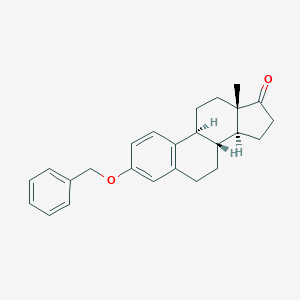
Éter Bencílico de Estrona
Descripción general
Descripción
Estrone Benzyl Ether is a derivative of estrone, a naturally occurring estrogen hormone
Aplicaciones Científicas De Investigación
Estrone Benzyl Ether has a wide range of applications in scientific research:
Chemistry: It serves as a precursor for the synthesis of various estrone derivatives with potential biological activities.
Biology: The compound is used in studies related to hormone receptor interactions and estrogenic activity.
Medicine: Research on Estrone Benzyl Ether includes its potential use in hormone replacement therapy and cancer treatment.
Industry: It is utilized in the development of pharmaceuticals and as an intermediate in the synthesis of other bioactive compounds
Mecanismo De Acción
Target of Action
Estrone Benzyl Ether, also known as 3-O-Benzyl Estrone, is a derivative of estrone . Estrone is a naturally occurring estrogen, specifically an agonist of the estrogen receptors (ERs) ERα and ERβ . It is a relatively weak estrogen compared to estradiol .
Mode of Action
Estrone, and by extension Estrone Benzyl Ether, enters the cells of responsive tissues (e.g., female organs, breasts, hypothalamus, pituitary) where they interact with estrogen receptors . Hormone-bound estrogen receptors dimerize, translocate to the nucleus of cells, and bind to estrogen response elements (ERE) of genes . This interaction leads to the regulation of gene transcription, which results in the formation of messenger RNA .
Biochemical Pathways
Estrone is produced in vivo from androstenedione or from testosterone via estradiol . It is primarily produced in the ovaries, placenta, and in peripheral tissues (especially adipose tissue) through the conversion of androstenedione . Estrone may be further metabolized to 16-alpha-hydroxyestrone, which may be reduced to estriol by estradiol dehydrogenase . In the case of estrone biodegradation, it gets degraded to intermediates such as estradiol, 3-phenyl-undecane, etc., that may eventually get converted into carbon dioxide and water .
Pharmacokinetics
Estrone, and by extension Estrone Benzyl Ether, is metabolized in the liver via hydroxylation, sulfation, and glucuronidation . The metabolites include estradiol, estrone sulfate, estrone glucuronide, among others .
Result of Action
The molecular and cellular effects of Estrone Benzyl Ether’s action are similar to those of estrone. As an estrogen, estrone increases the hepatic synthesis of sex hormone-binding globulin (SHBG), thyroid-binding globulin (TBG), and other serum proteins and suppresses follicle-stimulating hormone (FSH) release from the anterior pituitary .
Action Environment
Environmental factors can influence the action, efficacy, and stability of Estrone Benzyl Ether. For instance, estrone, an emerging pollutant, accumulates in the environment and becomes a potential risk to human and wildlife health . Exposure to exogenous estrone results in erroneous hormonal function causing reproductive disorders, metabolic disorders, and cancers . Thus, the environmental presence of estrone and its derivatives can have significant impacts on health and disease.
Análisis Bioquímico
Biochemical Properties
Estrone Benzyl Ether interacts with various enzymes, proteins, and other biomolecules in biochemical reactions. Estrone, the parent compound, is produced in vivo from androstenedione or from testosterone via estradiol . It may be further metabolized to 16-alpha-hydroxyestrone, which may be reduced to estriol by estradiol dehydrogenase .
Cellular Effects
Estrone Benzyl Ether has shown substantial antiproliferative activity against various human adherent gynecological cancer cell lines . A pronounced proapoptotic effect was observed on three cervical cell lines . Additionally, cell cycle blockade in the G2/M phase was detected, which might be a consequence of the effect of the compound on tubulin polymerization .
Molecular Mechanism
The parent compound, estrone, enters the cells of responsive tissues where it interacts with estrogen receptors . Hormone-bound estrogen receptors dimerize, translocate to the nucleus of cells, and bind to estrogen response elements (ERE) of genes .
Metabolic Pathways
Estrone Benzyl Ether is likely involved in similar metabolic pathways as estrone. Estrone is produced primarily in the ovaries, placenta, and in peripheral tissues (especially adipose tissue) through conversion of androstenedione .
