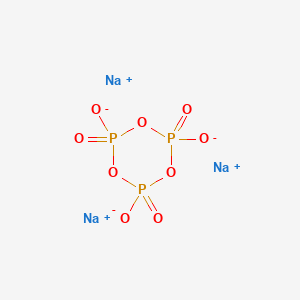
Sodium trimetaphosphate
Descripción general
Descripción
Sodium trimetaphosphate, also known as STMP, is a metaphosphate of sodium with the formula Na3P3O9 . It is a colorless solid that finds specialized applications in food and construction industries . It is also known as the sodium salt of trimetaphosphoric acid .
Synthesis Analysis
Sodium trimetaphosphate can be synthesized from sodium chloride and 85 wt % orthophosphoric acid as starting materials . This one-step thermal synthesis method involves heating the starting materials to produce sodium trimetaphosphate .Molecular Structure Analysis
Sodium trimetaphosphate has a molecular formula of Na3P3O9 . Its molecular weight is 305.89 g/mol . The structure of sodium trimetaphosphate is a cyclic phosphate with an O:P ratio of 3 .Physical And Chemical Properties Analysis
Sodium trimetaphosphate appears as colorless or white crystals . It has a density of 2.49 g/cm^3 (anhydrous) and 1.786 g/cm^3 (hexahydrate) . It is soluble in water (22 g/100 mL) but insoluble in alcohol . The hexahydrate form of sodium trimetaphosphate has a melting point of 53 °C (127 °F; 326 K) and decomposes to anhydrous .Aplicaciones Científicas De Investigación
Food Packaging
STMP is used as a crosslinking agent to prepare chitosan/methylcellulose composite films. The crosslinked films, with good antibacterial activity (~99%), had increased tensile strength, a higher elongation at break, a lower swelling ratio and solubility, and a lower enzymatic degradation than the non-crosslinked films . The films crosslinked by STMP can be potentially applied to the food industry, such as food functional packaging .
Conductive Hydrogels
STMP has been used in the development of interpenetrating PEDOT:PSS-based conductive hydrogels. The addition of STMP allowed sufficient physical crosslinking with PEDOT:PSS, resulting in improved hydrogel conductivity, wettability, and rheological properties . These hydrogels exhibit shear-thinning behaviors, which are potentially favorable for extrusion-based 3D bioprinting applications .
Water Treatment
STMP could be used in water treatment applications . It is known to be a good dispersant for kaolin suspensions, which are important raw materials in advanced ceramic processing .
Metal Surface Treatment
STMP finds applications in metal surface treatment . It has strong electronegativity and an excellent capability of chelating metal cations .
Detergent Additive
STMP is used as an additive in detergents . It helps in improving the cleaning efficiency of the detergents .
Corrosion Inhibitors and Anti-scaling Agents
STMP serves as corrosion inhibitors and anti-scaling agents . It helps in preventing the corrosion of metals and scaling in various applications .
7. Fillers, Finishing Agents, Plating Agents, and Surface Treating Agents STMP is used as fillers, finishing agents, plating agents, and surface treating agents . It helps in improving the performance and efficiency of these applications .
Food Additives
STMP is used as a food additive . It is one of the most popular cross-linkers used in food chemistry .
Direcciones Futuras
The global sodium trimetaphosphate market is projected to expand at a CAGR of 5.4% during the forecast years of 2022-2032 . It is used as a crosslinking agent in the esterification of starch, which projects immense growth opportunities . The demand for sodium trimetaphosphate is also expected to rise due to the growing desire for convenience and processed foods .
Mecanismo De Acción
Target of Action
Sodium trimetaphosphate (STMP) is a metaphosphate of sodium . It is primarily used in the food and construction industries . In the field of biomedical applications, STMP has been used as a crosslinking additive in the development of conductive hydrogels .
Mode of Action
STMP interacts with its targets through a process of crosslinking. For instance, in the development of conductive hydrogels, STMP is incorporated along with other additives such as glycerol. The addition of STMP allows sufficient physical crosslinking with the polymer, resulting in improved hydrogel conductivity, wettability, and rheological properties .
