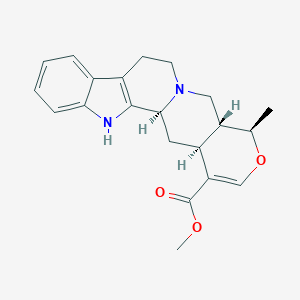
Mayumbine
Descripción general
Descripción
Mayumbine (19-epi-ajmalicine; CAS: 25532-45-0) is a heteroyohimbine monoterpene indole alkaloid (MIA) belonging to the Corynanthe structural subclass. It is biosynthesized in plants such as Rauvolfia tetraphylla and Catharanthus roseus . Structurally, it is characterized by a stereochemical inversion at the C19 position compared to ajmalicine, making it a C19 epimer . This subtle structural difference significantly impacts its biological activity and receptor specificity.
Mecanismo De Acción
Target of Action
19-epi-Ajmalicine, also known as Mayumbine, is a heteroyohimbine-type alkaloid . It is structurally related to yohimbine, rauwolscine, and other yohimban derivatives . Like corynanthine, it acts as a α1-adrenergic receptor antagonist with preferential actions over α2-adrenergic receptors . This suggests that the primary targets of 19-epi-Ajmalicine are α1 and α2-adrenergic receptors.
Mode of Action
The mode of action of 19-epi-Ajmalicine involves its interaction with α1 and α2-adrenergic receptors. As an antagonist, it binds to these receptors and inhibits their activity . This interaction changes the shape and threshold of cardiac action potentials , which can lead to alterations in heart rhythm.
Biochemical Pathways
The biosynthesis of 19-epi-Ajmalicine involves a complex pathway that includes several biochemical reactions and key enzymes . The pathway starts with the formation of strictosidine aglycone, a common biosynthetic intermediate for many indole alkaloids . An enzyme called tetrahydroalstonine synthase (THAS) then uses cathenamine as a substrate and NADPH as a cofactor to form 19-epi-Ajmalicine .
Pharmacokinetics (ADME Properties)
It is known that ajmalicine, a closely related compound, has potent sodium channel blocking effects and a very short half-life , which suggests that 19-epi-Ajmalicine may have similar properties
Result of Action
The molecular and cellular effects of 19-epi-Ajmalicine’s action are primarily related to its antagonistic activity on α1 and α2-adrenergic receptors. By inhibiting these receptors, 19-epi-Ajmalicine can alter cardiac action potentials and potentially improve abnormal heart rhythms .
Action Environment
The action, efficacy, and stability of 19-epi-Ajmalicine can be influenced by various environmental factors. For instance, the production of 19-epi-Ajmalicine in plants can be affected by factors such as soil quality, temperature, and light conditions . In the human body, factors such as pH, temperature, and the presence of other substances can influence the action and efficacy of 19-epi-Ajmalicine.
Análisis Bioquímico
Biochemical Properties
19-epi-Ajmalicine interacts with various enzymes and proteins in biochemical reactions. It can be enzymatically obtained along with its isomers . The enzymes involved in its biosynthesis include G10H, 10HGO, TDC, SLS, STR, and SDG . These enzymes play a crucial role in the terpenoid indole alkaloid (TIA) pathway, which is essential for the production of 19-epi-Ajmalicine .
Cellular Effects
The effects of 19-epi-Ajmalicine on various types of cells and cellular processes are significant. It influences cell function, including impacts on cell signaling pathways, gene expression, and cellular metabolism
Molecular Mechanism
The molecular mechanism of 19-epi-Ajmalicine involves its interactions at the molecular level. It exerts its effects through binding interactions with biomolecules, enzyme inhibition or activation, and changes in gene expression . The STR gene encoding strictosidine synthase of the TIA pathway could be the regulatory gene of the 19-epi-Ajmalicine biosynthesis .
Dosage Effects in Animal Models
The effects of 19-epi-Ajmalicine vary with different dosages in animal models. This includes any threshold effects observed in these studies, as well as any toxic or adverse effects at high doses
Metabolic Pathways
19-epi-Ajmalicine is involved in several metabolic pathways. It interacts with enzymes or cofactors in these pathways, which could also include any effects on metabolic flux or metabolite levels . The metabolic regulation of 19-epi-Ajmalicine is significant, and fluxomic analysis helps in understanding the flux of the alkaloid through the complicated metabolic pathway .
