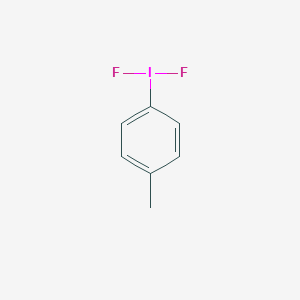
Difluoroiodotolueno
- Haga clic en CONSULTA RÁPIDA para recibir una cotización de nuestro equipo de expertos.
- Con productos de calidad a un precio COMPETITIVO, puede centrarse más en su investigación.
Descripción general
Descripción
Difluoroiodotoluene, also known as Difluoroiodotoluene, is a useful research compound. Its molecular formula is C7H7F2I-2 and its molecular weight is 256.03 g/mol. The purity is usually 95%.
The exact mass of the compound Difluoroiodotoluene is unknown and the complexity rating of the compound is unknown. The United Nations designated GHS hazard class pictogram is Corrosive;Irritant, and the GHS signal word is DangerThe storage condition is unknown. Please store according to label instructions upon receipt of goods.
BenchChem offers high-quality Difluoroiodotoluene suitable for many research applications. Different packaging options are available to accommodate customers' requirements. Please inquire for more information about Difluoroiodotoluene including the price, delivery time, and more detailed information at info@benchchem.com.
Aplicaciones Científicas De Investigación
Fluorociclación de ácidos carboxílicos o alcoholes insaturados
Difluoroiodotolueno se ha utilizado en la fluorociclación de ácidos carboxílicos o alcoholes insaturados . Este proceso implica el uso de yodo (III) hipervalente y depende del mecanismo de la reacción. La selectividad entre la ciclación 6-endo y 5-exo está determinada por el grupo funcional del sustrato .
Fluoración de compuestos que contienen azufre
This compound se ha utilizado en la fluoración de compuestos que contienen azufre . Este proceso implica la reacción de Fluoro-Pummerer, que introduce flúor en la posición α de los sulfoxidos o sulfuros a través de intermedios de iones sulfonio .
Síntesis de sulfuros α-fluoro
El tratamiento de sulfuros β-oxo con un equivalente de this compound en solución de diclorometano puede conducir a la formación de sulfuros α-fluoro . Esta reacción es promovida por grupos que atraen electrones en la posición α .
Síntesis de sulfoxidos α-fluoro
This compound se puede utilizar para producir sulfoxidos α-fluoro. Esto se logra tratando los sustratos con exceso de reactivo .
Síntesis de sulfuros de vinilo
En los casos en que los sustratos tienen hidrógenos β, puede ocurrir una reacción de eliminación, produciendo sulfuros de vinilo . Estos sulfuros de vinilo pueden luego agregar dos equivalentes de fluoruro a través de una reacción de adición-Pummerer para producir α, β-difluorosulfuros .
Síntesis de compuestos organofluorados
Debido a sus propiedades físicas y químicas únicas, los compuestos organofluorados se aplican ampliamente en productos farmacéuticos, agroquímicos, materiales funcionales y muchas otras áreas
Mecanismo De Acción
Target of Action
Difluoroiodotoluene, also known as p-(Difluoroiodo)toluene, primarily targets sulfur-containing compounds . It is used in the fluorination of these compounds, introducing fluorine into the α-position of sulfoxides or sulfides via sulfonium ion intermediates . This process is known as the Fluoro-Pummerer reaction .
Mode of Action
Difluoroiodotoluene interacts with its targets through a process known as the Fluoro-Pummerer reaction . This reaction involves the introduction of fluorine into the α-position of sulfoxides or sulfides via sulfonium ion intermediates . The reaction is promoted by electron-withdrawing groups in the α-position . In cases where substrates have β-hydrogens, an elimination reaction operates, producing vinyl sulfides . These vinyl sulfides can then add two equivalents of fluoride via an additive-Pummerer reaction to produce α, β-difluorosulfides .
Biochemical Pathways
The primary biochemical pathway affected by Difluoroiodotoluene is the fluorination of sulfur-containing compounds . This process leads to the formation of a range of novel 3,4-difluoro pyrrolidinones and piperidinones .
Pharmacokinetics
The strength of the carbon-fluorine bond generally conveys stability to fluorinated drugs, making them likely to be recalcitrant in the environment . This could potentially impact the bioavailability of Difluoroiodotoluene, but more research is needed to confirm this.
Result of Action
The result of Difluoroiodotoluene’s action is the formation of a range of novel 3,4-difluoro pyrrolidinones and piperidinones . These compounds are formed through the Fluoro-Pummerer reaction and subsequent reactions
Action Environment
The action of Difluoroiodotoluene can be influenced by environmental factors. For example, the presence of electron-withdrawing groups in the α-position promotes the Fluoro-Pummerer reaction . Additionally, the presence of β-hydrogens in the substrate can lead to an elimination reaction . .