Biochemical Pathways
The primary biochemical pathway involving STMP is its role in the synthesis of ATP components. It has been suggested that STMP could have played a significant role in the early Earth’s biomolecule synthesis and phosphorylation . Various trimetaphosphate-mediated reaction pathways have been discussed, emphasizing the role of trimetaphosphate in prebiotic chemistry .
Pharmacokinetics
It is known that stmp is a colorless solid that is soluble in water but insoluble in alcohol . This solubility could potentially affect its bioavailability, but more research is needed to fully understand its pharmacokinetic properties.
Result of Action
The molecular and cellular effects of STMP’s action are primarily seen in its role as a crosslinking agent. For example, in the development of conductive hydrogels, the addition of STMP results in improved hydrogel conductivity, wettability, and rheological properties .
Action Environment
The action, efficacy, and stability of STMP can be influenced by various environmental factors. For instance, the synthesis of STMP from sodium chloride and orthophosphoric acid was found to be strongly influenced by the reaction temperature and the volume-mean diameter of sodium chloride . Additionally, the use of STMP in the development of conductive hydrogels showed that the concentration of STMP influenced the physical crosslinking with the polymer .
Propiedades
IUPAC Name |
trisodium;2,4,6-trioxido-1,3,5,2λ5,4λ5,6λ5-trioxatriphosphinane 2,4,6-trioxide | |
---|---|---|
Source | PubChem | |
URL | https://pubchem.ncbi.nlm.nih.gov | |
Description | Data deposited in or computed by PubChem | |
InChI |
InChI=1S/3Na.H3O9P3/c;;;1-10(2)7-11(3,4)9-12(5,6)8-10/h;;;(H,1,2)(H,3,4)(H,5,6)/q3*+1;/p-3 | |
Source | PubChem | |
URL | https://pubchem.ncbi.nlm.nih.gov | |
Description | Data deposited in or computed by PubChem | |
InChI Key |
UGTZMIPZNRIWHX-UHFFFAOYSA-K | |
Source | PubChem | |
URL | https://pubchem.ncbi.nlm.nih.gov | |
Description | Data deposited in or computed by PubChem | |
Canonical SMILES |
[O-]P1(=O)OP(=O)(OP(=O)(O1)[O-])[O-].[Na+].[Na+].[Na+] | |
Source | PubChem | |
URL | https://pubchem.ncbi.nlm.nih.gov | |
Description | Data deposited in or computed by PubChem | |
Molecular Formula |
Na3O9P3 | |
Source | PubChem | |
URL | https://pubchem.ncbi.nlm.nih.gov | |
Description | Data deposited in or computed by PubChem | |
Related CAS |
13566-25-1 (Parent) | |
Record name | Sodium trimetaphosphate [USAN] | |
Source | ChemIDplus | |
URL | https://pubchem.ncbi.nlm.nih.gov/substance/?source=chemidplus&sourceid=0007785844 | |
Description | ChemIDplus is a free, web search system that provides access to the structure and nomenclature authority files used for the identification of chemical substances cited in National Library of Medicine (NLM) databases, including the TOXNET system. | |
DSSTOX Substance ID |
DTXSID7052789 | |
Record name | Sodium trimetaphosphate | |
Source | EPA DSSTox | |
URL | https://comptox.epa.gov/dashboard/DTXSID7052789 | |
Description | DSSTox provides a high quality public chemistry resource for supporting improved predictive toxicology. | |
Molecular Weight |
305.89 g/mol | |
Source | PubChem | |
URL | https://pubchem.ncbi.nlm.nih.gov | |
Description | Data deposited in or computed by PubChem | |
Physical Description |
Dry Powder; Liquid; NKRA; Other Solid, Hexahydrate: Efflorescent solid; [Merck Index] White odorless powder; [MSDSonline] | |
Record name | Metaphosphoric acid (H3P3O9), sodium salt (1:3) | |
Source | EPA Chemicals under the TSCA | |
URL | https://www.epa.gov/chemicals-under-tsca | |
Description | EPA Chemicals under the Toxic Substances Control Act (TSCA) collection contains information on chemicals and their regulations under TSCA, including non-confidential content from the TSCA Chemical Substance Inventory and Chemical Data Reporting. | |
Record name | Sodium trimetaphosphate | |
Source | Haz-Map, Information on Hazardous Chemicals and Occupational Diseases | |
URL | https://haz-map.com/Agents/7181 | |
Description | Haz-Map® is an occupational health database designed for health and safety professionals and for consumers seeking information about the adverse effects of workplace exposures to chemical and biological agents. | |
