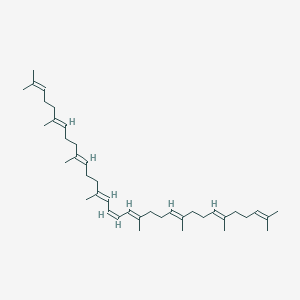
15-cis-Fitóeno
Descripción general
Descripción
15-cis-Phytoene is a colorless carotenoid and a key intermediate in the biosynthesis of carotenoids, which are essential pigments in plants, algae, and some bacteria. It is the first C40 carotenoid formed in the carotenoid biosynthesis pathway and serves as a precursor to other carotenoids such as lycopene, beta-carotene, and xanthophylls .
Aplicaciones Científicas De Investigación
15-cis-Phytoene has several scientific research applications:
Mecanismo De Acción
Target of Action
The primary target of 15-cis-Phytoene is the enzyme 15-cis-phytoene desaturase (PDS) . This enzyme plays a crucial role in the carotenoid biosynthesis in plants and cyanobacteria . It is a membrane-bound enzyme localized in plastids .
Mode of Action
15-cis-Phytoene interacts with its target, the PDS enzyme, by serving as a substrate . The PDS enzyme introduces two double bonds into the colorless substrate phytoene through dehydrogenation and isomerizes two additional double bonds . This interaction results in the conversion of 15-cis-phytoene into 9,15,9’-tri-cis-ζ-carotene .
Biochemical Pathways
The interaction of 15-cis-Phytoene with PDS starts a biochemical pathway involving three further enzymes: zeta-carotene isomerase, zeta-carotene desaturase, and carotene cis-trans isomerase . This pathway, known as the poly-cis pathway, leads to the formation of the red-colored lycopene . In bacteria and fungi, the homologous phytoene desaturase converts phytoene directly to lycopene via an all-trans pathway .
Pharmacokinetics
It is known that the compound plays a significant role in the biosynthesis of carotenoids, which are crucial for plant growth and development .
Result of Action
The action of 15-cis-Phytoene results in the synthesis of β-carotene, which influences the color of certain fruits . For instance, in apricots, a mutation in the gene encoding PDS disrupted the β-carotene biosynthesis pathway, resulting in decreased β-carotene content and a white phenotype .
Action Environment
The action of 15-cis-Phytoene can be influenced by various environmental factors. For example, the expression of the genes involved in β-carotene synthesis, including those encoding PDS, can significantly differ between different cultivars during the color transition stage . This suggests that environmental factors such as light, temperature, and soil conditions, which can influence gene expression, may also affect the action of 15-cis-Phytoene.
Análisis Bioquímico
Biochemical Properties
15-cis-Phytoene interacts with several enzymes and proteins in the carotenoid biosynthesis pathway. The most significant interaction is with the enzyme phytoene synthase (PSY), which catalyzes the first committed step of carotenogenesis . This interaction is crucial for the production of carotenoids, which are essential for various biological functions, including photoprotection and light-harvesting in photosynthesis .
Cellular Effects
15-cis-Phytoene influences various cellular processes, primarily through its role in carotenoid biosynthesis. It affects cell function by influencing cell signaling pathways, gene expression, and cellular metabolism . For instance, the synthesis of β-carotene, which is derived from 15-cis-Phytoene, can influence the color of apricot pulp .
Molecular Mechanism
The molecular mechanism of 15-cis-Phytoene involves binding interactions with biomolecules, enzyme activation, and changes in gene expression. Phytoene synthase (PSY) catalyzes the condensation of two GGPP molecules to produce 15-cis-Phytoene . This is the first committed step in carotenogenesis, leading to the production of carotenoids .
Metabolic Pathways
15-cis-Phytoene is involved in the carotenoid biosynthesis pathway . It interacts with enzymes such as phytoene synthase (PSY) and is a precursor for the synthesis of other carotenoids .
Subcellular Localization
15-cis-Phytoene is synthesized in plastids, which are a type of organelle found in plant cells Its activity and function are closely tied to its location within these organelles
Métodos De Preparación
Synthetic Routes and Reaction Conditions
15-cis-Phytoene can be synthesized through the enzymatic condensation of two molecules of geranylgeranyl pyrophosphate. This reaction is catalyzed by the enzyme phytoene synthase. The reaction conditions typically involve the presence of magnesium ions and a suitable buffer system to maintain the pH .
Industrial Production Methods
In industrial settings, 15-cis-Phytoene is produced using genetically engineered microorganisms such as Escherichia coli or yeast. These microorganisms are modified to express the necessary enzymes for the biosynthesis of 15-cis-Phytoene from simple carbon sources. The production process involves fermentation, followed by extraction and purification of the compound .