Subcellular Localization
The subcellular localization of 19-epi-Ajmalicine and any effects on its activity or function are crucial aspects of its biochemistry. This could include any targeting signals or post-translational modifications that direct it to specific compartments or organelles
Actividad Biológica
Mayumbine, also known as 19-epi-ajmalicine, is a significant alkaloid derived from the plant Catharanthus roseus. This compound is part of the heteroyohimbine class of alkaloids, which exhibit diverse biological activities due to their unique chemical structures and stereochemistry. The following sections provide a comprehensive overview of the biological activity, biosynthesis, and pharmacological properties of this compound, supported by research findings and relevant case studies.
Chemical Structure and Biosynthesis
This compound is synthesized through complex biosynthetic pathways involving several enzymes. The primary precursor for this compound is strictosidine, which undergoes deglucosylation to form strictosidine aglycone. This aglycone can rearrange and be reduced by various enzymes to produce different alkaloids, including this compound. Key enzymes involved in this process include:
- Strictosidine β-D-glucosidase (SGD) : Catalyzes the conversion of strictosidine to strictosidine aglycone.
- Medium Chain Dehydrogenase/Reductase (MDR) : Responsible for the stereochemical variations that lead to the formation of this compound and other heteroyohimbines.
Recent studies have identified multiple heteroyohimbine synthases (HYSs) in C. roseus, which contribute to the production of this compound alongside ajmalicine and tetrahydroalstonine. For instance, one enzyme was found to produce a mixture of ajmalicine, tetrahydroalstonine, and this compound in a ratio of 55:27:15 at pH 6 .
Pharmacological Activities
This compound has been shown to interact with various biological targets, leading to significant pharmacological effects:
- Benzodiazepine Receptor Ligand : this compound acts as a ligand for benzodiazepine receptors, which are crucial for mediating anxiolytic effects. This interaction suggests potential applications in treating anxiety disorders .
- Antihypertensive Effects : Similar to its analog ajmalicine, this compound exhibits antihypertensive properties by acting on α1-adrenergic receptors .
- Neuropharmacological Effects : The compound's interaction with neurotransmitter systems indicates possible roles in managing conditions like depression and psychosis .
Case Studies and Research Findings
Several studies have investigated the biological activity of this compound:
- Enzymatic Activity Studies : Research demonstrated that specific MDRs from C. roseus could effectively convert strictosidine aglycone into this compound. The yield and product specificity varied significantly depending on enzyme conditions, highlighting the complexity of its biosynthesis .
- Pharmacological Testing : In vitro assays have shown that this compound possesses notable activity against certain cancer cell lines, suggesting its potential as an anticancer agent. For example, it has been evaluated alongside other monoterpene indole alkaloids for acetylcholinesterase inhibitory activity, revealing promising results in neuroprotection .
- Structural Investigations : Advanced techniques such as liquid chromatography-mass spectrometry (LC-MS) have been employed to analyze the products formed during enzymatic reactions involving this compound synthesis. These studies provide insights into the stereochemical diversity and potential therapeutic applications of this compound .
Summary Table of Biological Activities
Aplicaciones Científicas De Investigación
Chemical Properties and Mechanism of Action
Mayumbine is structurally related to yohimbine, sharing a complex molecular framework characterized by multiple rings and functional groups. Its binding affinity to benzodiazepine receptors suggests potential anxiolytic effects similar to those observed with diazepam . However, comprehensive studies on its exact mechanism of action are still required.
Pharmacological Applications
- Antihypertensive Effects
-
Neuropharmacology
- Anxiolytic Activity : Binding to benzodiazepine receptors may confer anxiolytic properties, making it a candidate for anxiety treatment.
- Neuroprotective Effects : Preliminary studies suggest that this compound may protect neuronal cells from toxic insults, indicating potential applications in neurodegenerative diseases .