Explanation | Copyright (c) 2022 Haz-Map(R). All rights reserved. Unless otherwise indicated, all materials from Haz-Map are copyrighted by Haz-Map(R). No part of these materials, either text or image may be used for any purpose other than for personal use. Therefore, reproduction, modification, storage in a retrieval system or retransmission, in any form or by any means, electronic, mechanical or otherwise, for reasons other than personal use, is strictly prohibited without prior written permission. | |
Mechanism of Action |
Condensed phosphates (CP) used as food additives depressed the growth of 7 strains of Streptococcus mutans (serotype a-g) as assessed by disk diffusion methods. Minimal inhibitory concn (MIC) of condensed phosphates on Streptococcus mutans appeared to be related to their chelating capacity. The antibacterial action of condensed phosphates seemed largely bacteriostatic. Condensed phosphates depressed lactate production from glucose and sucrose by the cells of strain Kl-R. Condensed phosphates depressed insoluble glucan production from sucrose by the cells of Kl-R. The inhibition of sugar metabolism may be due to the interference of sugar transport into Streptococcus mutans induced by the chelation effects of condensed phosphates. Hamsters were inoculated orally with strain Kl-R and reared on the high-sucrose diet No 2000 supplemented with 2% (wt/wt) condensed phosphates for 60 days. Dietary supplements of condensed phosphates were associated with reduced caries activity and plaque formation. | |
Record name | SODIUM TRIMETAPHOSPHATE | |
Source | Hazardous Substances Data Bank (HSDB) | |
URL | https://pubchem.ncbi.nlm.nih.gov/source/hsdb/5048 | |
Description | The Hazardous Substances Data Bank (HSDB) is a toxicology database that focuses on the toxicology of potentially hazardous chemicals. It provides information on human exposure, industrial hygiene, emergency handling procedures, environmental fate, regulatory requirements, nanomaterials, and related areas. The information in HSDB has been assessed by a Scientific Review Panel. | |
Product Name |
Sodium trimetaphosphate | |
CAS RN |
7785-84-4 | |
Record name | Sodium trimetaphosphate [USAN] | |
Source | ChemIDplus | |
URL | https://pubchem.ncbi.nlm.nih.gov/substance/?source=chemidplus&sourceid=0007785844 | |
Description | ChemIDplus is a free, web search system that provides access to the structure and nomenclature authority files used for the identification of chemical substances cited in National Library of Medicine (NLM) databases, including the TOXNET system. | |
Record name | Metaphosphoric acid (H3P3O9), sodium salt (1:3) | |
Source | EPA Chemicals under the TSCA | |
URL | https://www.epa.gov/chemicals-under-tsca | |
Description | EPA Chemicals under the Toxic Substances Control Act (TSCA) collection contains information on chemicals and their regulations under TSCA, including non-confidential content from the TSCA Chemical Substance Inventory and Chemical Data Reporting. | |
Record name | Sodium trimetaphosphate | |
Source | EPA DSSTox | |
URL | https://comptox.epa.gov/dashboard/DTXSID7052789 | |
Description | DSSTox provides a high quality public chemistry resource for supporting improved predictive toxicology. | |
Record name | Trisodium trimetaphosphate | |
Source | European Chemicals Agency (ECHA) | |
URL | https://echa.europa.eu/substance-information/-/substanceinfo/100.029.171 | |
Description | The European Chemicals Agency (ECHA) is an agency of the European Union which is the driving force among regulatory authorities in implementing the EU's groundbreaking chemicals legislation for the benefit of human health and the environment as well as for innovation and competitiveness. | |
Explanation | Use of the information, documents and data from the ECHA website is subject to the terms and conditions of this Legal Notice, and subject to other binding limitations provided for under applicable law, the information, documents and data made available on the ECHA website may be reproduced, distributed and/or used, totally or in part, for non-commercial purposes provided that ECHA is acknowledged as the source: "Source: European Chemicals Agency, http://echa.europa.eu/". Such acknowledgement must be included in each copy of the material. ECHA permits and encourages organisations and individuals to create links to the ECHA website under the following cumulative conditions: Links can only be made to webpages that provide a link to the Legal Notice page. | |
