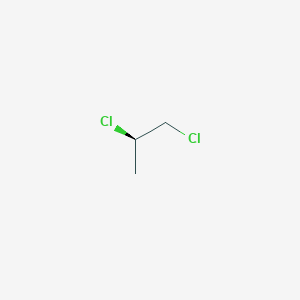
1,2-Dicloropropano
Descripción general
Descripción
Es un líquido incoloro, inflamable con un olor dulce, y su fórmula química es C3H6Cl2 . Este compuesto se utiliza principalmente como intermediario en la producción de otros productos químicos y tiene diversas aplicaciones industriales.
Aplicaciones Científicas De Investigación
El 1,2-Dicloro-Propano tiene varias aplicaciones de investigación científica:
Química: Se utiliza como disolvente y como intermediario en la síntesis de otros productos químicos.
Biología: Se utiliza en estudios relacionados con sus efectos toxicológicos y su potencial como carcinógeno.
Medicina: Se realiza investigación para comprender sus efectos en la salud humana y sus posibles aplicaciones terapéuticas.
Industria: Se utiliza en la producción de percloroetileno y otros productos químicos clorados.
Mecanismo De Acción
El mecanismo de acción del 1,2-Dicloro-Propano implica su interacción con los componentes celulares. Se metaboliza por la enzima haloalcano deshalogenasa, que cataliza la escisión hidrolítica de los enlaces carbono-halógeno, lo que lleva a la formación de alcoholes primarios, iones haluro y protones . Este proceso puede provocar daño celular y se ha relacionado con sus efectos cancerígenos.
Análisis Bioquímico
Biochemical Properties
1,2-Dichloropropane is readily absorbed following inhalation and oral exposure . The predominant pathway for 1,2-dichloropropane metabolism consists of oxidation of the parent compound followed by glutathione conjugation resulting in formation of mercapturic acids .
Cellular Effects
Exposure to 1,2-Dichloropropane upregulates the expression of Activation-Induced Cytidine Deaminase (AID) in human cholangiocytes co-cultured with macrophages . This suggests that inflammatory response of macrophages and consequent aberrant AID expression or DNA damage in the cholangiocytes underlie the mechanism of 1,2-Dichloropropane-induced cholangiocarcinoma in humans .
Molecular Mechanism
The molecular mechanism of 1,2-Dichloropropane involves its metabolism through oxidation followed by glutathione conjugation . This process results in the formation of mercapturic acids .
Temporal Effects in Laboratory Settings
It is known that 1,2-Dichloropropane is rapidly and extensively absorbed following inhalation and oral exposure .
Dosage Effects in Animal Models
In animal experiments, low amounts of 1,2-Dichloropropane breathed in over short- and long-term periods result in damage to the liver, kidney, and respiratory systems, while high amounts resulted in death .
Metabolic Pathways
The predominant pathway for 1,2-Dichloropropane metabolism consists of oxidation of the parent compound followed by glutathione conjugation . This process results in the formation of mercapturic acids .
Transport and Distribution
1,2-Dichloropropane is widely distributed; highest levels were found in the liver, kidney, and blood; high levels were also observed in the lung following inhalation exposure .
Métodos De Preparación
El 1,2-Dicloro-Propano se produce típicamente como subproducto en la producción de epiclorhidrina . La producción industrial implica la cloración de propeno, lo que da como resultado una mezcla de propanos clorados, incluido el 1,2-Dicloro-Propano . Las condiciones de reacción suelen implicar el uso de gas cloro y un catalizador a temperaturas elevadas.
Análisis De Reacciones Químicas
El 1,2-Dicloro-Propano experimenta varios tipos de reacciones químicas, incluidas:
Reacciones de Sustitución: Puede reaccionar con nucleófilos, como los iones hidróxido, para formar propilenglicol.
Reacciones de Oxidación: Se puede oxidar para formar varios propanoles y propanonas clorados.
Reacciones de Reducción: Se puede reducir para formar propeno y cloruro de hidrógeno.
Los reactivos comunes utilizados en estas reacciones incluyen oxidantes fuertes, ácidos fuertes y bases . Los principales productos formados dependen de las condiciones de reacción y los reactivos específicos utilizados.