Análisis De Reacciones Químicas
Types of Reactions
15-cis-Phytoene undergoes several types of reactions, including:
Desaturation: Catalyzed by phytoene desaturase, converting 15-cis-Phytoene to 9,15,9’-tri-cis-zeta-carotene.
Isomerization: Conversion of cis double bonds to trans double bonds, which is essential for the formation of lycopene.
Common Reagents and Conditions
Desaturation: Requires the enzyme phytoene desaturase and plastoquinone as an electron acceptor.
Isomerization: Often occurs spontaneously under light or can be catalyzed by specific isomerase enzymes.
Major Products Formed
9,15,9’-tri-cis-zeta-carotene: Formed through desaturation of 15-cis-Phytoene.
Lycopene: Formed through further desaturation and isomerization steps.
Comparación Con Compuestos Similares
Similar Compounds
Phytofluene: Another early intermediate in the carotenoid biosynthesis pathway, formed from 15-cis-Phytoene.
Zeta-carotene: Formed through the desaturation of phytofluene.
Lycopene: A red carotenoid formed through further desaturation and isomerization of zeta-carotene.
Uniqueness
15-cis-Phytoene is unique in its structure as the first C40 carotenoid in the biosynthesis pathway. Its formation marks the commitment to carotenoid biosynthesis, and its subsequent transformations lead to the production of a wide variety of carotenoids with diverse functions and applications .
Actividad Biológica
15-cis-Phytoene is a significant carotenoid precursor in the biosynthesis of various carotenoids, including β-carotene. This compound plays a crucial role in plant physiology, particularly in the synthesis of pigments that are essential for photosynthesis and plant health. The biological activity of 15-cis-phytoene encompasses its synthesis, metabolic pathways, and potential health benefits.
1. Biosynthesis and Metabolic Pathways
15-cis-Phytoene is synthesized from two molecules of geranylgeranyl pyrophosphate (GGPP) through the action of phytoene synthase (PSY), which catalyzes the first committed step in carotenoid biosynthesis. This pathway is crucial as it leads to the formation of various carotenoids that contribute to plant coloration and photoprotection.
Key Enzymes Involved
- Phytoene Synthase (PSY) : Catalyzes the condensation of GGPP to form 15-cis-phytoene.
- Phytoene Desaturase (PDS) : Converts 15-cis-phytoene into other carotenoids through a series of desaturation steps, ultimately leading to lycopene.
Enzyme | Function | Product |
---|---|---|
PSY | Condensation of GGPP | 15-cis-Phytoene |
PDS | Desaturation | Lycopene |
2. Biological Activity and Health Benefits
Research indicates that carotenoids, including those derived from 15-cis-phytoene, possess various biological activities that can benefit human health:
- Antioxidant Properties : Carotenoids exhibit significant antioxidant activity, helping to neutralize free radicals and reduce oxidative stress. This property is beneficial for preventing chronic diseases such as cancer and cardiovascular diseases.
- Vision Health : Carotenoids are known to support eye health by protecting against age-related macular degeneration (AMD) and other vision-related issues.
- Skin Protection : Carotenoids can enhance skin health by providing protection against UV radiation and improving skin hydration and elasticity.
3. Case Studies and Research Findings
Several studies have explored the effects of 15-cis-phytoene and its derivatives on plant health and human nutrition:
Study on Apricot Coloration
A study investigated the role of 15-cis-phytoene desaturase (PDS) in apricot fruit coloration. It was found that mutations in the PDS gene led to reduced β-carotene content, affecting fruit color and potentially its nutritional value. This highlights the importance of carotenoid biosynthesis in fruit quality .
Carotenoid Biosynthesis in Maize
In maize, mutations affecting the Z-ISO gene were shown to block carotenoid production, leading to an accumulation of cis-carotenes, including 15-cis-phytoene. This research emphasizes the genetic regulation of carotenoid biosynthesis pathways .
4. Future Directions in Research
Further research is necessary to fully understand the regulatory mechanisms governing 15-cis-phytoene metabolism and its potential applications in agricultural biotechnology. Genetic engineering approaches could enhance carotenoid content in crops, improving their nutritional profiles.
