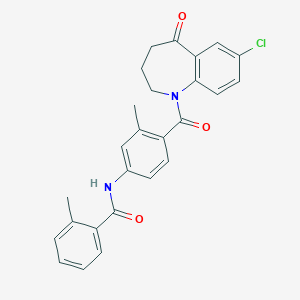
5-Dehidro Tolvaptan
Descripción general
Descripción
Dehydrotolvaptan, also known as Dehydrotolvaptan, is a useful research compound. Its molecular formula is C26H23ClN2O3 and its molecular weight is 446.9 g/mol. The purity is usually 95%.
BenchChem offers high-quality Dehydrotolvaptan suitable for many research applications. Different packaging options are available to accommodate customers' requirements. Please inquire for more information about Dehydrotolvaptan including the price, delivery time, and more detailed information at info@benchchem.com.
Aplicaciones Científicas De Investigación
Tratamiento de la enfermedad renal poliquística autosómica dominante (ADPKD)
5-Dehidro Tolvaptan ha sido identificado como un antagonista selectivo del receptor V2 de la vasopresina, que es el primer y único tratamiento específico aprobado para la ADPKD . Ha demostrado eficacia en la prevención de la progresión de la ADPKD cuando se administra de manera oportuna, particularmente en pacientes con mejor historial renal familiar y menor duración de la hipertensión .
Manejo de la retención de agua en la insuficiencia cardíaca
El compuesto ha sido revisado por su efectividad en el tratamiento de la retención de agua en pacientes con insuficiencia cardíaca. Actúa para aumentar la excreción de agua libre (aquaresis) sin introducir anomalías electrolíticas o empeorar la función renal . Esta aplicación es significativa debido a la alta prevalencia de la insuficiencia cardíaca y las complicaciones asociadas.
Tratamiento de la hiponatremia
This compound se utiliza en el manejo de la hiponatremia, que es una condición caracterizada por bajos niveles de sodio en la sangre. Es particularmente útil en casos de hiponatremia hipervolémica y euvolémica, donde ayuda a corregir los niveles de sodio sin causar cambios rápidos que podrían conducir al síndrome de desmielinización osmótica .
Monitoreo de los efectos adversos aquiréticos
La investigación también se ha centrado en los efectos adversos aquiréticos de Tolvaptan, asegurando que el aumento de la diuresis no afecte la eficacia del tratamiento o cause cambios significativos en la función renal y el equilibrio electrolítico . Esto es crucial para la seguridad a largo plazo de los pacientes que se someten a tratamiento con este compuesto.
Propiedades farmacocinéticas y farmacodinámicas
Se ha realizado una revisión exhaustiva de las propiedades farmacocinéticas y farmacodinámicas de Tolvaptan, incluidas las interacciones medicamentosas. Comprender estas propiedades es esencial para guiar el uso clínico adecuado y garantizar la seguridad del paciente durante el tratamiento .
Formulación intravenosa para congestión avanzada
Se ha desarrollado una formulación intravenosa de Tolvaptan, conocida como Samtas®, para individuos que experimentan congestión avanzada refractaria a los diuréticos convencionales y que tienen dificultades para la ingesta oral . Esto amplía las aplicaciones terapéuticas del compuesto, especialmente en entornos de atención aguda.
Mecanismo De Acción
Target of Action
5-Dehydro Tolvaptan primarily targets the vasopressin V2 receptors . These receptors are found in the walls of the vasculature and luminal membranes of renal collecting ducts . Vasopressin, a hormone that regulates water retention in the body, acts on these V2 receptors .
Mode of Action
5-Dehydro Tolvaptan acts as a selective and competitive antagonist of the vasopressin V2 receptor . By blocking V2 receptors in the renal collecting ducts, it prevents the insertion of aquaporins into the walls, thereby inhibiting water absorption . This action ultimately results in an increase in urine volume and a decrease in urine osmolality .
Biochemical Pathways
The primary biochemical pathway affected by 5-Dehydro Tolvaptan is the vasopressin-regulated water reabsorption pathway . By antagonizing the vasopressin V2 receptor, 5-Dehydro Tolvaptan disrupts the normal function of this pathway, leading to increased excretion of water and electrolytes from the kidneys .
Pharmacokinetics
Studies on tolvaptan, a related compound, suggest that it is well absorbed with a bioavailability of around 40% . It is extensively protein-bound (99%) and is metabolized in the liver via the CYP3A4 pathway . The elimination half-life of Tolvaptan is approximately 12 hours .
