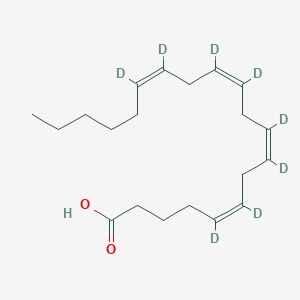
(5Z,8Z,11Z,14Z)-5,6,8,9,11,12,14,15-octadeuterioicosa-5,8,11,14-tetraenoic acid
Descripción general
Descripción
“(5Z,8Z,11Z,14Z)-5,6,8,9,11,12,14,15-octadeuterioicosa-5,8,11,14-tetraenoic acid” is a derivative of arachidonic acid . Arachidonic acid is an omega-6 unsaturated fatty acid that is a constituent of cellular membranes, esterified to the sn-2 position of glycerophospholipids . It plays important roles in a variety of biological processes, including signal transduction, contraction, chemotaxis, cell proliferation and differentiation, and apoptosis .
Synthesis Analysis
The synthesis of similar compounds has been reported in the literature. For instance, the total synthesis of (5Z, 8Z, 11Z, 14Z)-18- and 19-oxoeicosa-5,8,11,14-tetraenoic acids was reported by Stepan G. Romanov et al . Another study reported the synthesis of methyl (5Z,8Z,11Z,14Z,17Z)‐ and (5Z,8Z,11Z,14Z,17E)‐ [18‐14C] eicosapentaenoate .Molecular Structure Analysis
The molecular structure of this compound is similar to that of arachidonic acid, with the difference being the presence of deuterium atoms . The molecular formula of arachidonic acid is C20H32O2 .Chemical Reactions Analysis
Arachidonic acid, from which this compound is derived, is involved in various chemical reactions. It is released from membrane phospholipids by the activation of phospholipases and is available for oxidative metabolism by several enzyme systems including cyclooxygenases, lipoxygenases, and cytochrome P450 enzymes .Physical And Chemical Properties Analysis
The physical and chemical properties of this compound would be similar to those of arachidonic acid. The molecular weight of arachidonic acid is 304.47 g/mol, and it appears as an oil .Aplicaciones Científicas De Investigación
Internal Standard for Quantification
Arachidonic Acid-d8 is used as an internal standard for the quantification of arachidonic acid by GC- or LC-mass spectrometry . This allows for accurate and precise measurements of arachidonic acid in various samples.
Production by Fermentation and Genetic Modification
Arachidonic Acid-d8 can be produced by fermentation and genetic modification of Mortierella alpina . This method provides a sustainable alternative to obtaining arachidonic acid from animal tissues.
Medicine
Arachidonic Acid-d8 plays essential roles in human immune, cardiovascular, and nervous systems . It has been widely used in medicine, contributing to the development of treatments for various diseases.
Cosmetics
Due to its beneficial effects on the skin, Arachidonic Acid-d8 is used in the cosmetics industry . It can help improve skin health and appearance.
Nutrition
Arachidonic Acid-d8 is an essential fatty acid and is used in nutrition . It is often added to infant and elderly formulas to ensure they receive this important nutrient.
Precursor for Prostaglandins, Thromboxanes, and Leukotrienes
Arachidonic Acid-d8 is a precursor for all prostaglandins, thromboxanes, and leukotrienes . These compounds play important roles in various biological processes, including inflammation and blood clotting.
Research on ω-6 Polyunsaturated Fatty Acids
Arachidonic Acid-d8 is a relevant ω-6 polyunsaturated fatty acid . It is used in research to understand the role of these fatty acids in human health.
Synthetic Biology
Arachidonic Acid-d8 is used in synthetic biology methods and technologies to increase ARA production . This can help meet the growing demand for this essential fatty acid.
Mecanismo De Acción
Arachidonic Acid-d8, also known as (5Z,8Z,11Z,14Z)-5,6,8,9,11,12,14,15-octadeuterioicosa-5,8,11,14-tetraenoic acid, is a deuterium-labeled form of Arachidonic Acid . It plays a crucial role in various biological processes, including inflammation and cell signaling .
Target of Action
Arachidonic Acid-d8 primarily targets the Arachidonic Acid (AA) cascade in the brain . This cascade is selectively targeted by mood stabilizers such as lithium, valproate, and carbamazepine . The AA cascade is also a common target for drugs or diet therapies in bipolar disorder .
Mode of Action
Arachidonic Acid-d8 interacts with its targets through a series of biochemical reactions. Chronic administration of mood stabilizers decreases the binding activity of activator protein-2, which in turn reduces the transcription, translation, and activity of its AA-selective calcium-dependent phospholipase A2 gene product . Valproate is a non-competitive inhibitor of long-chain acyl-CoA synthetase .
Biochemical Pathways
Arachidonic Acid-d8 affects several biochemical pathways. It is a precursor for all prostaglandins, thromboxanes, and leukotrienes . Virtually all cellular Arachidonic Acid is esterified in membrane phospholipids . The AA cascade is a common pathway affected by Arachidonic Acid-d8 .