Comparación Con Compuestos Similares
El 1,2-Dicloro-Propano se puede comparar con otros compuestos similares, como:
- 1,1-Dicloropropano
- 1,3-Dicloropropano
- 2,2-Dicloropropano
Estos compuestos comparten estructuras químicas similares, pero difieren en sus propiedades físicas y químicas, así como en sus aplicaciones. El 1,2-Dicloro-Propano es único debido a sus aplicaciones industriales específicas y su papel como intermediario en la producción de otros productos químicos.
Actividad Biológica
1,2-Dichloropropane (DCP) is a chlorinated hydrocarbon that has been utilized in various industrial applications, including as a solvent and in the production of other chemicals. Understanding its biological activity is crucial due to its potential health effects in humans and animals. This article synthesizes findings from various studies, focusing on the compound's toxicity, metabolic pathways, and associated health risks.
1,2-Dichloropropane is a colorless liquid with a sweet odor, primarily used as a solvent and in organic synthesis. Upon exposure, DCP undergoes metabolic transformation primarily in the liver. Studies indicate that after oral administration, approximately 50% of DCP is excreted in urine within 24 hours, with metabolites such as N-acetyl-S-(2-hydroxypropyl)-l-cysteine being predominant .
Metabolic Pathways
- Major Metabolites :
- N-acetyl-S-(2-hydroxypropyl)-l-cysteine
- β-chlorolactate
- Excretion :
Hepatic Effects
Numerous studies have documented the hepatotoxicity of DCP. Acute exposure can lead to:
Table 1: Summary of Hepatic Effects from Animal Studies
Exposure Route | Concentration | Observed Effect |
---|---|---|
Inhalation | ≥1000 ppm | Hepatic necrosis |
Oral | ≥500 mg/kg | Centrilobular hepatocyte changes |
Oral | ≥125 mg/kg/day | Delayed skull ossification in fetuses |
Neurological Effects
DCP is known to cause central nervous system (CNS) depression. Symptoms observed include:
Renal Effects
While the kidney is not typically considered a primary target for DCP toxicity, some studies have reported renal impairment at high doses:
- Acute kidney failure has been noted in human case reports following high-level exposure.
- Laboratory studies show inconsistent renal damage, primarily at concentrations above 1000 ppm .
Developmental Toxicity
Developmental studies indicate that DCP can adversely affect fetal development:
- Delayed ossification observed in rat and rabbit fetuses at doses ≥125 mg/kg/day.
- Increased mortality rates were noted in offspring exposed to high maternal doses during gestation .
Case Studies and Epidemiological Evidence
Several case studies have linked high levels of DCP exposure to serious health outcomes:
Propiedades
Número CAS |
78-87-5 |
---|---|
Fórmula molecular |
C3H6Cl2 |
Peso molecular |
112.98 g/mol |
Nombre IUPAC |
(2R)-1,2-dichloropropane |
InChI |
InChI=1S/C3H6Cl2/c1-3(5)2-4/h3H,2H2,1H3/t3-/m1/s1 |
Clave InChI |
KNKRKFALVUDBJE-GSVOUGTGSA-N |
Impurezas |
WATER-O.O5% MAX, OXYGEN COMPOUNDS-0.1% MAX |
SMILES |
CC(CCl)Cl |
SMILES isomérico |
C[C@H](CCl)Cl |
SMILES canónico |
CC(CCl)Cl |
Punto de ebullición |
203 to 205 °F at 760 mm Hg (NTP, 1992) 95.5 °C 96.4 °C; (-3.7 °C at 10 mm Hg) 96 °C 206°F |
Color/Form |
Colorless liquid |
Densidad |
1.158 at 68 °F (USCG, 1999) 1.159 @ 25 °C/25 °C Relative density (water = 1): 1.16 1.16 |
Punto de inflamación |
60 °F (NTP, 1992) 60 °F (16 °C) (Closed cup) 16 °C c.c. 60°F |
melting_point |
-148 °F (NTP, 1992) -100.0 °C -100.4 °C -100 °C 149°F -149°F |
Key on ui other cas no. |
78-87-5 68390-96-5 78-87-5; 26638-19-7(mixed isomers) |
Descripción física |
1,2-dichloropropane is a colorless watery liquid with a sweet odor. Sinks in water. Produces an irritating vapor. (USCG, 1999) Liquid COLOURLESS LIQUID WITH CHARACTERISTIC ODOUR. Colorless liquid with a chloroform-like odor. Colorless liquid with a chloroform-like odor. [pesticide] |
Pictogramas |
