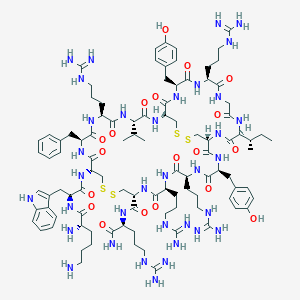
Tachyplesin I
Descripción general
Descripción
Synthesis Analysis
The synthesis of Tachyplesin I and its derivatives emphasizes chemical modifications to enhance therapeutic efficacy and stability. Advances in synthetic techniques have allowed for the production of this compound analogs with improved antimicrobial and antitumor properties, illustrating the peptide's adaptability and potential for tailored therapeutic applications.
Molecular Structure Analysis
This compound's structure is characterized by a β-sheet configuration that contributes to its stability and function. This structural motif is essential for its interaction with microbial membranes and cancer cells, leading to its potent bioactivity. The peptide's structure-activity relationship highlights the importance of its conformation in mediating its dual antimicrobial and antitumor effects.
Chemical Reactions and Properties
Chemically, this compound is resistant to proteolytic degradation, which is pivotal for its function in the hostile environments of microbial infection and tumor growth. Its amino acid composition and cyclic structure confer a significant resistance to enzymatic breakdown, enhancing its longevity and effectiveness in biological systems.
Physical Properties Analysis
The physical properties of this compound, such as its solubility, stability, and amphipathicity, are crucial for its biological functions. These properties facilitate the peptide's interaction with lipid membranes of pathogens and cancer cells, leading to membrane disruption and the subsequent antimicrobial and antitumor activities.
Chemical Properties Analysis
This compound exhibits a broad spectrum of chemical interactions, primarily through its ability to bind to and disrupt cellular membranes. This action is mediated by its positive charge and hydrophobic regions, which enable it to insert into lipid bilayers and exert its cytotoxic effects on target cells.
Aplicaciones Científicas De Investigación
Antitumor Activity : Tachyplesin I may inhibit migration and promote apoptosis in glioma cells, contributing to its antitumor function. This was highlighted in a study on U251 Gliomaspheres, suggesting its potential in cancer therapy (Li et al., 2017).
Antimicrobial and Antifungal Properties : It is known for inhibiting the growth of bacteria and fungi, acting as a self-defense mechanism in horseshoe crabs against invading microorganisms. This property is shared by this compound and its isopeptides, tachyplesin II and polyphemusins I and II (Miyata et al., 1989).
Potential in Antimicrobial Drug Development : Tachyplesins, including this compound, found in Southeast Asian horseshoe crab hemocytes, are considered for antimicrobial drug development due to their broad-spectrum activity (Muta et al., 1990).
Apoptosis Induction : A variant, RGD-tachyplesin, has been shown to inhibit tumor growth and proliferation both in vitro and in vivo, with apoptotic molecules involved in the induction of apoptosis, indicating its potential in cancer therapy (Chen et al., 2001).
Lipopolysaccharide-Binding Properties : this compound, being a lipopolysaccharide-binding peptide, is useful in research involving bacterial endotoxins (Shieh et al., 1989).
Aquaculture Applications : In aquaculture, this compound can reduce the viability of bivalve pathogens, suggesting its use in producing disease-resistant bivalves through genetic manipulation (Morvan et al., 1997).
Membrane Disruption in Bacteria : It disrupts Escherichia coli outer membrane and mimics bacterial inner membranes, making it a potent antimicrobial agent (Ramamoorthy et al., 2006).
Complement Activation in Tumor Cells : Tachyplesin activates the classic complement pathway to kill tumor cells by increasing membrane permeability (Chen et al., 2005).
Safety as an Antibacterial Drug : The safety of this compound as an antibacterial drug is under exploration due to its therapeutic potential for infections, tumors, and viruses (Hong et al., 2016).
Mecanismo De Acción
Safety and Hazards
Direcciones Futuras
Tachyplesin I, a peptide with dual antimicrobial and antitumor effects, holds great promise as a therapeutic alternative for diseases, with the advantage of broad-spectrum activities, quick killing efficacy, and a low tendency to induce resistance . The continuous advancements in chemical modification and innovative applications of this compound give hope for future improvements in therapeutic efficacy .