Métodos De Preparación
Synthetic Routes and Reaction Conditions
Estrone Benzyl Ether can be synthesized through several methods. One common approach involves the benzylation of estrone using benzyl bromide in the presence of a base such as potassium carbonate. The reaction typically occurs in an organic solvent like acetone or dimethylformamide (DMF) under reflux conditions. The product is then purified through recrystallization or column chromatography .
Industrial Production Methods
Industrial production of Estrone Benzyl Ether may involve similar synthetic routes but on a larger scale. The process would be optimized for efficiency, yield, and cost-effectiveness. This could include the use of continuous flow reactors and automated purification systems to streamline the production process.
Análisis De Reacciones Químicas
Types of Reactions
Estrone Benzyl Ether undergoes various chemical reactions, including:
Oxidation: The compound can be oxidized to form corresponding ketones or aldehydes.
Reduction: Reduction reactions can convert the benzyl ether group back to the hydroxyl group.
Substitution: The benzyl group can be substituted with other functional groups through nucleophilic substitution reactions.
Common Reagents and Conditions
Oxidation: Common oxidizing agents include potassium permanganate (KMnO₄) and chromium trioxide (CrO₃).
Reduction: Reducing agents such as lithium aluminum hydride (LiAlH₄) or sodium borohydride (NaBH₄) are used.
Substitution: Nucleophiles like sodium methoxide (NaOMe) or sodium ethoxide (NaOEt) can be employed for substitution reactions.
Major Products
The major products formed from these reactions depend on the specific conditions and reagents used. For example, oxidation may yield estrone ketone derivatives, while reduction can regenerate estrone from Estrone Benzyl Ether.
Comparación Con Compuestos Similares
Similar Compounds
Estrone: The parent compound of Estrone Benzyl Ether, with similar estrogenic activity but different chemical properties.
Estradiol: Another estrogen hormone with higher potency than estrone.
Estriol: A weaker estrogen compared to estrone and estradiol, often used in hormone replacement therapy.
Uniqueness
Estrone Benzyl Ether is unique due to the presence of the benzyl ether group, which imparts distinct chemical properties and potential biological activities. This modification can enhance the compound’s stability, solubility, and interaction with biological targets, making it a valuable tool in scientific research .
Actividad Biológica
3-O-Benzyl Estrone (3-O-BE) is a synthetic derivative of estrone, notable for its significant biological activity, particularly in relation to estrogen receptors. This compound has garnered attention in medicinal chemistry due to its potential applications in hormone replacement therapy and cancer treatment. The following sections explore the biological activity, mechanisms of action, and relevant research findings associated with 3-O-Benzyl Estrone.
Chemical Structure and Properties
3-O-Benzyl Estrone is characterized by the addition of a benzyl group at the 3-position of the estrone molecule. Its chemical formula is CHO, with a molecular weight of approximately 360.49 g/mol. The compound typically appears as a white solid and is soluble in organic solvents, making it suitable for various chemical applications.
The biological activity of 3-O-BE primarily stems from its interaction with estrogen receptors (ERs). Upon binding to ERs, 3-O-BE can activate estrogen signaling pathways, leading to the transcription of estrogen-responsive genes that regulate various physiological processes such as cell proliferation and differentiation. The compound's mechanism involves:
- Binding Affinity : 3-O-BE exhibits a well-defined binding affinity to ER alpha (ERα) and ER beta (ERβ), which are crucial for mediating estrogenic effects in target tissues.
- Regulation of Gene Expression : Activation of these receptors influences downstream gene expression involved in reproductive health, metabolic processes, and cancer progression.
Biological Activity
Research indicates that 3-O-Benzyl Estrone possesses significant biological activities:
- Estrogenic Activity : The compound retains some estrogenic properties, allowing it to mimic natural estrogens. This characteristic is particularly useful in studies exploring estrogen signaling pathways.
- Antiproliferative Effects : Studies have demonstrated that 3-O-BE can inhibit cell proliferation in various cancer cell lines, particularly those related to gynecological cancers. For instance, it has shown low micromolar cell growth-inhibitory potential against human adherent cancer cell lines .
- Inhibition of Cancer Cell Migration : In vitro assays have revealed that 3-O-BE can inhibit the migration and invasive ability of breast cancer cells, suggesting its potential as an antimetastatic agent .
