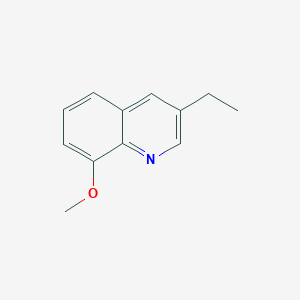
3-Ethyl-8-methoxyquinoline
Descripción general
Descripción
El ácido oxidrónico, también conocido como ácido (hidroximetileno)bisfosfónico, es un compuesto bisfosfonato con la fórmula molecular CH₆O₇P₂ y un peso molecular de 192,00 g/mol . Se utiliza principalmente como agente de imagenología esquelética de diagnóstico para demostrar áreas de osteogénesis alterada en pacientes adultos y pediátricos . El compuesto es conocido por su capacidad de unirse a la hidroxiapatita en el hueso, lo que lo hace útil en diversas aplicaciones médicas y científicas.
Métodos De Preparación
Rutas sintéticas y condiciones de reacción
La síntesis del ácido oxidrónico generalmente implica la reacción de ácido fosforoso (H₃PO₃) con formaldehído (CH₂O) en condiciones controladas. La reacción se lleva a cabo en un medio acuoso, y el producto se aísla por cristalización . La reacción general se puede representar como sigue:
H₃PO₃+CH₂O→CH₆O₇P₂
Métodos de producción industrial
La producción industrial del ácido oxidrónico sigue rutas sintéticas similares, pero a mayor escala. El proceso implica el uso de reactivos de alta pureza y condiciones de reacción controladas para garantizar la calidad constante del producto final. La mezcla de reacción se calienta normalmente para facilitar la reacción, y el producto se purifica mediante cristalización y filtración .
Análisis De Reacciones Químicas
Tipos de reacciones
El ácido oxidrónico experimenta varios tipos de reacciones químicas, incluyendo:
Oxidación: El ácido oxidrónico puede oxidarse para formar varios productos de oxidación.
Reducción: El compuesto puede reducirse en condiciones específicas para producir formas reducidas.
Reactivos y condiciones comunes
Oxidación: Los agentes oxidantes comunes incluyen permanganato de potasio (KMnO₄) y peróxido de hidrógeno (H₂O₂).
Reducción: Se utilizan agentes reductores como borohidruro de sodio (NaBH₄) e hidruro de aluminio y litio (LiAlH₄).
Principales productos formados
Los principales productos formados a partir de estas reacciones dependen de los reactivos y condiciones específicos utilizados. Por ejemplo, la oxidación del ácido oxidrónico puede producir varios derivados de ácido fosfónico, mientras que la reducción puede producir ácido hidroximetilfosfónico .
Aplicaciones Científicas De Investigación
Antimicrobial Properties
Research indicates that compounds in the quinoline family, including 3-ethyl-8-methoxyquinoline, exhibit notable antimicrobial activities. Quinoline derivatives have been shown to inhibit various pathogens, suggesting that this compound may disrupt bacterial cell wall synthesis or viral replication mechanisms. For instance, similar compounds have demonstrated efficacy in reducing intracellular viral protein production, indicating potential applications in antiviral therapies.
Anticancer Potential
Quinoline derivatives are also being investigated for their anticancer properties. Studies have shown that modifications in the quinoline structure can significantly alter their interaction profiles with cancer cells. The unique substitution pattern of this compound may enhance its efficacy against specific cancer types, warranting further investigation into its structure-activity relationships (SARs) to optimize its therapeutic potential .
Materials Science Applications
This compound can also be utilized in materials science, particularly as a component in organic light-emitting diodes (OLEDs). The compound's ability to act as an electron carrier makes it suitable for applications in electronic devices, where efficient charge transport is essential .
Synthesis and Characterization
The synthesis of this compound typically involves alkylation reactions using ethyl halides on 8-methoxyquinoline. Various methods can yield this compound, including:
- Alkylation : Involves the reaction of 8-methoxyquinoline with ethyl halides in the presence of a base.
- Condensation Reactions : Alternative synthetic routes may include condensation reactions that facilitate the formation of the desired compound.
Characterization of synthesized compounds is crucial for confirming their structure and purity. Techniques such as NMR spectroscopy and mass spectrometry are commonly employed to validate the identity of this compound .
Case Study 1: Antiviral Activity
A study investigating similar quinoline derivatives found that they exhibited significant antiviral activity against various viral strains. The mechanisms involved included inhibition of viral replication and interference with viral protein synthesis. This suggests that this compound could be explored further for its antiviral properties through both in-silico and in vitro studies.