Propiedades
IUPAC Name |
(1R,4S,7S,10S,13R,18R,21S,24S,27S,30R,33S,39S,42S)-N-[(2S)-1-amino-5-carbamimidamido-1-oxopentan-2-yl]-10-benzyl-33-[(2S)-butan-2-yl]-7,21,24,39-tetrakis(3-carbamimidamidopropyl)-13-[[(2S)-2-[[(2S)-2,6-diaminohexanoyl]amino]-3-(1H-indol-3-yl)propanoyl]amino]-27,42-bis[(4-hydroxyphenyl)methyl]-3,6,9,12,20,23,26,29,32,35,38,41,44-tridecaoxo-4-propan-2-yl-15,16,46,47-tetrathia-2,5,8,11,19,22,25,28,31,34,37,40,43-tridecazabicyclo[28.14.4]octatetracontane-18-carboxamide | |
---|---|---|
Source | PubChem | |
URL | https://pubchem.ncbi.nlm.nih.gov | |
Description | Data deposited in or computed by PubChem | |
InChI |
InChI=1S/C99H151N35O19S4/c1-5-53(4)78-94(153)132-75-51-157-156-50-74(91(150)127-69(43-55-28-32-58(135)33-29-55)85(144)121-64(24-14-38-114-96(105)106)81(140)119-47-76(137)133-78)131-93(152)77(52(2)3)134-84(143)67(27-17-41-117-99(111)112)124-86(145)68(42-54-18-7-6-8-19-54)126-90(149)73(130-88(147)71(125-80(139)61(101)21-11-12-36-100)45-57-46-118-62-22-10-9-20-60(57)62)49-155-154-48-72(89(148)120-63(79(102)138)23-13-37-113-95(103)104)129-83(142)66(26-16-40-116-98(109)110)122-82(141)65(25-15-39-115-97(107)108)123-87(146)70(128-92(75)151)44-56-30-34-59(136)35-31-56/h6-10,18-20,22,28-35,46,52-53,61,63-75,77-78,118,135-136H,5,11-17,21,23-27,36-45,47-51,100-101H2,1-4H3,(H2,102,138)(H,119,140)(H,120,148)(H,121,144)(H,122,141)(H,123,146)(H,124,145)(H,125,139)(H,126,149)(H,127,150)(H,128,151)(H,129,142)(H,130,147)(H,131,152)(H,132,153)(H,133,137)(H,134,143)(H4,103,104,113)(H4,105,106,114)(H4,107,108,115)(H4,109,110,116)(H4,111,112,117)/t53-,61-,63-,64-,65-,66-,67-,68-,69-,70-,71-,72-,73-,74-,75-,77-,78-/m0/s1 | |
Source | PubChem | |
URL | https://pubchem.ncbi.nlm.nih.gov | |
Description | Data deposited in or computed by PubChem | |
InChI Key |
ZJQFYZCNRTZAIM-PMXBASNASA-N | |
Source | PubChem | |
URL | https://pubchem.ncbi.nlm.nih.gov | |
Description | Data deposited in or computed by PubChem | |
Canonical SMILES |
CCC(C)C1C(=O)NC2CSSCC(C(=O)NC(C(=O)NC(C(=O)NCC(=O)N1)CCCNC(=N)N)CC3=CC=C(C=C3)O)NC(=O)C(NC(=O)C(NC(=O)C(NC(=O)C(CSSCC(NC(=O)C(NC(=O)C(NC(=O)C(NC2=O)CC4=CC=C(C=C4)O)CCCNC(=N)N)CCCNC(=N)N)C(=O)NC(CCCNC(=N)N)C(=O)N)NC(=O)C(CC5=CNC6=CC=CC=C65)NC(=O)C(CCCCN)N)CC7=CC=CC=C7)CCCNC(=N)N)C(C)C | |
Source | PubChem | |
URL | https://pubchem.ncbi.nlm.nih.gov | |
Description | Data deposited in or computed by PubChem | |
Isomeric SMILES |
CC[C@H](C)[C@H]1C(=O)N[C@H]2CSSC[C@@H](C(=O)N[C@H](C(=O)N[C@H](C(=O)NCC(=O)N1)CCCNC(=N)N)CC3=CC=C(C=C3)O)NC(=O)[C@@H](NC(=O)[C@@H](NC(=O)[C@@H](NC(=O)[C@H](CSSC[C@H](NC(=O)[C@@H](NC(=O)[C@@H](NC(=O)[C@@H](NC2=O)CC4=CC=C(C=C4)O)CCCNC(=N)N)CCCNC(=N)N)C(=O)N[C@@H](CCCNC(=N)N)C(=O)N)NC(=O)[C@H](CC5=CNC6=CC=CC=C65)NC(=O)[C@H](CCCCN)N)CC7=CC=CC=C7)CCCNC(=N)N)C(C)C | |
Source | PubChem | |
URL | https://pubchem.ncbi.nlm.nih.gov | |
Description | Data deposited in or computed by PubChem | |
Molecular Formula |
C99H151N35O19S4 | |
Source | PubChem | |
URL | https://pubchem.ncbi.nlm.nih.gov | |