Comparative Biological Activity
To better understand the unique properties of 3-O-Benzyl Estrone, a comparison with structurally similar compounds is beneficial:
Compound Name | Structure Description | Unique Features |
---|---|---|
Estrone | CHO | Natural estrogen; primary precursor to estradiol. |
17β-Estradiol | CHO | More potent than estrone; primary endogenous estrogen. |
3-O-Sulfamoyl Estrone | CHNOS | Enhances water solubility; used for drug delivery. |
16α-Hydroxyestrone | CHO | Metabolite of estradiol; exhibits unique biological properties. |
The modification at the 3-position with a benzyl group in 3-O-BE alters its pharmacological profile, enhancing its potential as an inhibitor of estrogen-related pathways while still maintaining some level of estrogenic activity.
Research Findings and Case Studies
- Anticancer Properties : A study highlighted that compounds similar to 3-O-BE exhibited antiproliferative activities against human gynecological cancer cell lines. The introduction of a benzyl moiety was found to enhance the antiproliferative potential significantly .
- Cell Cycle Disruption : Flow cytometry analyses demonstrated that certain analogs of 3-O-BE could induce disturbances in the cell cycle, leading to increased hypodiploid populations in breast cancer cells .
- Microtubule Stabilization : Investigations into the mechanistic actions of related compounds revealed microtubule-stabilizing activity, further supporting their potential as anticancer agents .
Propiedades
IUPAC Name |
(8R,9S,13S,14S)-13-methyl-3-phenylmethoxy-7,8,9,11,12,14,15,16-octahydro-6H-cyclopenta[a]phenanthren-17-one | |
---|---|---|
Source | PubChem | |
URL | https://pubchem.ncbi.nlm.nih.gov | |
Description | Data deposited in or computed by PubChem | |
InChI |
InChI=1S/C25H28O2/c1-25-14-13-21-20-10-8-19(27-16-17-5-3-2-4-6-17)15-18(20)7-9-22(21)23(25)11-12-24(25)26/h2-6,8,10,15,21-23H,7,9,11-14,16H2,1H3/t21-,22-,23+,25+/m1/s1 | |
Source | PubChem | |
URL | https://pubchem.ncbi.nlm.nih.gov | |
Description | Data deposited in or computed by PubChem | |
InChI Key |
MSINETGATWEUAB-AHCIIZGASA-N | |
Source | PubChem | |
URL | https://pubchem.ncbi.nlm.nih.gov | |
Description | Data deposited in or computed by PubChem | |
Canonical SMILES |
CC12CCC3C(C1CCC2=O)CCC4=C3C=CC(=C4)OCC5=CC=CC=C5 | |
Source | PubChem | |
URL | https://pubchem.ncbi.nlm.nih.gov | |
Description | Data deposited in or computed by PubChem | |
Isomeric SMILES |
C[C@]12CC[C@H]3[C@H]([C@@H]1CCC2=O)CCC4=C3C=CC(=C4)OCC5=CC=CC=C5 | |
Source | PubChem | |
URL | https://pubchem.ncbi.nlm.nih.gov | |
Description | Data deposited in or computed by PubChem | |
Molecular Formula |
C25H28O2 | |
Source | PubChem | |
URL | https://pubchem.ncbi.nlm.nih.gov | |
Description | Data deposited in or computed by PubChem | |
DSSTOX Substance ID |
DTXSID50456391 | |
Record name | Estrone Benzyl Ether | |
Source | EPA DSSTox | |
URL | https://comptox.epa.gov/dashboard/DTXSID50456391 | |
Description | DSSTox provides a high quality public chemistry resource for supporting improved predictive toxicology. | |
Molecular Weight |
360.5 g/mol | |
Source | PubChem | |
URL | https://pubchem.ncbi.nlm.nih.gov | |
Description | Data deposited in or computed by PubChem | |
CAS No. |
858-98-0 | |
Record name | Estrone Benzyl Ether | |
Source | EPA DSSTox | |
URL | https://comptox.epa.gov/dashboard/DTXSID50456391 | |
Description | DSSTox provides a high quality public chemistry resource for supporting improved predictive toxicology. | |
Record name | (8R,9S,13S,14S)-13-methyl-3-phenylmethoxy-7,8,9,11,12,14,15,16-octahydro-6H-cyclopenta[a]phenanthren-17-one | |
Source | European Chemicals Agency (ECHA) | |
URL | https://echa.europa.eu/information-on-chemicals | |
Description | The European Chemicals Agency (ECHA) is an agency of the European Union which is the driving force among regulatory authorities in implementing the EU's groundbreaking chemicals legislation for the benefit of human health and the environment as well as for innovation and competitiveness. | |
Explanation | Use of the information, documents and data from the ECHA website is subject to the terms and conditions of this Legal Notice, and subject to other binding limitations provided for under applicable law, the information, documents and data made available on the ECHA website may be reproduced, distributed and/or used, totally or in part, for non-commercial purposes provided that ECHA is acknowledged as the source: "Source: European Chemicals Agency, http://echa.europa.eu/". Such acknowledgement must be included in each copy of the material. ECHA permits and encourages organisations and individuals to create links to the ECHA website under the following cumulative conditions: Links can only be made to webpages that provide a link to the Legal Notice page. | |
Synthesis routes and methods
Procedure details
Retrosynthesis Analysis
AI-Powered Synthesis Planning: Our tool employs the Template_relevance Pistachio, Template_relevance Bkms_metabolic, Template_relevance Pistachio_ringbreaker, Template_relevance Reaxys, Template_relevance Reaxys_biocatalysis model, leveraging a vast database of chemical reactions to predict feasible synthetic routes.