Pharmacokinetics
Arachidonic Acid-d8 is hydrolyzed by a direct action of phospholipase A2 (PLA2) or through the combined action of phospholipase C and diacylglycerol lipase, and released into the cytoplasm . The cyclooxygenase reaction provokes a bisoxygenation of arachidonic acid to generate prostaglandin G2 (PGG2), which is then transformed into PGH2 through a peroxidase reaction .
Result of Action
Arachidonic Acid-d8 contributes to the development of inflammation as intercellular pro-inflammatory mediators . It also promotes the excitability of the peripheral somatosensory system, contributing to pain exacerbation . Arachidonic Acid-d8 for signaling purposes is derived by the action of group IVA cytosolic phospholipase A2 (cPLA2, 85 kDa), whereas inflammatory Arachidonic Acid-d8 is generated by the action of a low-molecular-weight secretory PLA2 (sPLA2, 14-18 kDa) .
Propiedades
IUPAC Name |
(5Z,8Z,11Z,14Z)-5,6,8,9,11,12,14,15-octadeuterioicosa-5,8,11,14-tetraenoic acid | |
---|---|---|
Source | PubChem | |
URL | https://pubchem.ncbi.nlm.nih.gov | |
Description | Data deposited in or computed by PubChem | |
InChI |
InChI=1S/C20H32O2/c1-2-3-4-5-6-7-8-9-10-11-12-13-14-15-16-17-18-19-20(21)22/h6-7,9-10,12-13,15-16H,2-5,8,11,14,17-19H2,1H3,(H,21,22)/b7-6-,10-9-,13-12-,16-15-/i6D,7D,9D,10D,12D,13D,15D,16D | |
Source | PubChem | |
URL | https://pubchem.ncbi.nlm.nih.gov | |
Description | Data deposited in or computed by PubChem | |
InChI Key |
YZXBAPSDXZZRGB-FBFLGLDDSA-N | |
Source | PubChem | |
URL | https://pubchem.ncbi.nlm.nih.gov | |
Description | Data deposited in or computed by PubChem | |
Canonical SMILES |
CCCCCC=CCC=CCC=CCC=CCCCC(=O)O | |
Source | PubChem | |
URL | https://pubchem.ncbi.nlm.nih.gov | |
Description | Data deposited in or computed by PubChem | |
Isomeric SMILES |
[2H]/C(=C(\[2H])/C/C(=C(/[2H])\C/C(=C(/[2H])\C/C(=C(/[2H])\CCCC(=O)O)/[2H])/[2H])/[2H])/CCCCC | |
Source | PubChem | |
URL | https://pubchem.ncbi.nlm.nih.gov | |
Description | Data deposited in or computed by PubChem | |
Molecular Formula |
C20H32O2 | |
Source | PubChem | |
URL | https://pubchem.ncbi.nlm.nih.gov | |
Description | Data deposited in or computed by PubChem | |
Molecular Weight |
312.5 g/mol | |
Source | PubChem | |
URL | https://pubchem.ncbi.nlm.nih.gov | |
Description | Data deposited in or computed by PubChem | |
Product Name |
Arachidonic Acid-d8 |
Retrosynthesis Analysis
AI-Powered Synthesis Planning: Our tool employs the Template_relevance Pistachio, Template_relevance Bkms_metabolic, Template_relevance Pistachio_ringbreaker, Template_relevance Reaxys, Template_relevance Reaxys_biocatalysis model, leveraging a vast database of chemical reactions to predict feasible synthetic routes.
One-Step Synthesis Focus: Specifically designed for one-step synthesis, it provides concise and direct routes for your target compounds, streamlining the synthesis process.
Accurate Predictions: Utilizing the extensive PISTACHIO, BKMS_METABOLIC, PISTACHIO_RINGBREAKER, REAXYS, REAXYS_BIOCATALYSIS database, our tool offers high-accuracy predictions, reflecting the latest in chemical research and data.
Strategy Settings
Precursor scoring | Relevance Heuristic |
---|---|
Min. plausibility | 0.01 |
Model | Template_relevance |
Template Set | Pistachio/Bkms_metabolic/Pistachio_ringbreaker/Reaxys/Reaxys_biocatalysis |
Top-N result to add to graph | 6 |
Feasible Synthetic Routes
Descargo de responsabilidad e información sobre productos de investigación in vitro
Tenga en cuenta que todos los artículos e información de productos presentados en BenchChem están destinados únicamente con fines informativos. Los productos disponibles para la compra en BenchChem están diseñados específicamente para estudios in vitro, que se realizan fuera de organismos vivos. Los estudios in vitro, derivados del término latino "in vidrio", involucran experimentos realizados en entornos de laboratorio controlados utilizando células o tejidos. Es importante tener en cuenta que estos productos no se clasifican como medicamentos y no han recibido la aprobación de la FDA para la prevención, tratamiento o cura de ninguna condición médica, dolencia o enfermedad. Debemos enfatizar que cualquier forma de introducción corporal de estos productos en humanos o animales está estrictamente prohibida por ley. Es esencial adherirse a estas pautas para garantizar el cumplimiento de los estándares legales y éticos en la investigación y experimentación.