Description | Data deposited in or computed by PubChem | |
Molecular Weight |
2263.8 g/mol | |
Source | PubChem | |
URL | https://pubchem.ncbi.nlm.nih.gov | |
Description | Data deposited in or computed by PubChem | |
CAS RN |
118231-04-2 | |
Record name | Tachyplesin peptide, Tachypleus tridentatus | |
Source | ChemIDplus | |
URL | https://pubchem.ncbi.nlm.nih.gov/substance/?source=chemidplus&sourceid=0118231042 | |
Description | ChemIDplus is a free, web search system that provides access to the structure and nomenclature authority files used for the identification of chemical substances cited in National Library of Medicine (NLM) databases, including the TOXNET system. | |
Q & A
A: Tachyplesin I primarily targets bacterial membranes, exhibiting a higher affinity for negatively charged lipids commonly found in bacterial membranes compared to zwitterionic lipids prevalent in mammalian cell membranes. [, ] This selectivity contributes to its lower toxicity towards mammalian cells. [] Upon interacting with bacterial membranes, this compound can induce several downstream effects:
- Membrane permeabilization: this compound can integrate into the lipid bilayer, disrupting membrane integrity and causing leakage of intracellular contents, ultimately leading to cell death. [, ] This permeabilization effect has been demonstrated in various bacterial species, including Escherichia coli and Staphylococcus aureus. [, ]
- Disruption of membrane potential: this compound can alter the membrane potential of bacteria, leading to an imbalance in ion gradients, which disrupts essential cellular processes and contributes to cell death. []
- Induction of apoptosis-like death: In E. coli, this compound has been shown to induce a cascade of events characteristic of apoptosis-like death, including reactive oxygen species (ROS) generation, membrane depolarization, and DNA fragmentation. []
ANone: this compound is a cationic peptide composed of 17 amino acid residues with the following sequence:
- Spectroscopic Data:
- Nuclear Magnetic Resonance (NMR) Spectroscopy: this compound adopts a well-defined, rigid conformation in solution characterized by an antiparallel β-sheet structure stabilized by two disulfide bonds. [, ]
- Circular Dichroism (CD) Spectroscopy: CD spectra confirm the β-sheet conformation of this compound in aqueous solutions, with characteristic minima and maxima. [, , , ]
ANone: this compound exhibits considerable stability under various conditions:
- Temperature: It remains stable at temperatures below 100°C. []
- pH: It shows stability at pH values less than 10.3. []
- Cation Concentration: Cation concentrations can influence its antibacterial activity. []
- Solvent Polarity: Solvent polarity can also affect its antibacterial activity. []
ANone: this compound is not known to possess catalytic properties. Its primary mode of action is through direct interaction with bacterial membranes rather than enzymatic activity.