- Antimicrobial Properties
Biotechnological Applications
- Biosynthesis Studies
- Metabolic Engineering
Case Studies and Research Findings
Q & A
Basic Research Questions
Q. What spectroscopic methods are used to characterize mayumbine’s structural configuration, and how are spectral data interpreted?
this compound’s structural elucidation relies on advanced spectroscopic techniques. Nuclear Magnetic Resonance (NMR) spectroscopy, particularly -NMR, is critical for identifying hydrogen environments and stereochemistry. For instance, distinct peaks at ~3.1 ppm and ~2.7 ppm in this compound’s NMR spectrum indicate specific proton environments, while shifts in derivatives (e.g., [21α-]-mayumbine) reveal stereochemical variations . High-resolution mass spectrometry (HR-MS) and infrared (IR) spectroscopy further validate molecular mass and functional groups. Researchers should cross-reference spectral data with computational models (e.g., DFT calculations) to resolve ambiguities.
Q. What are the standard protocols for isolating this compound from natural sources?
this compound is typically isolated from Catharanthus roseus or related Apocynaceae species using solvent extraction (e.g., methanol/chloroform mixtures) followed by chromatographic purification. Column chromatography (silica gel or Sephadex) and HPLC with reverse-phase C18 columns are employed for separation. Purity is confirmed via thin-layer chromatography (TLC) and ≥95% HPLC peak area thresholds. Researchers must document solvent ratios, temperature, and pressure conditions to ensure reproducibility .
Advanced Research Questions
Q. How do enzymatic pathways govern this compound biosynthesis, and what genetic tools enable pathway modulation?
this compound biosynthesis involves heteroyohimbine synthase (HYS), an enzyme that catalyzes the conversion of strictosidine aglycone to this compound via stereospecific reactions. Heterologous expression in yeast (Saccharomyces cerevisiae) with codon-optimized HYS genes (e.g., from Catharanthus roseus) allows pathway engineering. Researchers should use CRISPR-Cas9 for gene knockout/knock-in studies and LC-MS/MS to track intermediate metabolites (e.g., ajmalicine, tetrahydroalstonine). Enzyme kinetics assays (Michaelis-Menten parameters) further elucidate catalytic efficiency .
Q. What strategies resolve contradictions in structural data between this compound and its derivatives?
Discrepancies in NMR or crystallographic data (e.g., conflicting proton coupling constants) may arise from stereochemical variations or sample impurities. To resolve these:
- Perform 2D NMR (COSY, NOESY) to confirm spatial relationships between protons.
- Compare experimental data with synthetic standards or published spectra in databases like PubChem.
- Utilize X-ray diffraction for absolute configuration determination. Contradictions should be analyzed through iterative hypothesis testing, leveraging peer-reviewed structural databases and collaborative validation .
Q. How is stereoselectivity controlled during this compound synthesis, and what role do catalysts play?
Stereoselective synthesis of this compound requires chiral catalysts (e.g., organocatalysts or metal-ligand complexes) to direct enantiomeric outcomes. Asymmetric hydrogenation and enzymatic resolution (e.g., lipase-mediated kinetic resolution) are common strategies. Researchers must optimize reaction conditions (temperature, solvent polarity) and characterize enantiomeric excess (ee) via chiral HPLC or circular dichroism (CD). Computational docking studies can predict catalyst-substrate interactions to refine stereochemical control .
Q. What experimental designs are recommended for comparative studies of this compound’s bioactivity against related alkaloids?
- In vitro assays : Use competitive binding assays (e.g., radioligand displacement for benzodiazepine receptors) with IC calculations.
- Dose-response curves : Compare this compound with ajmalicine or tetrahydroalstonine using standardized cell lines (e.g., HEK-293 for receptor studies).
- Statistical rigor : Apply ANOVA with post-hoc Tukey tests to assess significance (p < 0.05). Include negative controls (e.g., vehicle-only) and triplicate runs. Document protocols in alignment with journal guidelines (e.g., Beilstein Journal of Organic Chemistry) to ensure reproducibility .
Q. Methodological Considerations
- Data validation : Cross-check spectral and bioactivity data with open-access repositories (e.g., ChEMBL, UniProt) to mitigate confirmation bias .