Actividad Biológica
Difluoroiodotoluene is a compound that has garnered attention in the field of synthetic chemistry, particularly for its role as a reagent in various fluorination reactions. Its biological activity stems from its unique chemical properties, which enable it to participate in diverse reaction mechanisms. This article explores the biological activity of difluoroiodotoluene, focusing on its mechanisms of action, applications in medicinal chemistry, and relevant research findings.
Difluoroiodotoluene acts primarily as a hypervalent iodine reagent, facilitating fluorocyclization reactions. The mechanism of action can be categorized into two main pathways:
- Fluorination First, Cyclization Later :
-
Cyclization First, Fluorination Later :
- In contrast, when reacting with unsaturated carboxylic acids, difluoroiodotoluene typically undergoes cyclization before fluorination. This results in the formation of 5-exo lactones. The transition states involved in these reactions demonstrate significant differences in energy barriers, which dictate the favored pathway .
Research Findings
Recent studies have provided insights into the biological implications and synthetic utility of difluoroiodotoluene:
- A study by Sawaguchi et al. (2000) demonstrated that difluoroiodotoluene could effectively introduce fluorine into various substrates, enhancing their biological activity by modifying their lipophilicity and permeability .
- Density Functional Theory (DFT) calculations have been employed to elucidate the reaction mechanisms and predict product selectivity based on substrate characteristics. These computational studies indicate that the reaction outcomes are highly dependent on the nature of the substrate and the specific reaction conditions used .
Applications in Medicinal Chemistry
The ability to introduce fluorine atoms into organic molecules is crucial for drug design and development. Fluorinated compounds often exhibit improved pharmacokinetic properties, making difluoroiodotoluene a valuable tool in medicinal chemistry:
- Antimicrobial Agents : Fluorinated derivatives synthesized using difluoroiodotoluene have shown enhanced antimicrobial activity compared to their non-fluorinated counterparts.
- Anticancer Compounds : Research indicates that fluorinated compounds can exhibit selective cytotoxicity against cancer cells, highlighting the potential of difluoroiodotoluene in developing new cancer therapies .
Case Studies
Several case studies illustrate the effectiveness of difluoroiodotoluene in synthesizing biologically active compounds:
- Synthesis of Antiviral Agents : In one study, difluoroiodotoluene was used to synthesize a series of antiviral agents that demonstrated significant activity against viral infections. The introduction of fluorine atoms was shown to enhance binding affinity to viral targets.
- Development of Fluorinated Antibiotics : Another case involved the modification of existing antibiotics with fluorine using difluoroiodotoluene, resulting in compounds with improved efficacy against resistant bacterial strains.
Propiedades
Número CAS |
371-11-9 |
---|---|
Fórmula molecular |
C7H7F2I-2 |
Peso molecular |
256.03 g/mol |
Nombre IUPAC |
1-iodo-4-methylbenzene;difluoride |
InChI |
InChI=1S/C7H7I.2FH/c1-6-2-4-7(8)5-3-6;;/h2-5H,1H3;2*1H/p-2 |
Clave InChI |
PRWFBLUVLWFEMJ-UHFFFAOYSA-L |
SMILES |
CC1=CC=C(C=C1)I(F)F |
SMILES canónico |
CC1=CC=C(C=C1)I.[F-].[F-] |
Pictogramas |
Corrosive; Irritant |
Sinónimos |
P-(DIFLUOROIODO)TOLUENE; 4-IodotolueneDifluoride(Tol-If2); tol-IF2; 4-IODOTOLUENE DIFLUORIDE (TOL-IF2) ,98%MIN; 4-Iodotoluene difluoride; p-Tolyliododifluoride |
Origen del producto |
United States |
Descargo de responsabilidad e información sobre productos de investigación in vitro
Tenga en cuenta que todos los artículos e información de productos presentados en BenchChem están destinados únicamente con fines informativos. Los productos disponibles para la compra en BenchChem están diseñados específicamente para estudios in vitro, que se realizan fuera de organismos vivos. Los estudios in vitro, derivados del término latino "in vidrio", involucran experimentos realizados en entornos de laboratorio controlados utilizando células o tejidos. Es importante tener en cuenta que estos productos no se clasifican como medicamentos y no han recibido la aprobación de la FDA para la prevención, tratamiento o cura de ninguna condición médica, dolencia o enfermedad. Debemos enfatizar que cualquier forma de introducción corporal de estos productos en humanos o animales está estrictamente prohibida por ley. Es esencial adherirse a estas pautas para garantizar el cumplimiento de los estándares legales y éticos en la investigación y experimentación.