dihydro-3-methyl-7-(phenylmethyl)-1H-purine-2,6-dione | |
Source | CAS Common Chemistry | |
URL | https://commonchemistry.cas.org/detail?cas_rn=93703-26-5 | |
Description | CAS Common Chemistry is an open community resource for accessing chemical information. Nearly 500,000 chemical substances from CAS REGISTRY cover areas of community interest, including common and frequently regulated chemicals, and those relevant to high school and undergraduate chemistry classes. This chemical information, curated by our expert scientists, is provided in alignment with our mission as a division of the American Chemical Society. | |
Explanation | The data from CAS Common Chemistry is provided under a CC-BY-NC 4.0 license, unless otherwise stated. | |
Record name | 7-benzyl-8-bromo-3-methylxanthine | |
Source | EPA DSSTox | |
URL | https://comptox.epa.gov/dashboard/DTXSID70356889 | |
Description | DSSTox provides a high quality public chemistry resource for supporting improved predictive toxicology. | |
Synthesis routes and methods I
Procedure details
Synthesis routes and methods II
Procedure details
Synthesis routes and methods III
Procedure details
Descargo de responsabilidad e información sobre productos de investigación in vitro
Tenga en cuenta que todos los artículos e información de productos presentados en BenchChem están destinados únicamente con fines informativos. Los productos disponibles para la compra en BenchChem están diseñados específicamente para estudios in vitro, que se realizan fuera de organismos vivos. Los estudios in vitro, derivados del término latino "in vidrio", involucran experimentos realizados en entornos de laboratorio controlados utilizando células o tejidos. Es importante tener en cuenta que estos productos no se clasifican como medicamentos y no han recibido la aprobación de la FDA para la prevención, tratamiento o cura de ninguna condición médica, dolencia o enfermedad. Debemos enfatizar que cualquier forma de introducción corporal de estos productos en humanos o animales está estrictamente prohibida por ley. Es esencial adherirse a estas pautas para garantizar el cumplimiento de los estándares legales y éticos en la investigación y experimentación.