Record name | Sodium trimetaphosphate | |
Source | FDA Global Substance Registration System (GSRS) | |
URL | https://gsrs.ncats.nih.gov/ginas/app/beta/substances/3IH6169RL0 | |
Description | The FDA Global Substance Registration System (GSRS) enables the efficient and accurate exchange of information on what substances are in regulated products. Instead of relying on names, which vary across regulatory domains, countries, and regions, the GSRS knowledge base makes it possible for substances to be defined by standardized, scientific descriptions. | |
Explanation | Unless otherwise noted, the contents of the FDA website (www.fda.gov), both text and graphics, are not copyrighted. They are in the public domain and may be republished, reprinted and otherwise used freely by anyone without the need to obtain permission from FDA. Credit to the U.S. Food and Drug Administration as the source is appreciated but not required. | |
Record name | SODIUM TRIMETAPHOSPHATE | |
Source | Hazardous Substances Data Bank (HSDB) | |
URL | https://pubchem.ncbi.nlm.nih.gov/source/hsdb/5048 | |
Description | The Hazardous Substances Data Bank (HSDB) is a toxicology database that focuses on the toxicology of potentially hazardous chemicals. It provides information on human exposure, industrial hygiene, emergency handling procedures, environmental fate, regulatory requirements, nanomaterials, and related areas. The information in HSDB has been assessed by a Scientific Review Panel. | |
Synthesis routes and methods
Procedure details
Retrosynthesis Analysis
AI-Powered Synthesis Planning: Our tool employs the Template_relevance Pistachio, Template_relevance Bkms_metabolic, Template_relevance Pistachio_ringbreaker, Template_relevance Reaxys, Template_relevance Reaxys_biocatalysis model, leveraging a vast database of chemical reactions to predict feasible synthetic routes.
One-Step Synthesis Focus: Specifically designed for one-step synthesis, it provides concise and direct routes for your target compounds, streamlining the synthesis process.
Accurate Predictions: Utilizing the extensive PISTACHIO, BKMS_METABOLIC, PISTACHIO_RINGBREAKER, REAXYS, REAXYS_BIOCATALYSIS database, our tool offers high-accuracy predictions, reflecting the latest in chemical research and data.
Strategy Settings
Precursor scoring | Relevance Heuristic |
---|---|
Min. plausibility | 0.01 |
Model | Template_relevance |
Template Set | Pistachio/Bkms_metabolic/Pistachio_ringbreaker/Reaxys/Reaxys_biocatalysis |
Top-N result to add to graph | 6 |
Feasible Synthetic Routes
Q & A
ANone: Sodium trimetaphosphate (STMP) primarily interacts through chelation, forming stable complexes with metal cations. This property underpins many of its applications.
- In starch modification: STMP cross-links starch molecules by forming phosphate ester bonds with hydroxyl groups. [] This increases viscosity, improves freeze-thaw stability, and enhances shear and acid tolerance. [, ]
- In biomaterial applications: STMP crosslinks polymers like chitosan [] and gums [], influencing microparticle size, swelling capacity, and degradation rate, ultimately impacting drug release profiles. [, ]
- In enamel protection: STMP interacts with calcium ions, potentially forming calcium phosphate complexes on the enamel surface, hindering demineralization and promoting remineralization. [, , ]
ANone: Sodium trimetaphosphate is a cyclic triphosphate.
- Spectroscopic data:
ANone: STMP exhibits good material compatibility with various polymers and is stable under a range of conditions, making it suitable for diverse applications.
- Compatibility: STMP demonstrates compatibility with starches, [, , , ] cellulose, [] chitosan, [] gums, [] and polyvinylidene fluoride. []
- Stability:
- Thermal stability: STMP is stable at high temperatures, enabling its use in processes like starch modification at 130°C. []
- Hydrolytic stability: While generally stable, STMP can undergo hydrolysis in highly acidic or alkaline conditions. [, ]
- Grinding stability: Grinding STMP, especially at low pressure, can lead to its transformation to amorphous forms and the generation of higher chain phosphates. []
ANone: While not a traditional catalyst, STMP demonstrates interesting behavior in prebiotic chemistry.