ANone: Yes, computational approaches have provided insights into this compound's interactions with membranes:
- Molecular Dynamics (MD) Simulations: MD simulations have been employed to investigate the orientation and conformational changes of this compound at model membrane interfaces, providing a detailed view of its membrane insertion mechanism. [, ]
- Quantitative Structure-Activity Relationship (QSAR) Studies: QSAR models have been developed to correlate the structural features of this compound and its analogs with their antimicrobial activity, aiding in the design of more potent and selective peptides. [, ]
ANone: The relationship between this compound's structure and its biological activity has been extensively investigated, revealing key features contributing to its efficacy and selectivity:
- Cyclic Structure: The two disulfide bonds are essential for maintaining its rigid β-hairpin conformation, which is crucial for its antimicrobial activity. Linear analogs with disrupted disulfide bonds generally exhibit reduced activity. [, , , ]
- Cationic Nature: The positively charged residues (lysine and arginine) contribute to its electrostatic interactions with negatively charged bacterial membranes. []
- Hydrophobicity: The hydrophobic residues (phenylalanine, valine, isoleucine) contribute to its ability to insert and disrupt lipid bilayers. [, ]
- β-Turn Region: The amino acid residues within the β-turn region influence its membrane interaction, hemolytic activity, and cell selectivity. Substitutions in this region, particularly with hydrophilic residues like serine, can reduce hemolytic activity while maintaining antimicrobial activity. []
ANone: While this compound demonstrates good stability under various conditions, its formulation for therapeutic use presents challenges:
- Proteolytic Degradation: Like many peptides, this compound is susceptible to degradation by proteases, which can limit its bioavailability and in vivo efficacy. []
- Formulation Strategies: Various strategies are being explored to improve its stability and bioavailability, including:
ANone: While research on this compound is ongoing, its safety profile, environmental impact, and responsible handling require thorough assessment:
- Toxicity: Although this compound exhibits low toxicity towards mammalian cells compared to bacteria, further studies are needed to comprehensively evaluate its safety profile, including potential long-term effects. [, ]
- Environmental Impact: Information regarding its environmental fate, degradation pathways, and potential ecotoxicological effects is limited. Strategies to mitigate potential negative impacts should be explored. []
A: Research on the pharmacokinetics and pharmacodynamics of this compound is currently limited. Further investigations are needed to understand its absorption, distribution, metabolism, excretion, and in vivo activity and efficacy. []
ANone: this compound has demonstrated promising antimicrobial activity in both in vitro and in vivo settings:
- In vitro studies: Numerous studies have established its potent activity against a broad range of Gram-negative and Gram-positive bacteria, including clinically relevant pathogens. [, , , , ]
- In vivo studies: this compound has shown efficacy in animal models of bacterial infection, exhibiting therapeutic potential for treating bacterial infections. []
A: While this compound has been touted as a promising candidate to combat antibiotic resistance, recent studies have shown that bacteria can develop resistance to this compound under selective pressure. [, ] The resistance mechanisms involve:
- Reduced outer membrane permeability: This is often caused by changes in the expression of outer membrane proteins (OMPs) or the overexpression of efflux pumps, preventing the peptide from reaching its target. [, ]
- Increased proteolytic degradation: Some bacteria might upregulate protease production, leading to the breakdown of this compound before it can exert its antimicrobial effect. []
A: Although this compound displays a relatively low toxicity profile towards mammalian cells compared to bacteria, further research is essential to fully elucidate its safety profile, particularly regarding potential long-term effects. [, ]
ANone: Research is ongoing to develop effective drug delivery systems that can enhance its stability, bioavailability, and target specificity:
- Nanoparticle encapsulation: Encapsulating this compound within nanoparticles offers protection from degradation, controlled release, and improved delivery to target tissues, potentially enhancing its therapeutic efficacy. []
A: Further research is needed to identify potential biomarkers that could predict the efficacy of this compound treatment, monitor treatment response, or serve as indicators of potential adverse effects. []
ANone: Various analytical techniques are employed to characterize and quantify this compound:
- High-Performance Liquid Chromatography (HPLC): HPLC is widely used to purify and analyze this compound, separating it from other components in a mixture. [, ]
- NMR Spectroscopy: NMR provides detailed structural information about this compound's conformation and dynamics in solution. [, ]
- CD Spectroscopy: CD spectroscopy is used to study its secondary structure, particularly its β-sheet conformation. [, , , ]
Descargo de responsabilidad e información sobre productos de investigación in vitro
Tenga en cuenta que todos los artículos e información de productos presentados en BenchChem están destinados únicamente con fines informativos. Los productos disponibles para la compra en BenchChem están diseñados específicamente para estudios in vitro, que se realizan fuera de organismos vivos. Los estudios in vitro, derivados del término latino "in vidrio", involucran experimentos realizados en entornos de laboratorio controlados utilizando células o tejidos. Es importante tener en cuenta que estos productos no se clasifican como medicamentos y no han recibido la aprobación de la FDA para la prevención, tratamiento o cura de ninguna condición médica, dolencia o enfermedad. Debemos enfatizar que cualquier forma de introducción corporal de estos productos en humanos o animales está estrictamente prohibida por ley. Es esencial adherirse a estas pautas para garantizar el cumplimiento de los estándares legales y éticos en la investigación y experimentación.