Case Study 2: Antimicrobial Efficacy
In another investigation focused on antimicrobial activity, quinoline derivatives were tested against several bacterial strains. The results indicated that modifications to the quinoline structure could enhance antibacterial efficacy, particularly against antibiotic-resistant strains. This highlights the potential role of this compound as a lead compound for developing new antimicrobial agents .
Mecanismo De Acción
El ácido oxidrónico ejerce sus efectos principalmente uniéndose a la hidroxiapatita en el tejido óseo. Esta unión inhibe la actividad de los osteoclastos, las células responsables de la resorción ósea. El compuesto es captado por los osteoclastos a través de la endocitosis de fase fluida, y una vez dentro de las células, interrumpe la vía del mevalonato, lo que lleva a la inhibición de la resorción ósea . Este mecanismo es similar al de otros bisfosfonatos, lo que hace que el ácido oxidrónico sea eficaz en el tratamiento de afecciones relacionadas con la resorción ósea excesiva .
Comparación Con Compuestos Similares
Compuestos similares
Ácido zoledrónico: Otro bisfosfonato utilizado para tratar enfermedades óseas.
Alendronato: Utilizado para tratar la osteoporosis, tiene una estructura química diferente pero pertenece a la misma clase de bisfosfonatos.
Singularidad
El ácido oxidrónico es único en su afinidad de unión específica a la hidroxiapatita y sus aplicaciones de diagnóstico en la imagenología esquelética. Su capacidad para demostrar áreas de osteogénesis alterada lo hace particularmente valioso en el diagnóstico médico .
Actividad Biológica
3-Ethyl-8-methoxyquinoline is a quinoline derivative with notable biological activities, including antimicrobial and antiviral properties. This compound, characterized by its unique molecular structure, has gained attention for its potential applications in various therapeutic fields.
Chemical Structure and Properties
- Molecular Formula : C₁₂H₁₃N₁O
- Molecular Weight : Approximately 199.24 g/mol
- Structural Features : The compound features an ethyl group at the 3-position and a methoxy group at the 8-position, contributing to its distinctive chemical properties and biological activities.
Biological Activity
Research indicates that this compound exhibits several significant biological activities:
- Antimicrobial Activity : The compound demonstrates effectiveness against various bacterial strains. For instance, studies have shown it can inhibit the growth of Pseudomonas aeruginosa and Klebsiella pneumoniae, with inhibition zones comparable to standard antibiotics .
- Antiviral Properties : In vitro studies suggest that this compound can disrupt viral replication mechanisms, potentially reducing the intracellular production of viral proteins. This indicates its promise as a candidate for antiviral therapies .
The mechanisms through which this compound exerts its biological effects are multifaceted:
- Disruption of Cell Wall Synthesis : Similar compounds in the quinoline family are known to inhibit bacterial cell wall synthesis, leading to cell lysis.
- Inhibition of Viral Replication : The compound may interfere with viral entry or replication within host cells, an area that warrants further exploration through in vitro and in vivo studies .
Comparative Analysis with Related Compounds
The following table summarizes some related quinoline derivatives and their notable properties:
Compound Name | Structure Features | Notable Properties |
---|---|---|
8-Hydroxyquinoline | Hydroxyl group at position 8 | Strong chelating agent |
4-Methylquinoline | Methyl group at position 4 | Antimicrobial activity |
5-Ethylquinolin-8-ol | Hydroxyl group at position 8 with ethyl substitution | Antiviral properties |
6-Methoxyquinoline | Methoxy group at position 6 | Anticancer activity |
The unique substitution pattern of this compound influences its solubility, reactivity, and biological activity compared to these similar compounds .
Case Studies and Research Findings
Recent studies have highlighted the potential of quinoline derivatives, including this compound, in addressing antibiotic resistance:
- A study reported that derivatives exhibited higher antimicrobial activity than standard drugs against resistant strains like Staphylococcus aureus, with minimum inhibitory concentration (MIC) values indicating strong efficacy .
- Another investigation focused on the synthesis of novel quinoline derivatives for enhanced antiviral activity against influenza viruses, demonstrating that modifications in the chemical structure could significantly impact their bioactivity .
Future Directions
The promising biological activity of this compound suggests several avenues for future research:
- In Vivo Studies : Conducting animal studies to evaluate the pharmacokinetics and therapeutic potential.