Propiedades
IUPAC Name |
(6E,10E,14E,16Z,18E,22E,26E)-2,6,10,14,19,23,27,31-octamethyldotriaconta-2,6,10,14,16,18,22,26,30-nonaene | |
---|---|---|
Source | PubChem | |
URL | https://pubchem.ncbi.nlm.nih.gov | |
Description | Data deposited in or computed by PubChem | |
InChI |
InChI=1S/C40H64/c1-33(2)19-13-23-37(7)27-17-31-39(9)29-15-25-35(5)21-11-12-22-36(6)26-16-30-40(10)32-18-28-38(8)24-14-20-34(3)4/h11-12,19-22,27-30H,13-18,23-26,31-32H2,1-10H3/b12-11-,35-21+,36-22+,37-27+,38-28+,39-29+,40-30+ | |
Source | PubChem | |
URL | https://pubchem.ncbi.nlm.nih.gov | |
Description | Data deposited in or computed by PubChem | |
InChI Key |
YVLPJIGOMTXXLP-BHLJUDRVSA-N | |
Source | PubChem | |
URL | https://pubchem.ncbi.nlm.nih.gov | |
Description | Data deposited in or computed by PubChem | |
Canonical SMILES |
CC(=CCCC(=CCCC(=CCCC(=CC=CC=C(C)CCC=C(C)CCC=C(C)CCC=C(C)C)C)C)C)C | |
Source | PubChem | |
URL | https://pubchem.ncbi.nlm.nih.gov | |
Description | Data deposited in or computed by PubChem | |
Isomeric SMILES |
CC(=CCC/C(=C/CC/C(=C/CC/C(=C/C=C\C=C(\CC/C=C(/CC/C=C(/CCC=C(C)C)\C)\C)/C)/C)/C)/C)C | |
Source | PubChem | |
URL | https://pubchem.ncbi.nlm.nih.gov | |
Description | Data deposited in or computed by PubChem | |
Molecular Formula |
C40H64 | |
Source | PubChem | |
URL | https://pubchem.ncbi.nlm.nih.gov | |
Description | Data deposited in or computed by PubChem | |
DSSTOX Substance ID |
DTXSID10433340 | |
Record name | 15-cis-Phytoene | |
Source | EPA DSSTox | |
URL | https://comptox.epa.gov/dashboard/DTXSID10433340 | |
Description | DSSTox provides a high quality public chemistry resource for supporting improved predictive toxicology. | |
Molecular Weight |
544.9 g/mol | |
Source | PubChem | |
URL | https://pubchem.ncbi.nlm.nih.gov | |
Description | Data deposited in or computed by PubChem | |
CAS No. |
13920-14-4 | |
Record name | Phytoene | |
Source | CAS Common Chemistry | |
URL | https://commonchemistry.cas.org/detail?cas_rn=13920-14-4 | |
Description | CAS Common Chemistry is an open community resource for accessing chemical information. Nearly 500,000 chemical substances from CAS REGISTRY cover areas of community interest, including common and frequently regulated chemicals, and those relevant to high school and undergraduate chemistry classes. This chemical information, curated by our expert scientists, is provided in alignment with our mission as a division of the American Chemical Society. | |
Explanation | The data from CAS Common Chemistry is provided under a CC-BY-NC 4.0 license, unless otherwise stated. | |
Record name | Phytoene | |
Source | ChemIDplus | |
URL | https://pubchem.ncbi.nlm.nih.gov/substance/?source=chemidplus&sourceid=0013920144 | |
Description | ChemIDplus is a free, web search system that provides access to the structure and nomenclature authority files used for the identification of chemical substances cited in National Library of Medicine (NLM) databases, including the TOXNET system. | |
Record name | 15-cis-Phytoene | |
Source | EPA DSSTox | |
URL | https://comptox.epa.gov/dashboard/DTXSID10433340 | |
Description | DSSTox provides a high quality public chemistry resource for supporting improved predictive toxicology. | |
Record name | PHYTOENE | |
Source | FDA Global Substance Registration System (GSRS) | |
URL | https://gsrs.ncats.nih.gov/ginas/app/beta/substances/876K2ZK1OF | |
Description | The FDA Global Substance Registration System (GSRS) enables the efficient and accurate exchange of information on what substances are in regulated products. Instead of relying on names, which vary across regulatory domains, countries, and regions, the GSRS knowledge base makes it possible for substances to be defined by standardized, scientific descriptions. | |
Explanation | Unless otherwise noted, the contents of the FDA website (www.fda.gov), both text and graphics, are not copyrighted. They are in the public domain and may be republished, reprinted and otherwise used freely by anyone without the need to obtain permission from FDA. Credit to the U.S. Food and Drug Administration as the source is appreciated but not required. | |
Record name | 15-cis-Phytoene | |
Source | Human Metabolome Database (HMDB) | |
URL | http://www.hmdb.ca/metabolites/HMDB0039093 | |
Description | The Human Metabolome Database (HMDB) is a freely available electronic database containing detailed information about small molecule metabolites found in the human body. | |
Explanation | HMDB is offered to the public as a freely available resource. Use and re-distribution of the data, in whole or in part, for commercial purposes requires explicit permission of the authors and explicit acknowledgment of the source material (HMDB) and the original publication (see the HMDB citing page). We ask that users who download significant portions of the database cite the HMDB paper in any resulting publications. | |
Retrosynthesis Analysis
AI-Powered Synthesis Planning: Our tool employs the Template_relevance Pistachio, Template_relevance Bkms_metabolic, Template_relevance Pistachio_ringbreaker, Template_relevance Reaxys, Template_relevance Reaxys_biocatalysis model, leveraging a vast database of chemical reactions to predict feasible synthetic routes.