- Peptide synthesis: STMP facilitates the formation of oligopeptides from glycine in alkaline conditions, potentially playing a role in prebiotic peptide synthesis. [] The addition of imidazole enhances this effect. []
ANone: Currently, the provided research articles do not delve extensively into the use of computational chemistry and modeling for sodium trimetaphosphate.
ANone: Limited information on the SAR of STMP is available within the provided research papers. Further investigation is required to understand how modifications to its cyclic structure influence its activity and selectivity.
- Stability: Controlling pH is crucial for maintaining STMP stability as extreme pH can induce hydrolysis. []
- Solubility: STMP exhibits good water solubility. []
- Bioavailability: While bioavailability data is not explicitly discussed, encapsulating STMP within microparticles, like those based on chitosan [] or xylan, [] can provide controlled release and potentially enhance its bioavailability.
ANone: The provided research primarily focuses on STMP's material properties and applications. Further research is needed to elucidate its PK/PD profile, including ADME characteristics and in vivo activity.
ANone: Several studies highlight the in vitro efficacy of STMP:
- Enamel protection: In vitro studies demonstrate that STMP, especially in nano-sized formulations, enhances the protective effect of fluoride against enamel demineralization. [, , ]
- Antimicrobial activity: STMP combined with silver nanoparticles shows antimicrobial efficacy against Streptococcus mutans and Candida albicans biofilms in vitro. []
- Biofilm modulation: TMPn, particularly with fluoride, can influence biofilm composition, increasing P and F levels and affecting ECM components. []
ANone: The provided research focuses primarily on material properties and in vitro applications of STMP, with no mention of resistance mechanisms. Further research is required to address this aspect.
A: While STMP is generally recognized as safe for use in food and cosmetics, [] high doses have been associated with adverse effects in animal studies:
- High-dose effects in rats: Rats fed a diet containing 10% STMP showed transient tubular necrosis. [] Other studies reported growth inhibition, kidney abnormalities, bone decalcification, and liver changes with chronic high-dose exposure. []
- Skin and eye irritation: High concentrations of STMP can be irritating to the skin and eyes. []
ANone: STMP research bridges disciplines like:
- Material science: Exploiting its cross-linking properties to modify starch, [, , , ] develop biomaterials, [, ] and create novel gel polymer electrolytes. []
- Dentistry: Investigating its role in enamel protection and remineralization. [, , ]
- Microbiology: Studying its impact on biofilms and potential as an antimicrobial agent. [, , ]
- Prebiotic chemistry: Exploring its involvement in peptide synthesis under early Earth conditions. []
ANone: Several alternatives to STMP exist, each with its own profile:
- Glutaraldehyde (GA): Commonly used as a crosslinking agent, but STMP-crosslinked scaffolds show higher degradation rates and superior cell viability compared to GA. []
- Sodium tripolyphosphate (STPP): Another crosslinking agent, but its effectiveness in starch phosphorylation is influenced by pH differently than STMP. []
- Other crosslinking agents: Epichlorohydrin is an alternative for starch modification, but STMP-crosslinked starch hydrogels exhibit higher swelling. []
Descargo de responsabilidad e información sobre productos de investigación in vitro
Tenga en cuenta que todos los artículos e información de productos presentados en BenchChem están destinados únicamente con fines informativos. Los productos disponibles para la compra en BenchChem están diseñados específicamente para estudios in vitro, que se realizan fuera de organismos vivos. Los estudios in vitro, derivados del término latino "in vidrio", involucran experimentos realizados en entornos de laboratorio controlados utilizando células o tejidos. Es importante tener en cuenta que estos productos no se clasifican como medicamentos y no han recibido la aprobación de la FDA para la prevención, tratamiento o cura de ninguna condición médica, dolencia o enfermedad. Debemos enfatizar que cualquier forma de introducción corporal de estos productos en humanos o animales está estrictamente prohibida por ley. Es esencial adherirse a estas pautas para garantizar el cumplimiento de los estándares legales y éticos en la investigación y experimentación.