Result of Action
The primary result of 5-Dehydro Tolvaptan’s action is an increase in urine volume and a decrease in urine osmolality . This leads to an overall negative fluid balance in patients taking the drug, resulting in increases in serum sodium and osmolality . It is particularly useful for patients with conditions like congestive heart failure, cirrhosis, and the syndrome of inappropriate antidiuretic hormone (SIADH), where higher serum levels of vasopressin are observed .
Action Environment
The action of 5-Dehydro Tolvaptan can be influenced by various environmental factors. For instance, co-administration with CYP3A4 inhibitors or inducers should be avoided due to potential drug interactions . Furthermore, the compound should be handled in a well-ventilated place, and contact with skin and eyes should be avoided . It should be stored in a sealed container in a dry room .
Análisis Bioquímico
Biochemical Properties
5-Dehydro Tolvaptan is metabolized to at least 20 phase I metabolites, the biotransformation reactions being catalyzed mainly by CYP3A4 and CYP3A5 isoforms . The phase-I reactions include hydroxylation (in different positions), carboxylation, oxidation, hydrogenation, dealkylation, isomerization, and a combination of the above .
Cellular Effects
5-Dehydro Tolvaptan, like Tolvaptan, is expected to have effects on various types of cells and cellular processes. Tolvaptan has been shown to reduce cAMP levels, cellular proliferation, and fluid secretion of cystic epithelia in animal models
Molecular Mechanism
The molecular mechanism of 5-Dehydro Tolvaptan is likely similar to that of Tolvaptan, given that it is an impurity of Tolvaptan. Tolvaptan is a selective and competitive arginine vasopressin receptor 2 antagonist . It interferes with cAMP accumulation, slowing total kidney volume .
Dosage Effects in Animal Models
Tolvaptan has been shown to slow kidney function decline in high-risk patients with autosomal dominant polycystic kidney disease (ADPKD) .
Metabolic Pathways
5-Dehydro Tolvaptan is involved in metabolic pathways catalyzed mainly by CYP3A4 and CYP3A5 isoforms . Most of the phase I metabolites undergo glucuronidation
Propiedades
IUPAC Name |
N-[4-(7-chloro-5-oxo-3,4-dihydro-2H-1-benzazepine-1-carbonyl)-3-methylphenyl]-2-methylbenzamide | |
---|---|---|
Source | PubChem | |
URL | https://pubchem.ncbi.nlm.nih.gov | |
Description | Data deposited in or computed by PubChem | |
InChI |
InChI=1S/C26H23ClN2O3/c1-16-6-3-4-7-20(16)25(31)28-19-10-11-21(17(2)14-19)26(32)29-13-5-8-24(30)22-15-18(27)9-12-23(22)29/h3-4,6-7,9-12,14-15H,5,8,13H2,1-2H3,(H,28,31) | |
Source | PubChem | |
URL | https://pubchem.ncbi.nlm.nih.gov | |
Description | Data deposited in or computed by PubChem | |
InChI Key |
VENGMROMZOKURN-UHFFFAOYSA-N | |
Source | PubChem | |
URL | https://pubchem.ncbi.nlm.nih.gov | |
Description | Data deposited in or computed by PubChem | |
Canonical SMILES |
CC1=CC=CC=C1C(=O)NC2=CC(=C(C=C2)C(=O)N3CCCC(=O)C4=C3C=CC(=C4)Cl)C | |
Source | PubChem | |
URL | https://pubchem.ncbi.nlm.nih.gov | |
Description | Data deposited in or computed by PubChem | |
Molecular Formula |
C26H23ClN2O3 | |
Source | PubChem | |
URL | https://pubchem.ncbi.nlm.nih.gov | |
Description | Data deposited in or computed by PubChem | |
DSSTOX Substance ID |
DTXSID60463591 | |
Record name | N-[4-(7-Chloro-5-oxo-2,3,4,5-tetrahydro-1H-1-benzazepine-1-carbonyl)-3-methylphenyl]-2-methylbenzamide | |
Source | EPA DSSTox | |
URL | https://comptox.epa.gov/dashboard/DTXSID60463591 | |
Description | DSSTox provides a high quality public chemistry resource for supporting improved predictive toxicology. | |
Molecular Weight |
446.9 g/mol | |
Source | PubChem | |
URL | https://pubchem.ncbi.nlm.nih.gov | |
Description | Data deposited in or computed by PubChem | |
CAS No. |
137973-76-3 | |
Record name | N-(4-((7-Chloro-2,3,4,5-tetrahydro-5-oxo-1H-1-benzazepin-1-yl)carbonyl)-3-methylphenyl)-2-methylbenzamide | |