One-Step Synthesis Focus: Specifically designed for one-step synthesis, it provides concise and direct routes for your target compounds, streamlining the synthesis process.
Accurate Predictions: Utilizing the extensive PISTACHIO, BKMS_METABOLIC, PISTACHIO_RINGBREAKER, REAXYS, REAXYS_BIOCATALYSIS database, our tool offers high-accuracy predictions, reflecting the latest in chemical research and data.
Strategy Settings
Precursor scoring | Relevance Heuristic |
---|---|
Min. plausibility | 0.01 |
Model | Template_relevance |
Template Set | Pistachio/Bkms_metabolic/Pistachio_ringbreaker/Reaxys/Reaxys_biocatalysis |
Top-N result to add to graph | 6 |
Feasible Synthetic Routes
Q1: What is 15-cis-phytoene and what is its role in carotenoid biosynthesis?
A1: 15-cis-phytoene is the first colorless carotene produced in the carotenoid biosynthetic pathway. It is formed from two molecules of geranylgeranyl diphosphate (GGPP) in a reaction catalyzed by the enzyme phytoene synthase (PSY) [, ]. This step marks the beginning of the pathway that ultimately leads to the production of a variety of colored carotenoids, including β-carotene, lycopene, and xanthophylls, which play crucial roles in photosynthesis, photoprotection, and signaling in plants [, , , ].
Q2: How does the geometric isomerism of 15-cis-phytoene impact its role in the carotenoid pathway?
A2: The 15-cis configuration of phytoene is crucial for the subsequent desaturation steps in the pathway. Plant phytoene desaturase (PDS) specifically acts on 15-cis-phytoene, introducing two double bonds to form 9,15,9′-tri-cis-ζ-carotene [, , ]. This cis configuration is essential for the activity of the next enzyme in the pathway, ζ-carotene desaturase (ZDS) [, ].
Q3: What happens when phytoene desaturation is inhibited, and how does this affect 15-cis-phytoene levels?
A4: Blocking phytoene desaturation, either by genetic mutations or herbicide treatments (e.g., norflurazon), leads to the accumulation of 15-cis-phytoene and other colorless carotenoid precursors [, , , , ]. This accumulation confirms the role of 15-cis-phytoene as the initial precursor in the pathway and highlights the importance of desaturation for the formation of colored carotenoids.
Q4: Are there other isomers of phytoene besides the 15-cis form, and what is their relevance?
A5: While 15-cis-phytoene is the dominant isomer produced in plants and algae like Dunaliella salina, small amounts of all-trans-phytoene can also be detected [, ]. The ratio of these isomers can be influenced by factors like light intensity []. The accumulation of primarily 15-cis-phytoene suggests that this isomer is the preferred substrate for downstream enzymes in these organisms.
Q5: What are the potential applications of 15-cis-phytoene and how is it produced?
A6: 15-cis-phytoene has gained interest for its potential use in cosmetics as a UV protectant and skin-whitening agent []. Microalgae like Dunaliella salina, which naturally accumulate high levels of 15-cis-phytoene under specific conditions, are being explored as potential "cell factories" for its production [].
Q6: How does the study of phytoene desaturase (PDS) contribute to our understanding of 15-cis-phytoene metabolism?
A7: Investigating PDS, the enzyme responsible for the desaturation of 15-cis-phytoene, offers valuable insights into this early step in carotenoid biosynthesis. Research on PDS has revealed its requirement for plastoquinone as an electron acceptor and its susceptibility to inhibition by herbicides like norflurazon [, ]. These studies have helped elucidate the mechanism of phytoene desaturation and its importance in controlling the flow of precursors like 15-cis-phytoene through the carotenoid pathway.
Descargo de responsabilidad e información sobre productos de investigación in vitro
Tenga en cuenta que todos los artículos e información de productos presentados en BenchChem están destinados únicamente con fines informativos. Los productos disponibles para la compra en BenchChem están diseñados específicamente para estudios in vitro, que se realizan fuera de organismos vivos. Los estudios in vitro, derivados del término latino "in vidrio", involucran experimentos realizados en entornos de laboratorio controlados utilizando células o tejidos. Es importante tener en cuenta que estos productos no se clasifican como medicamentos y no han recibido la aprobación de la FDA para la prevención, tratamiento o cura de ninguna condición médica, dolencia o enfermedad. Debemos enfatizar que cualquier forma de introducción corporal de estos productos en humanos o animales está estrictamente prohibida por ley. Es esencial adherirse a estas pautas para garantizar el cumplimiento de los estándares legales y éticos en la investigación y experimentación.