Flammable; Irritant; Health Hazard |
Vida útil |
Sensitive to heat. |
Solubilidad |
less than 0.1 mg/mL at 70.7° F (NTP, 1992) 0.02 M Sol in alcohol, ether, benzene and chloroform Miscible with org solvents In water, 2,800 mg/l @ 25 °C Solubility in water, g/100ml at 20 °C: 0.26 0.3% |
Sinónimos |
1,2-DCP; 1,2-Dichloropropane; NSC 1237; Propylene Chloride; Propylene Dichloride; R 270da |
Densidad de vapor |
3.9 (NTP, 1992) (Relative to Air) 3.9 (AIR= 1) Relative vapor density (air = 1): 3.9 3.9 |
Presión de vapor |
40 mm Hg at 66.9 °F ; 42 mm Hg at 68° F (NTP, 1992) 53.30 mmHg 53.3 mm Hg @ 25 °C Vapor pressure, kPa at 20 °C: 27.9 40 mmHg |
Origen del producto |
United States |
Synthesis routes and methods
Procedure details
Retrosynthesis Analysis
AI-Powered Synthesis Planning: Our tool employs the Template_relevance Pistachio, Template_relevance Bkms_metabolic, Template_relevance Pistachio_ringbreaker, Template_relevance Reaxys, Template_relevance Reaxys_biocatalysis model, leveraging a vast database of chemical reactions to predict feasible synthetic routes.
One-Step Synthesis Focus: Specifically designed for one-step synthesis, it provides concise and direct routes for your target compounds, streamlining the synthesis process.
Accurate Predictions: Utilizing the extensive PISTACHIO, BKMS_METABOLIC, PISTACHIO_RINGBREAKER, REAXYS, REAXYS_BIOCATALYSIS database, our tool offers high-accuracy predictions, reflecting the latest in chemical research and data.
Strategy Settings
Precursor scoring | Relevance Heuristic |
---|---|
Min. plausibility | 0.01 |
Model | Template_relevance |
Template Set | Pistachio/Bkms_metabolic/Pistachio_ringbreaker/Reaxys/Reaxys_biocatalysis |
Top-N result to add to graph | 6 |
Feasible Synthetic Routes
Q1: What are the major health concerns associated with 1,2-dichloropropane (1,2-DCP) exposure?
A1: 1,2-DCP is primarily known for its hepatotoxicity, causing liver damage in both animal models and humans. [, , , ] Additionally, it has been linked to renal toxicity, affecting kidney function. [] Alarmingly, epidemiological studies have shown a strong correlation between occupational exposure to 1,2-DCP and the development of cholangiocarcinoma, a rare and aggressive form of bile duct cancer. [, , , ]
Q2: How does 1,2-DCP exert its toxic effects on the liver?
A2: While the exact mechanisms are still under investigation, research suggests that 1,2-DCP toxicity stems from its metabolism within the liver. [] This process likely involves conjugation with glutathione, leading to the depletion of this vital antioxidant and rendering the liver more susceptible to oxidative damage. []
Q3: What are the long-term effects of 1,2-DCP exposure?
A3: Long-term exposure to 1,2-DCP, even at low levels, has been shown to increase the risk of developing cancer, particularly cholangiocarcinoma, in both male and female mice. [, ] This carcinogenic potential is further supported by observations in human studies, particularly among workers in the printing industry. [, , ]
Q4: How does dietary intake affect the toxicity of 1,2-DCP?
A4: Studies in rats have shown that a low-protein, choline-deficient diet increases susceptibility to the toxic effects of 1,2-DCP inhalation compared to a control diet. [] Interestingly, supplementation with methionine or a combination of cystine and choline provided a protective effect. [] This highlights the importance of nutritional status in modulating the toxicity of this compound.
Q5: How is 1,2-DCP metabolized in the rat?
A5: The primary metabolic pathway of 1,2-DCP in rats involves conjugation with glutathione, leading to the formation of N-acetyl-S-(2-hydroxypropyl)cysteine as the major urinary metabolite. [] Minor metabolites include β-chlorolactate and N-acetyl-S-(2,3-dihydroxypropyl)cysteine. []
Q6: What is the fate of 1-chloro-2-hydroxypropane, a proposed intermediate in 1,2-DCP metabolism?
