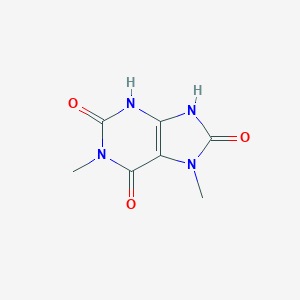
1,7-Dimethyluric acid
Descripción general
Descripción
1,7-Dimethyluric acid is a metabolite of caffeine produced by the action of xanthine oxidase on paraxanthine . It can be detected in urine as a biomarker of caffeine consumption .
Molecular Structure Analysis
The molecular formula of 1,7-Dimethyluric acid is C7H8N4O3 . The molecular weight is 196.16 g/mol .
Chemical Reactions Analysis
1,7-Dimethyluric acid is a metabolite of caffeine produced by the action of xanthine oxidase on paraxanthine . It can be detected in urine as a biomarker of caffeine consumption . Electrochemical oxidation of 1,7-dimethyluric acid has been studied over a wide pH range of 2.2-10.3 at solid electrodes .
Aplicaciones Científicas De Investigación
Chemical Synthesis
1,7-Dimethyluric acid is used as a heterocyclic building block in chemical synthesis . It’s an important compound in the field of organic chemistry, particularly in the synthesis of more complex molecules.
Metabolite of Caffeine
1,7-Dimethyluric acid is a metabolite of caffeine . It’s produced by the action of xanthine oxidase on paraxanthine and can be detected in urine as a biomarker of caffeine consumption .
Electrochemical Studies
Electrochemical oxidation of 1,7-dimethyluric acid has been studied over a wide pH range of 2.2-10.3 at solid electrodes . This research can contribute to the understanding of redox reactions and electrochemical processes.
Pharmacology
In the field of pharmacology, 1,7-Dimethyluric acid is used for the simultaneous determination of plasma levels of theophylline and its metabolites without interference from caffeine or caffeine metabolites by High-Performance Liquid Chromatography (HPLC) . This makes it a valuable tool in drug metabolism studies.
Toxicology
In toxicology, 1,7-Dimethyluric acid can be used to study the metabolism and effects of xenobiotics . It’s particularly useful in understanding how the body processes foreign substances, including drugs and toxins.
Neuroscience
In neuroscience, 1,7-Dimethyluric acid, as a metabolite of caffeine, can be used to study the effects of caffeine on the nervous system . This includes research into sleep and biological rhythms, addiction, and pain research.
Mecanismo De Acción
Target of Action
1,7-Dimethyluric acid is a metabolite of caffeine . It is produced by the action of xanthine oxidase on paraxanthine
Biochemical Pathways
1,7-Dimethyluric acid is part of the caffeine metabolism pathway . It is produced by the action of the enzyme xanthine oxidase on paraxanthine
Pharmacokinetics
Caffeine is known to be rapidly and completely absorbed from the gastrointestinal tract, distributed throughout the body, extensively metabolized by the liver, and excreted in urine .
Result of Action
As a metabolite of caffeine, its effects may be related to those of caffeine, which include central nervous system stimulation, diuresis, and relaxation of smooth muscles .