One-Step Synthesis Focus: Specifically designed for one-step synthesis, it provides concise and direct routes for your target compounds, streamlining the synthesis process.
Accurate Predictions: Utilizing the extensive PISTACHIO, BKMS_METABOLIC, PISTACHIO_RINGBREAKER, REAXYS, REAXYS_BIOCATALYSIS database, our tool offers high-accuracy predictions, reflecting the latest in chemical research and data.
Strategy Settings
Precursor scoring | Relevance Heuristic |
---|---|
Min. plausibility | 0.01 |
Model | Template_relevance |
Template Set | Pistachio/Bkms_metabolic/Pistachio_ringbreaker/Reaxys/Reaxys_biocatalysis |
Top-N result to add to graph | 6 |
Feasible Synthetic Routes
Q1: What makes estrone benzyl ether derivatives interesting for anti-cancer research?
A1: Research shows that modifications to the estrone benzyl ether structure, specifically at the 16-position, can yield compounds with promising antiproliferative and antimetastatic properties. For example, 16-azidomethyl substituted 3-O-benzyl estrone analogs (16AABE and 16BABE) demonstrated significant activity against gynecological cancer cell lines, including breast cancer. [] These analogs were shown to: * Increase hypodiploid populations of breast cancer cells, indicating cell death. []* Induce cell cycle disturbances, further hindering cancer cell proliferation. []* Inhibit breast cancer cell migration and invasion, highlighting their potential as antimetastatic agents. []
Q2: How do these 16-azidomethyl substituted estrone analogs exert their anti-cancer effects?
A2: While the exact mechanisms are still under investigation, research suggests these analogs might function through multiple pathways:
- Estrogenic Activity: Both 16AABE and 16BABE exhibited considerable estrogenic activity in reporter gene assays. [] This suggests they may influence cancer cell behavior by interacting with estrogen receptors.
- Tubulin Polymerization: These analogs significantly increased the rate of tubulin polymerization in vitro. [] Tubulin is crucial for cell division, and its disruption can inhibit cancer cell growth.
Q3: Beyond modifications at the 16-position, are there other areas of the estrone benzyl ether structure being explored for functionalization?
A3: Yes, studies have investigated functionalizations of estrone benzyl ether at the 11 and 12 positions. [, ] While specific details regarding the outcomes of these modifications are not available in the provided abstracts, it highlights the ongoing interest in exploring the structure-activity relationship of this molecule.
Descargo de responsabilidad e información sobre productos de investigación in vitro
Tenga en cuenta que todos los artículos e información de productos presentados en BenchChem están destinados únicamente con fines informativos. Los productos disponibles para la compra en BenchChem están diseñados específicamente para estudios in vitro, que se realizan fuera de organismos vivos. Los estudios in vitro, derivados del término latino "in vidrio", involucran experimentos realizados en entornos de laboratorio controlados utilizando células o tejidos. Es importante tener en cuenta que estos productos no se clasifican como medicamentos y no han recibido la aprobación de la FDA para la prevención, tratamiento o cura de ninguna condición médica, dolencia o enfermedad. Debemos enfatizar que cualquier forma de introducción corporal de estos productos en humanos o animales está estrictamente prohibida por ley. Es esencial adherirse a estas pautas para garantizar el cumplimiento de los estándares legales y éticos en la investigación y experimentación.