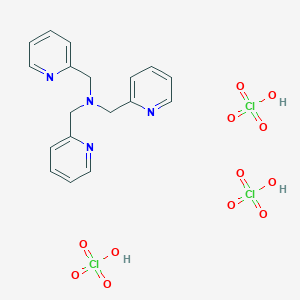
Tris((2-pyridinium)methyl)amine
Descripción general
Descripción
Tris(2-pyridylmethyl)amine triperchlorate is a coordination compound that features a tripodal ligand, tris(2-pyridylmethyl)amine, complexed with perchlorate ions. This compound is known for its ability to form stable complexes with various metals, making it a valuable ligand in coordination chemistry and catalysis.
Métodos De Preparación
Synthetic Routes and Reaction Conditions: The synthesis of tris(2-pyridylmethyl)amine typically involves the alkylation of 2-picolylamine with picolyl chloride. The reaction proceeds as follows:
2C5H4NCH2Cl+C5H4NCH2NH2→(C5H4NCH2)3N+2HCl
This reaction is carried out in an aqueous sodium hydroxide solution to facilitate the formation of the tris(2-pyridylmethyl)amine ligand .
Industrial Production Methods: Industrial production of tris(2-pyridylmethyl)amine triperchlorate involves similar synthetic routes but on a larger scale. The process includes the careful control of reaction conditions to ensure high yields and purity of the final product. The use of automated reactors and continuous flow systems can enhance the efficiency and scalability of the production process.
Análisis De Reacciones Químicas
Types of Reactions: Tris(2-pyridylmethyl)amine triperchlorate undergoes various types of chemical reactions, including:
Oxidation: The ligand can participate in oxidation reactions, often facilitated by metal complexes.
Reduction: Reduction reactions involving the ligand are less common but can occur under specific conditions.
Substitution: The ligand can undergo substitution reactions, where one or more of its pyridyl groups are replaced by other functional groups.
Common Reagents and Conditions:
Oxidation: Common oxidizing agents include hydrogen peroxide and potassium permanganate.
Reduction: Reducing agents such as sodium borohydride can be used.
Substitution: Substitution reactions often require the presence of a strong base or acid to facilitate the exchange of functional groups.
Major Products: The major products formed from these reactions depend on the specific reagents and conditions used. For example, oxidation reactions may yield oxidized derivatives of the ligand, while substitution reactions can produce a variety of functionalized tris(2-pyridylmethyl)amine derivatives .
Aplicaciones Científicas De Investigación
Mecanismo De Acción
The mechanism by which tris(2-pyridylmethyl)amine triperchlorate exerts its effects involves its ability to form stable complexes with metal ions. The ligand’s tripodal structure allows it to coordinate with metal centers in a precise geometry, facilitating various catalytic and coordination processes. The molecular targets and pathways involved depend on the specific metal ion and the context of the reaction or application .
Comparación Con Compuestos Similares
Tris(2-picolyl)amine: Similar to tris(2-pyridylmethyl)amine, this ligand also forms stable complexes with metals but may exhibit different coordination properties due to slight structural differences.
Tris(2-quinolylmethyl)amine: This ligand has a similar tripodal structure but with quinoline groups instead of pyridine, leading to different electronic and steric effects.
Uniqueness: Tris(2-pyridylmethyl)amine triperchlorate is unique due to its ability to form highly stable and versatile metal complexes. Its tripodal structure provides a robust framework for coordination, making it a valuable ligand in various fields of research and industry .
Actividad Biológica
Tris((2-pyridinium)methyl)amine (TPMA) is a compound that has garnered attention for its diverse biological activities, particularly in the fields of medicinal chemistry and biochemistry. This article aims to provide a comprehensive overview of TPMA’s biological activity, including its mechanisms of action, potential therapeutic applications, and relevant case studies.
Overview of this compound
TPMA is a ligand that coordinates with various metal ions, enhancing their biological reactivity. Its structure comprises three pyridyl groups attached to a central nitrogen atom, which allows it to interact with biological targets effectively. The compound has been studied for its anticancer properties, insulin-mimetic activities, and potential applications in drug development.
Anticancer Activity
TPMA has demonstrated significant anticancer activity, particularly against osteosarcoma cell lines. Research indicates that TPMA can act as a cationic species that interacts with anionic vanadate complexes, enhancing their therapeutic efficacy while reducing toxicity. For instance, studies have shown that vanadium complexes with TPMA exhibit improved cellular uptake and cytotoxicity against cancer cells compared to their non-complexed counterparts .
Case Study: Vanadium Complexes
A study explored the synthesis of vanadium(V) complexes with TPMA as ligands. These complexes were characterized using infrared spectroscopy and X-ray diffraction methods. The findings suggested that the protective cationic environment provided by TPMA enhances the stability and activity of vanadium species in physiological conditions .
Complex | Activity | Mechanism |
---|---|---|
V(TPMA) | Anticancer in osteosarcoma cells | Enhances cellular uptake and reduces toxicity |
Cu(TPMA)(Phen) | Artificial nuclease | Facilitates oxidation reactions |
Insulin-Mimetic Properties
TPMA has also been investigated for its insulin-mimetic properties. Studies indicate that TPMA and its metal complexes can mimic insulin's effects on glucose metabolism. Specifically, bis- and tris(pyridyl)amine-oxidovanadium complexes have shown promise in activating insulin signaling pathways, which may lead to novel treatments for diabetes .