- Ethical reporting : Disclose synthetic yields, purification losses, and assay limitations to enhance transparency .
- Collaborative frameworks : Share raw NMR/MS data via platforms like Zenodo to facilitate peer validation .
Comparación Con Compuestos Similares
Comparison with Similar Compounds
Functional and Pharmacological Differences
Receptor Specificity :
- Mayumbine : Binds to benzodiazepine receptors, modulating GABAergic activity .
- Ajmalicine : α1-Adrenergic receptor antagonist; used clinically for vascular disorders .
- Tetrahydroalstonine : Exhibits hypotensive effects but lacks significant receptor selectivity .
- Rauwolscine : α2-Adrenergic receptor antagonist with applications in metabolic studies .
Biosynthetic Pathways: Enzymes such as HYS and THAS catalyze the stereoselective reduction of strictosidine aglycone. HYS produces a racemic mixture of ajmalicine and this compound (55:15 ratio), while THAS enzymes favor tetrahydroalstonine (85%) with minor this compound (15%) . The AMS enzyme in C. roseus generates ajmalicine and this compound as a racemic mixture, highlighting evolutionary divergence in biosynthetic routes .
Commercial Availability and Cost :
this compound (¥3750/5mg) is significantly more expensive than tetrahydroalstonine (¥1350/5mg), likely due to lower natural abundance and specialized applications in receptor studies .
Research Findings
Enzyme Structure-Activity Relationships: Crystal structures of HYS and THAS enzymes reveal a critical amino acid loop (residues 210–220) that governs stereochemical outcomes. Mutations in this region alter product ratios, enabling engineered production of specific alkaloids . For example, substituting Val215 in THAS3 with bulkier residues increases this compound yield by 20% .
Comparative Efficacy in Receptor Binding: this compound’s benzodiazepine affinity (Ki = 120 nM) is comparable to diazepam (Ki = 20 nM), but its α1-adrenergic activity is 10-fold weaker than ajmalicine . In rat vas deferens assays, this compound showed negligible pre-synaptic α2-adrenoceptor blockade, unlike rauwolscine, which inhibited norepinephrine release by 70% .
Biosynthetic Engineering Potential: Co-expression of HYS and P450 oxidases in yeast yielded novel this compound derivatives with enhanced blood-brain barrier permeability, underscoring its utility in CNS drug development .
Propiedades
IUPAC Name |
methyl (1S,15R,16R,20S)-16-methyl-17-oxa-3,13-diazapentacyclo[11.8.0.02,10.04,9.015,20]henicosa-2(10),4,6,8,18-pentaene-19-carboxylate | |
---|---|---|
Source | PubChem | |
URL | https://pubchem.ncbi.nlm.nih.gov | |
Description | Data deposited in or computed by PubChem | |
InChI |
InChI=1S/C21H24N2O3/c1-12-16-10-23-8-7-14-13-5-3-4-6-18(13)22-20(14)19(23)9-15(16)17(11-26-12)21(24)25-2/h3-6,11-12,15-16,19,22H,7-10H2,1-2H3/t12-,15+,16-,19+/m1/s1 | |
Source | PubChem | |
URL | https://pubchem.ncbi.nlm.nih.gov | |
Description | Data deposited in or computed by PubChem | |
InChI Key |
GRTOGORTSDXSFK-XIEZEKGWSA-N | |
Source | PubChem | |
URL | https://pubchem.ncbi.nlm.nih.gov | |
Description | Data deposited in or computed by PubChem | |
Canonical SMILES |
CC1C2CN3CCC4=C(C3CC2C(=CO1)C(=O)OC)NC5=CC=CC=C45 | |
Source | PubChem | |
URL | https://pubchem.ncbi.nlm.nih.gov | |
Description | Data deposited in or computed by PubChem | |
Isomeric SMILES |
C[C@@H]1[C@H]2CN3CCC4=C([C@@H]3C[C@@H]2C(=CO1)C(=O)OC)NC5=CC=CC=C45 | |
Source | PubChem | |
URL | https://pubchem.ncbi.nlm.nih.gov | |
Description | Data deposited in or computed by PubChem | |
Molecular Formula |
C21H24N2O3 | |
Source | PubChem | |
URL | https://pubchem.ncbi.nlm.nih.gov | |
Description | Data deposited in or computed by PubChem | |
DSSTOX Substance ID |
DTXSID501316603 | |
Record name | 19-Epiajmalicine | |
Source | EPA DSSTox | |