- Mechanistic Studies : Investigating the specific pathways through which this compound exerts its antimicrobial and antiviral effects.
- Formulation Development : Exploring formulations that enhance bioavailability and target delivery to maximize therapeutic efficacy.
Propiedades
IUPAC Name |
3-ethyl-8-methoxyquinoline | |
---|---|---|
Source | PubChem | |
URL | https://pubchem.ncbi.nlm.nih.gov | |
Description | Data deposited in or computed by PubChem | |
InChI |
InChI=1S/C12H13NO/c1-3-9-7-10-5-4-6-11(14-2)12(10)13-8-9/h4-8H,3H2,1-2H3 | |
Source | PubChem | |
URL | https://pubchem.ncbi.nlm.nih.gov | |
Description | Data deposited in or computed by PubChem | |
InChI Key |
QBVUVMNSJBCGOL-UHFFFAOYSA-N | |
Source | PubChem | |
URL | https://pubchem.ncbi.nlm.nih.gov | |
Description | Data deposited in or computed by PubChem | |
Canonical SMILES |
CCC1=CN=C2C(=C1)C=CC=C2OC | |
Source | PubChem | |
URL | https://pubchem.ncbi.nlm.nih.gov | |
Description | Data deposited in or computed by PubChem | |
Molecular Formula |
C12H13NO | |
Source | PubChem | |
URL | https://pubchem.ncbi.nlm.nih.gov | |
Description | Data deposited in or computed by PubChem | |
DSSTOX Substance ID |
DTXSID30553817 | |
Record name | 3-Ethyl-8-methoxyquinoline | |
Source | EPA DSSTox | |
URL | https://comptox.epa.gov/dashboard/DTXSID30553817 | |
Description | DSSTox provides a high quality public chemistry resource for supporting improved predictive toxicology. | |
Molecular Weight |
187.24 g/mol | |
Source | PubChem | |
URL | https://pubchem.ncbi.nlm.nih.gov | |
Description | Data deposited in or computed by PubChem | |
CAS No. |
112955-03-0 | |
Record name | 3-Ethyl-8-methoxyquinoline | |
Source | EPA DSSTox | |
URL | https://comptox.epa.gov/dashboard/DTXSID30553817 | |
Description | DSSTox provides a high quality public chemistry resource for supporting improved predictive toxicology. | |
Synthesis routes and methods
Procedure details
Retrosynthesis Analysis
AI-Powered Synthesis Planning: Our tool employs the Template_relevance Pistachio, Template_relevance Bkms_metabolic, Template_relevance Pistachio_ringbreaker, Template_relevance Reaxys, Template_relevance Reaxys_biocatalysis model, leveraging a vast database of chemical reactions to predict feasible synthetic routes.
One-Step Synthesis Focus: Specifically designed for one-step synthesis, it provides concise and direct routes for your target compounds, streamlining the synthesis process.
Accurate Predictions: Utilizing the extensive PISTACHIO, BKMS_METABOLIC, PISTACHIO_RINGBREAKER, REAXYS, REAXYS_BIOCATALYSIS database, our tool offers high-accuracy predictions, reflecting the latest in chemical research and data.
Strategy Settings
Precursor scoring | Relevance Heuristic |
---|---|
Min. plausibility | 0.01 |
Model | Template_relevance |
Template Set | Pistachio/Bkms_metabolic/Pistachio_ringbreaker/Reaxys/Reaxys_biocatalysis |
Top-N result to add to graph | 6 |
Feasible Synthetic Routes
Descargo de responsabilidad e información sobre productos de investigación in vitro
Tenga en cuenta que todos los artículos e información de productos presentados en BenchChem están destinados únicamente con fines informativos. Los productos disponibles para la compra en BenchChem están diseñados específicamente para estudios in vitro, que se realizan fuera de organismos vivos. Los estudios in vitro, derivados del término latino "in vidrio", involucran experimentos realizados en entornos de laboratorio controlados utilizando células o tejidos. Es importante tener en cuenta que estos productos no se clasifican como medicamentos y no han recibido la aprobación de la FDA para la prevención, tratamiento o cura de ninguna condición médica, dolencia o enfermedad. Debemos enfatizar que cualquier forma de introducción corporal de estos productos en humanos o animales está estrictamente prohibida por ley. Es esencial adherirse a estas pautas para garantizar el cumplimiento de los estándares legales y éticos en la investigación y experimentación.