A6: Besides forming the known metabolite N-acetyl-S-(2-hydroxypropyl)cysteine, 1-chloro-2-hydroxypropane also undergoes oxidation to yield β-chlorolactaldehyde and β-chlorolactate as metabolites. []
Q7: What are the main environmental concerns related to 1,2-DCP?
A7: 1,2-DCP is a known groundwater contaminant. [, , , ] Its persistence in the environment poses risks to both human and ecological health. [] Bioremediation strategies are crucial for removing this pollutant from contaminated sites.
Q8: How can microbial communities contribute to the bioremediation of 1,2-DCP?
A8: Anaerobic bacteria, particularly species belonging to the genus Dehalococcoides, are capable of completely degrading 1,2-DCP to the environmentally benign product propene. [, , , , ] This process, known as reductive dechlorination, relies on 1,2-DCP as an electron acceptor and is supported by electron donors like hydrogen. []
Q9: What factors influence the effectiveness of bioremediation of 1,2-DCP?
A9: Several factors can affect the efficacy of 1,2-DCP bioremediation, including:
- Microbial community composition: The presence and abundance of specific dechlorinating bacteria, like Dehalococcoides, are crucial. []
- Environmental conditions: Temperature, pH, and the availability of electron donors like hydrogen influence dechlorination rates. []
- Contaminant concentration: While Dehalococcoides spp. can tolerate and degrade high concentrations of 1,2-DCP, extremely high levels might still inhibit their activity. []
Q10: How can stable carbon isotope analysis be used to monitor 1,2-DCP degradation?
A10: Stable carbon isotope analysis (CSIA) is a powerful tool for tracking the fate of 1,2-DCP in the environment. [] By measuring the isotopic fractionation of carbon during dichloroelimination, researchers can distinguish between biotic and abiotic degradation pathways, providing valuable insights into the effectiveness of bioremediation efforts. []
Q11: What is the molecular formula and weight of 1,2-DCP?
A11: The molecular formula of 1,2-DCP is C3H6Cl2, and its molecular weight is 112.99 g/mol.
Q12: What are the typical products of 1,2-DCP pyrolysis?
A12: The pyrolysis of 1,2-DCP primarily yields hydrogen chloride (HCl) and monochloropropene isomers (allyl chloride, cis-1-chloropropene, and trans-1-chloropropene). [] The relative amounts of these isomers depend on the temperature at which the pyrolysis is carried out. []
Q13: Can the pyrolysis of 1,2-DCP be influenced by other compounds?
A13: Yes, the co-pyrolysis of 1,2-DCP with methanol in the presence of activated alumina catalyst leads to the formation of oxygen-containing compounds such as acetone and acrolein, in addition to the typical pyrolysis products. []
Q14: What analytical techniques are commonly used to detect and quantify 1,2-DCP?
A14: Gas chromatography, often coupled with electron capture detection (GC-ECD) or mass spectrometry (GC-MS), is a widely employed technique for the analysis of 1,2-DCP in various matrices, including environmental samples and biological tissues. [, , , , ]
Q15: What factors influence the efficiency of 1,2-DCP adsorption onto activated carbon?
A15: The adsorption of 1,2-DCP from aqueous solutions onto activated carbon is affected by factors such as initial concentration of the contaminant, adsorbent dosage, temperature, particle diameter of the activated carbon, and the rate of stirring during the adsorption process. [, ]
Descargo de responsabilidad e información sobre productos de investigación in vitro
Tenga en cuenta que todos los artículos e información de productos presentados en BenchChem están destinados únicamente con fines informativos. Los productos disponibles para la compra en BenchChem están diseñados específicamente para estudios in vitro, que se realizan fuera de organismos vivos. Los estudios in vitro, derivados del término latino "in vidrio", involucran experimentos realizados en entornos de laboratorio controlados utilizando células o tejidos. Es importante tener en cuenta que estos productos no se clasifican como medicamentos y no han recibido la aprobación de la FDA para la prevención, tratamiento o cura de ninguna condición médica, dolencia o enfermedad. Debemos enfatizar que cualquier forma de introducción corporal de estos productos en humanos o animales está estrictamente prohibida por ley. Es esencial adherirse a estas pautas para garantizar el cumplimiento de los estándares legales y éticos en la investigación y experimentación.