URL | https://comptox.epa.gov/dashboard/DTXSID501316603 | |
Description | DSSTox provides a high quality public chemistry resource for supporting improved predictive toxicology. | |
Molecular Weight |
352.4 g/mol | |
Source | PubChem | |
URL | https://pubchem.ncbi.nlm.nih.gov | |
Description | Data deposited in or computed by PubChem | |
CAS No. |
25532-45-0 | |
Record name | 19-Epiajmalicine | |
Source | CAS Common Chemistry | |
URL | https://commonchemistry.cas.org/detail?cas_rn=25532-45-0 | |
Description | CAS Common Chemistry is an open community resource for accessing chemical information. Nearly 500,000 chemical substances from CAS REGISTRY cover areas of community interest, including common and frequently regulated chemicals, and those relevant to high school and undergraduate chemistry classes. This chemical information, curated by our expert scientists, is provided in alignment with our mission as a division of the American Chemical Society. | |
Explanation | The data from CAS Common Chemistry is provided under a CC-BY-NC 4.0 license, unless otherwise stated. | |
Record name | 19-epi-Ajmalicine | |
Source | ChemIDplus | |
URL | https://pubchem.ncbi.nlm.nih.gov/substance/?source=chemidplus&sourceid=0025532450 | |
Description | ChemIDplus is a free, web search system that provides access to the structure and nomenclature authority files used for the identification of chemical substances cited in National Library of Medicine (NLM) databases, including the TOXNET system. | |
Record name | 19-Epiajmalicine | |
Source | EPA DSSTox | |
URL | https://comptox.epa.gov/dashboard/DTXSID501316603 | |
Description | DSSTox provides a high quality public chemistry resource for supporting improved predictive toxicology. | |
Retrosynthesis Analysis
AI-Powered Synthesis Planning: Our tool employs the Template_relevance Pistachio, Template_relevance Bkms_metabolic, Template_relevance Pistachio_ringbreaker, Template_relevance Reaxys, Template_relevance Reaxys_biocatalysis model, leveraging a vast database of chemical reactions to predict feasible synthetic routes.
One-Step Synthesis Focus: Specifically designed for one-step synthesis, it provides concise and direct routes for your target compounds, streamlining the synthesis process.
Accurate Predictions: Utilizing the extensive PISTACHIO, BKMS_METABOLIC, PISTACHIO_RINGBREAKER, REAXYS, REAXYS_BIOCATALYSIS database, our tool offers high-accuracy predictions, reflecting the latest in chemical research and data.
Strategy Settings
Precursor scoring | Relevance Heuristic |
---|---|
Min. plausibility | 0.01 |
Model | Template_relevance |
Template Set | Pistachio/Bkms_metabolic/Pistachio_ringbreaker/Reaxys/Reaxys_biocatalysis |
Top-N result to add to graph | 6 |
Feasible Synthetic Routes
Descargo de responsabilidad e información sobre productos de investigación in vitro
Tenga en cuenta que todos los artículos e información de productos presentados en BenchChem están destinados únicamente con fines informativos. Los productos disponibles para la compra en BenchChem están diseñados específicamente para estudios in vitro, que se realizan fuera de organismos vivos. Los estudios in vitro, derivados del término latino "in vidrio", involucran experimentos realizados en entornos de laboratorio controlados utilizando células o tejidos. Es importante tener en cuenta que estos productos no se clasifican como medicamentos y no han recibido la aprobación de la FDA para la prevención, tratamiento o cura de ninguna condición médica, dolencia o enfermedad. Debemos enfatizar que cualquier forma de introducción corporal de estos productos en humanos o animales está estrictamente prohibida por ley. Es esencial adherirse a estas pautas para garantizar el cumplimiento de los estándares legales y éticos en la investigación y experimentación.