Source | ChemIDplus | |
URL | https://pubchem.ncbi.nlm.nih.gov/substance/?source=chemidplus&sourceid=0137973763 | |
Description | ChemIDplus is a free, web search system that provides access to the structure and nomenclature authority files used for the identification of chemical substances cited in National Library of Medicine (NLM) databases, including the TOXNET system. | |
Record name | N-[4-(7-Chloro-5-oxo-2,3,4,5-tetrahydro-1H-1-benzazepine-1-carbonyl)-3-methylphenyl]-2-methylbenzamide | |
Source | EPA DSSTox | |
URL | https://comptox.epa.gov/dashboard/DTXSID60463591 | |
Description | DSSTox provides a high quality public chemistry resource for supporting improved predictive toxicology. | |
Record name | N-(4-((7-CHLORO-2,3,4,5-TETRAHYDRO-5-OXO-1H-1-BENZAZEPIN-1-YL)CARBONYL)-3-METHYLPHENYL)-2-METHYLBENZAMIDE | |
Source | FDA Global Substance Registration System (GSRS) | |
URL | https://gsrs.ncats.nih.gov/ginas/app/beta/substances/YR22W5RA83 | |
Description | The FDA Global Substance Registration System (GSRS) enables the efficient and accurate exchange of information on what substances are in regulated products. Instead of relying on names, which vary across regulatory domains, countries, and regions, the GSRS knowledge base makes it possible for substances to be defined by standardized, scientific descriptions. | |
Explanation | Unless otherwise noted, the contents of the FDA website (www.fda.gov), both text and graphics, are not copyrighted. They are in the public domain and may be republished, reprinted and otherwise used freely by anyone without the need to obtain permission from FDA. Credit to the U.S. Food and Drug Administration as the source is appreciated but not required. | |
Synthesis routes and methods I
Procedure details
Synthesis routes and methods II
Procedure details
Retrosynthesis Analysis
AI-Powered Synthesis Planning: Our tool employs the Template_relevance Pistachio, Template_relevance Bkms_metabolic, Template_relevance Pistachio_ringbreaker, Template_relevance Reaxys, Template_relevance Reaxys_biocatalysis model, leveraging a vast database of chemical reactions to predict feasible synthetic routes.
One-Step Synthesis Focus: Specifically designed for one-step synthesis, it provides concise and direct routes for your target compounds, streamlining the synthesis process.
Accurate Predictions: Utilizing the extensive PISTACHIO, BKMS_METABOLIC, PISTACHIO_RINGBREAKER, REAXYS, REAXYS_BIOCATALYSIS database, our tool offers high-accuracy predictions, reflecting the latest in chemical research and data.
Strategy Settings
Precursor scoring | Relevance Heuristic |
---|---|
Min. plausibility | 0.01 |
Model | Template_relevance |
Template Set | Pistachio/Bkms_metabolic/Pistachio_ringbreaker/Reaxys/Reaxys_biocatalysis |
Top-N result to add to graph | 6 |
Feasible Synthetic Routes
Descargo de responsabilidad e información sobre productos de investigación in vitro
Tenga en cuenta que todos los artículos e información de productos presentados en BenchChem están destinados únicamente con fines informativos. Los productos disponibles para la compra en BenchChem están diseñados específicamente para estudios in vitro, que se realizan fuera de organismos vivos. Los estudios in vitro, derivados del término latino "in vidrio", involucran experimentos realizados en entornos de laboratorio controlados utilizando células o tejidos. Es importante tener en cuenta que estos productos no se clasifican como medicamentos y no han recibido la aprobación de la FDA para la prevención, tratamiento o cura de ninguna condición médica, dolencia o enfermedad. Debemos enfatizar que cualquier forma de introducción corporal de estos productos en humanos o animales está estrictamente prohibida por ley. Es esencial adherirse a estas pautas para garantizar el cumplimiento de los estándares legales y éticos en la investigación y experimentación.