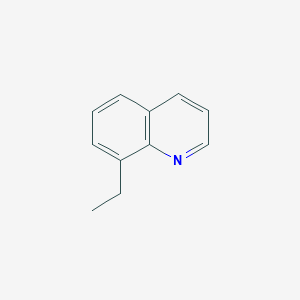
8-Etilquinolina
Descripción general
Descripción
8-Ethylquinoline is an organic compound with the molecular formula C11H11N. It is a derivative of quinoline, where an ethyl group is substituted at the 8th position of the quinoline ring. Quinoline itself is a heterocyclic aromatic organic compound with a double-ring structure consisting of a benzene ring fused to a pyridine ring. 8-Ethylquinoline is known for its applications in various fields, including medicinal chemistry, organic synthesis, and industrial processes.
Aplicaciones Científicas De Investigación
8-Ethylquinoline has a wide range of applications in scientific research:
Chemistry: It is used as a building block in the synthesis of more complex organic molecules. Its derivatives are often explored for their unique chemical properties.
Biology: 8-Ethylquinoline and its derivatives have been studied for their potential biological activities, including antimicrobial and anticancer properties.
Medicine: Quinoline derivatives, including 8-Ethylquinoline, are investigated for their potential as therapeutic agents. They are explored for their ability to interact with biological targets and pathways.
Industry: It is used in the production of dyes, agrochemicals, and other industrial chemicals. .
Mecanismo De Acción
Target of Action
8-Ethylquinoline is a derivative of quinoline, a heterocyclic aromatic organic compoundQuinoline derivatives, including 8-hydroxyquinoline, have been found to bind to a diverse range of targets with high affinities . These targets are often involved in various biological processes, contributing to the compound’s broad-ranging pharmacological potential .
Mode of Action
Quinoline derivatives have been shown to interact with their targets in a variety of ways, leading to changes in cellular functions . For instance, some quinoline derivatives have demonstrated potential efficacy through different mechanisms of action such as cell cycle arrest, apoptosis induction, angiogenesis inhibition, and cell metastasis disruption .
Biochemical Pathways
For example, they have been found to play roles in maintaining cellular homeostasis and detoxifying reactive oxygen species
Result of Action
Quinoline derivatives have been reported to exhibit a wide range of biological activities, including antimicrobial, anticancer, and antifungal effects . These effects are likely the result of the compound’s interaction with its targets and its impact on various biochemical pathways .
Análisis Bioquímico
Biochemical Properties
It is known that quinoline derivatives, such as 8-Ethylquinoline, can participate in various biochemical reactions
Cellular Effects
Quinoline derivatives have been shown to have effects on various types of cells and cellular processes . They can influence cell function, including impacts on cell signaling pathways, gene expression, and cellular metabolism
Molecular Mechanism
It is known that quinoline derivatives can exert their effects at the molecular level through various mechanisms, including binding interactions with biomolecules, enzyme inhibition or activation, and changes in gene expression
Metabolic Pathways
Quinoline derivatives are known to be involved in various metabolic pathways
Métodos De Preparación
Synthetic Routes and Reaction Conditions: The synthesis of 8-Ethylquinoline can be achieved through several methods. One common approach involves the Friedländer synthesis, which is a condensation reaction between aniline derivatives and carbonyl compounds. For 8-Ethylquinoline, the reaction typically involves the condensation of 2-aminobenzaldehyde with ethyl acetoacetate under acidic or basic conditions.
Another method is the Skraup synthesis, which involves the cyclization of aniline derivatives with glycerol and sulfuric acid in the presence of an oxidizing agent like nitrobenzene. This method can be adapted to introduce the ethyl group at the desired position.
Industrial Production Methods: Industrial production of 8-Ethylquinoline often employs catalytic processes to enhance yield and selectivity. Catalysts such as transition metals (e.g., palladium, platinum) are used to facilitate the cyclization and functionalization reactions. The use of microwave irradiation and solvent-free conditions has also been explored to make the process more environmentally friendly and efficient .
Análisis De Reacciones Químicas
Types of Reactions: 8-Ethylquinoline undergoes various chemical reactions, including:
Oxidation: It can be oxidized to form 8-Ethylquinoline N-oxide using oxidizing agents like hydrogen peroxide or peracids.
Reduction: Reduction reactions can convert 8-Ethylquinoline to its corresponding tetrahydroquinoline derivatives using reducing agents like lithium aluminum hydride.
Substitution: Electrophilic substitution reactions can introduce various functional groups into the quinoline ring. For example, nitration, sulfonation, and halogenation can be performed under appropriate conditions.
Common Reagents and Conditions:
Oxidation: Hydrogen peroxide, peracids.