Antichlamydial Activity
Recent research has highlighted the potential of TPMA derivatives in combating Chlamydia trachomatis, a major cause of sexually transmitted infections. Compounds based on TPMA have been shown to selectively inhibit the growth of this pathogen without affecting host cell viability. This selectivity is crucial for developing new therapeutic agents targeting chlamydial infections .
The biological activity of TPMA can be attributed to several mechanisms:
- Metal Coordination : The ability of TPMA to coordinate with transition metals enhances the reactivity of these metals in biological systems.
- Reactive Oxygen Species (ROS) Generation : Some TPMA complexes can generate ROS, contributing to their cytotoxic effects on cancer cells.
- Selective Targeting : The structural features of TPMA allow for selective interactions with specific biological targets, such as enzymes or receptors involved in disease processes.
Propiedades
IUPAC Name |
perchloric acid;1-pyridin-2-yl-N,N-bis(pyridin-2-ylmethyl)methanamine | |
---|---|---|
Source | PubChem | |
URL | https://pubchem.ncbi.nlm.nih.gov | |
Description | Data deposited in or computed by PubChem | |
InChI |
InChI=1S/C18H18N4.3ClHO4/c1-4-10-19-16(7-1)13-22(14-17-8-2-5-11-20-17)15-18-9-3-6-12-21-18;3*2-1(3,4)5/h1-12H,13-15H2;3*(H,2,3,4,5) | |
Source | PubChem | |
URL | https://pubchem.ncbi.nlm.nih.gov | |
Description | Data deposited in or computed by PubChem | |
InChI Key |
ZZDOWDOZOOGZKC-UHFFFAOYSA-N | |
Source | PubChem | |
URL | https://pubchem.ncbi.nlm.nih.gov | |
Description | Data deposited in or computed by PubChem | |
Canonical SMILES |
C1=CC=NC(=C1)CN(CC2=CC=CC=N2)CC3=CC=CC=N3.OCl(=O)(=O)=O.OCl(=O)(=O)=O.OCl(=O)(=O)=O | |
Source | PubChem | |
URL | https://pubchem.ncbi.nlm.nih.gov | |
Description | Data deposited in or computed by PubChem | |
Molecular Formula |
C18H21Cl3N4O12 | |
Source | PubChem | |
URL | https://pubchem.ncbi.nlm.nih.gov | |
Description | Data deposited in or computed by PubChem | |
DSSTOX Substance ID |
DTXSID70151871 | |
Record name | Tris((2-pyridinium)methyl)amine | |
Source | EPA DSSTox | |
URL | https://comptox.epa.gov/dashboard/DTXSID70151871 | |
Description | DSSTox provides a high quality public chemistry resource for supporting improved predictive toxicology. | |
Molecular Weight |
591.7 g/mol | |
Source | PubChem | |
URL | https://pubchem.ncbi.nlm.nih.gov | |
Description | Data deposited in or computed by PubChem | |
CAS No. |
117689-07-3 | |
Record name | Tris((2-pyridinium)methyl)amine | |
Source | ChemIDplus | |
URL | https://pubchem.ncbi.nlm.nih.gov/substance/?source=chemidplus&sourceid=0117689073 | |
Description | ChemIDplus is a free, web search system that provides access to the structure and nomenclature authority files used for the identification of chemical substances cited in National Library of Medicine (NLM) databases, including the TOXNET system. | |
Record name | Tris((2-pyridinium)methyl)amine | |
Source | EPA DSSTox | |
URL | https://comptox.epa.gov/dashboard/DTXSID70151871 | |
Description | DSSTox provides a high quality public chemistry resource for supporting improved predictive toxicology. | |
Retrosynthesis Analysis
AI-Powered Synthesis Planning: Our tool employs the Template_relevance Pistachio, Template_relevance Bkms_metabolic, Template_relevance Pistachio_ringbreaker, Template_relevance Reaxys, Template_relevance Reaxys_biocatalysis model, leveraging a vast database of chemical reactions to predict feasible synthetic routes.
One-Step Synthesis Focus: Specifically designed for one-step synthesis, it provides concise and direct routes for your target compounds, streamlining the synthesis process.
Accurate Predictions: Utilizing the extensive PISTACHIO, BKMS_METABOLIC, PISTACHIO_RINGBREAKER, REAXYS, REAXYS_BIOCATALYSIS database, our tool offers high-accuracy predictions, reflecting the latest in chemical research and data.
Strategy Settings
Precursor scoring | Relevance Heuristic |
---|---|
Min. plausibility | 0.01 |
Model | Template_relevance |
Template Set | Pistachio/Bkms_metabolic/Pistachio_ringbreaker/Reaxys/Reaxys_biocatalysis |
Top-N result to add to graph | 6 |
Feasible Synthetic Routes
Descargo de responsabilidad e información sobre productos de investigación in vitro
Tenga en cuenta que todos los artículos e información de productos presentados en BenchChem están destinados únicamente con fines informativos. Los productos disponibles para la compra en BenchChem están diseñados específicamente para estudios in vitro, que se realizan fuera de organismos vivos. Los estudios in vitro, derivados del término latino "in vidrio", involucran experimentos realizados en entornos de laboratorio controlados utilizando células o tejidos. Es importante tener en cuenta que estos productos no se clasifican como medicamentos y no han recibido la aprobación de la FDA para la prevención, tratamiento o cura de ninguna condición médica, dolencia o enfermedad. Debemos enfatizar que cualquier forma de introducción corporal de estos productos en humanos o animales está estrictamente prohibida por ley. Es esencial adherirse a estas pautas para garantizar el cumplimiento de los estándares legales y éticos en la investigación y experimentación.