Reduction: Lithium aluminum hydride, sodium borohydride.
Substitution: Nitric acid (for nitration), sulfuric acid (for sulfonation), halogens (for halogenation).
Major Products Formed:
Oxidation: 8-Ethylquinoline N-oxide.
Reduction: Tetrahydro-8-ethylquinoline.
Substitution: Various substituted quinoline derivatives depending on the reagents used
Comparación Con Compuestos Similares
Quinoline: The parent compound, which lacks the ethyl group at the 8th position.
8-Methylquinoline: Similar structure but with a methyl group instead of an ethyl group.
8-Isopropylquinoline: Contains an isopropyl group at the 8th position.
Uniqueness of 8-Ethylquinoline: 8-Ethylquinoline is unique due to the presence of the ethyl group, which can influence its chemical reactivity and biological activity. The ethyl group can affect the compound’s lipophilicity, making it more or less soluble in different solvents. This can impact its ability to interact with biological membranes and targets. Additionally, the steric and electronic effects of the ethyl group can alter the compound’s reactivity in various chemical reactions, making it a valuable compound for synthetic and medicinal chemistry .
Propiedades
IUPAC Name |
8-ethylquinoline | |
---|---|---|
Source | PubChem | |
URL | https://pubchem.ncbi.nlm.nih.gov | |
Description | Data deposited in or computed by PubChem | |
InChI |
InChI=1S/C11H11N/c1-2-9-5-3-6-10-7-4-8-12-11(9)10/h3-8H,2H2,1H3 | |
Source | PubChem | |
URL | https://pubchem.ncbi.nlm.nih.gov | |
Description | Data deposited in or computed by PubChem | |
InChI Key |
WSNATRDCOFYLCB-UHFFFAOYSA-N | |
Source | PubChem | |
URL | https://pubchem.ncbi.nlm.nih.gov | |
Description | Data deposited in or computed by PubChem | |
Canonical SMILES |
CCC1=CC=CC2=C1N=CC=C2 | |
Source | PubChem | |
URL | https://pubchem.ncbi.nlm.nih.gov | |
Description | Data deposited in or computed by PubChem | |
Molecular Formula |
C11H11N | |
Source | PubChem | |
URL | https://pubchem.ncbi.nlm.nih.gov | |
Description | Data deposited in or computed by PubChem | |
DSSTOX Substance ID |
DTXSID20173321 | |
Record name | Quinoline, 8-ethyl- | |
Source | EPA DSSTox | |
URL | https://comptox.epa.gov/dashboard/DTXSID20173321 | |
Description | DSSTox provides a high quality public chemistry resource for supporting improved predictive toxicology. | |
Molecular Weight |
157.21 g/mol | |
Source | PubChem | |
URL | https://pubchem.ncbi.nlm.nih.gov | |
Description | Data deposited in or computed by PubChem | |
CAS No. |
19655-56-2 | |
Record name | Quinoline, 8-ethyl- | |
Source | ChemIDplus | |
URL | https://pubchem.ncbi.nlm.nih.gov/substance/?source=chemidplus&sourceid=0019655562 | |
Description | ChemIDplus is a free, web search system that provides access to the structure and nomenclature authority files used for the identification of chemical substances cited in National Library of Medicine (NLM) databases, including the TOXNET system. | |
Record name | Quinoline, 8-ethyl- | |
Source | EPA DSSTox | |
URL | https://comptox.epa.gov/dashboard/DTXSID20173321 | |
Description | DSSTox provides a high quality public chemistry resource for supporting improved predictive toxicology. | |
Record name | 8-Ethylquinoline | |
Source | FDA Global Substance Registration System (GSRS) | |
URL | https://gsrs.ncats.nih.gov/ginas/app/beta/substances/AVE2CK5VM3 | |
Description | The FDA Global Substance Registration System (GSRS) enables the efficient and accurate exchange of information on what substances are in regulated products. Instead of relying on names, which vary across regulatory domains, countries, and regions, the GSRS knowledge base makes it possible for substances to be defined by standardized, scientific descriptions. | |
Explanation | Unless otherwise noted, the contents of the FDA website (www.fda.gov), both text and graphics, are not copyrighted. They are in the public domain and may be republished, reprinted and otherwise used freely by anyone without the need to obtain permission from FDA. Credit to the U.S. Food and Drug Administration as the source is appreciated but not required. | |
Synthesis routes and methods
Procedure details
Retrosynthesis Analysis
AI-Powered Synthesis Planning: Our tool employs the Template_relevance Pistachio, Template_relevance Bkms_metabolic, Template_relevance Pistachio_ringbreaker, Template_relevance Reaxys, Template_relevance Reaxys_biocatalysis model, leveraging a vast database of chemical reactions to predict feasible synthetic routes.
One-Step Synthesis Focus: Specifically designed for one-step synthesis, it provides concise and direct routes for your target compounds, streamlining the synthesis process.
Accurate Predictions: Utilizing the extensive PISTACHIO, BKMS_METABOLIC, PISTACHIO_RINGBREAKER, REAXYS, REAXYS_BIOCATALYSIS database, our tool offers high-accuracy predictions, reflecting the latest in chemical research and data.
Strategy Settings
Precursor scoring | Relevance Heuristic |
---|---|
Min. plausibility | 0.01 |
Model | Template_relevance |
Template Set | Pistachio/Bkms_metabolic/Pistachio_ringbreaker/Reaxys/Reaxys_biocatalysis |
Top-N result to add to graph | 6 |
Feasible Synthetic Routes
Q1: What is the structure of 8-ethylquinoline and how does it impact its reactivity?
A: 8-Ethylquinoline (C11H11N) is an organic compound consisting of a quinoline ring system with an ethyl substituent at the 8-position [, , ]. This structure allows for several types of reactions, including:
- Cyclometalation: The ethyl group can undergo C-H activation in the presence of transition metal complexes, forming a metallacycle where the metal coordinates to both the carbon of the ethyl group and the nitrogen of the quinoline ring. This process has been extensively studied with palladium(II) salts [, ].
- Electrophilic Aromatic Substitution: The electron-rich nature of the quinoline ring makes it susceptible to electrophilic attack, leading to the introduction of various substituents [].
- Oxidation: The nitrogen atom in the quinoline ring can be oxidized to form N-oxides, which exhibit distinct reactivity compared to the parent compound [, ].
Q2: What are some notable catalytic applications of 8-ethylquinoline derivatives?
A: 8-Ethylquinoline N-oxide has been identified as an effective oxidant in gold-catalyzed organic transformations [, ]. For example, it facilitates the synthesis of 4-(2-oxoalkoxy)butyl methanesulfonates through a unique ring-opening reaction of tetrahydrofuran (THF) []. This reaction proceeds through an α-oxo gold carbene intermediate generated from terminal alkynes in the presence of 8-ethylquinoline N-oxide and MesOH under mild conditions.
Q3: Has the stereochemistry of 8-ethylquinoline cyclometalation been investigated?
A: Yes, studies have specifically examined the stereochemistry at the carbon center during the cyclometalation of 8-ethylquinoline by palladium(II) salts []. Using a deuterium-labeled 8-ethylquinoline derivative, researchers demonstrated that the C-H activation step proceeds with retention of configuration at the carbon atom undergoing cyclometalation.
Q4: How do structural modifications of 8-ethylquinoline influence its coordination chemistry?
A: Introducing specific substituents on the 8-ethylquinoline scaffold can significantly impact its coordination behavior with transition metals []. For instance, incorporating a bromomethyl group at the 8-position enables oxidative addition reactions with palladium(II) complexes, leading to the formation of stable palladium(IV) complexes containing an intramolecular coordination system [].
Q5: What is the historical significance of 8-ethylquinoline in petroleum chemistry?
A: Historically, 8-ethylquinoline and its methylated derivatives have been identified and isolated from petroleum distillates [, , ]. This discovery highlights the presence of nitrogen-containing heterocycles in crude oil and their potential contribution to the properties of petroleum fractions.
Q6: Are there alternative synthetic routes to access alkylquinolines like 8-ethylquinoline?
A: Yes, researchers are exploring environmentally friendly approaches for the synthesis of alkylquinolines, including 8-ethylquinoline, using vapor phase reactions []. This method offers potential advantages in terms of reduced waste generation and improved sustainability compared to traditional solution-phase syntheses.
Descargo de responsabilidad e información sobre productos de investigación in vitro
Tenga en cuenta que todos los artículos e información de productos presentados en BenchChem están destinados únicamente con fines informativos. Los productos disponibles para la compra en BenchChem están diseñados específicamente para estudios in vitro, que se realizan fuera de organismos vivos. Los estudios in vitro, derivados del término latino "in vidrio", involucran experimentos realizados en entornos de laboratorio controlados utilizando células o tejidos. Es importante tener en cuenta que estos productos no se clasifican como medicamentos y no han recibido la aprobación de la FDA para la prevención, tratamiento o cura de ninguna condición médica, dolencia o enfermedad. Debemos enfatizar que cualquier forma de introducción corporal de estos productos en humanos o animales está estrictamente prohibida por ley. Es esencial adherirse a estas pautas para garantizar el cumplimiento de los estándares legales y éticos en la investigación y experimentación.