Propiedades
IUPAC Name |
1,7-dimethyl-3,9-dihydropurine-2,6,8-trione | |
---|---|---|
Source | PubChem | |
URL | https://pubchem.ncbi.nlm.nih.gov | |
Description | Data deposited in or computed by PubChem | |
InChI |
InChI=1S/C7H8N4O3/c1-10-3-4(8-6(10)13)9-7(14)11(2)5(3)12/h1-2H3,(H,8,13)(H,9,14) | |
Source | PubChem | |
URL | https://pubchem.ncbi.nlm.nih.gov | |
Description | Data deposited in or computed by PubChem | |
InChI Key |
NOFNCLGCUJJPKU-UHFFFAOYSA-N | |
Source | PubChem | |
URL | https://pubchem.ncbi.nlm.nih.gov | |
Description | Data deposited in or computed by PubChem | |
Canonical SMILES |
CN1C2=C(NC1=O)NC(=O)N(C2=O)C | |
Source | PubChem | |
URL | https://pubchem.ncbi.nlm.nih.gov | |
Description | Data deposited in or computed by PubChem | |
Molecular Formula |
C7H8N4O3 | |
Source | PubChem | |
URL | https://pubchem.ncbi.nlm.nih.gov | |
Description | Data deposited in or computed by PubChem | |
DSSTOX Substance ID |
DTXSID30187499 | |
Record name | 1,7-Dimethyluric acid | |
Source | EPA DSSTox | |
URL | https://comptox.epa.gov/dashboard/DTXSID30187499 | |
Description | DSSTox provides a high quality public chemistry resource for supporting improved predictive toxicology. | |
Molecular Weight |
196.16 g/mol | |
Source | PubChem | |
URL | https://pubchem.ncbi.nlm.nih.gov | |
Description | Data deposited in or computed by PubChem | |
Physical Description |
Solid | |
Record name | 1,7-Dimethyluric acid | |
Source | Human Metabolome Database (HMDB) | |
URL | http://www.hmdb.ca/metabolites/HMDB0011103 | |
Description | The Human Metabolome Database (HMDB) is a freely available electronic database containing detailed information about small molecule metabolites found in the human body. | |
Explanation | HMDB is offered to the public as a freely available resource. Use and re-distribution of the data, in whole or in part, for commercial purposes requires explicit permission of the authors and explicit acknowledgment of the source material (HMDB) and the original publication (see the HMDB citing page). We ask that users who download significant portions of the database cite the HMDB paper in any resulting publications. | |
CAS RN |
33868-03-0 | |
Record name | 1,7-Dimethyluric acid | |
Source | CAS Common Chemistry | |
URL | https://commonchemistry.cas.org/detail?cas_rn=33868-03-0 | |
Description | CAS Common Chemistry is an open community resource for accessing chemical information. Nearly 500,000 chemical substances from CAS REGISTRY cover areas of community interest, including common and frequently regulated chemicals, and those relevant to high school and undergraduate chemistry classes. This chemical information, curated by our expert scientists, is provided in alignment with our mission as a division of the American Chemical Society. | |
Explanation | The data from CAS Common Chemistry is provided under a CC-BY-NC 4.0 license, unless otherwise stated. | |
Record name | 1,7-Dimethyluric acid | |
Source | ChemIDplus | |
URL | https://pubchem.ncbi.nlm.nih.gov/substance/?source=chemidplus&sourceid=0033868030 | |
Description | ChemIDplus is a free, web search system that provides access to the structure and nomenclature authority files used for the identification of chemical substances cited in National Library of Medicine (NLM) databases, including the TOXNET system. | |
Record name | 1,7-Dimethyluric acid | |
Source | EPA DSSTox | |
URL | https://comptox.epa.gov/dashboard/DTXSID30187499 | |
Description | DSSTox provides a high quality public chemistry resource for supporting improved predictive toxicology. | |
Record name | 7,9-dihydro-1,7-dimethyl-1H-purine-2,6,8(3H)-trione | |
Source | European Chemicals Agency (ECHA) | |
URL | https://echa.europa.eu/substance-information/-/substanceinfo/100.046.991 | |
Description | The European Chemicals Agency (ECHA) is an agency of the European Union which is the driving force among regulatory authorities in implementing the EU's groundbreaking chemicals legislation for the benefit of human health and the environment as well as for innovation and competitiveness. | |
Explanation | Use of the information, documents and data from the ECHA website is subject to the terms and conditions of this Legal Notice, and subject to other binding limitations provided for under applicable law, the information, documents and data made available on the ECHA website may be reproduced, distributed and/or used, totally or in part, for non-commercial purposes provided that ECHA is acknowledged as the source: "Source: European Chemicals Agency, http://echa.europa.eu/". Such acknowledgement must be included in each copy of the material. ECHA permits and encourages organisations and individuals to create links to the ECHA website under the following cumulative conditions: Links can only be made to webpages that provide a link to the Legal Notice page. | |
Record name | 1,7-DIMETHYLURIC ACID | |
Source | FDA Global Substance Registration System (GSRS) | |
URL | https://gsrs.ncats.nih.gov/ginas/app/beta/substances/Z633Z7KAJU | |
Description | The FDA Global Substance Registration System (GSRS) enables the efficient and accurate exchange of information on what substances are in regulated products. Instead of relying on names, which vary across regulatory domains, countries, and regions, the GSRS knowledge base makes it possible for substances to be defined by standardized, scientific descriptions. | |
Explanation | Unless otherwise noted, the contents of the FDA website (www.fda.gov), both text and graphics, are not copyrighted. They are in the public domain and may be republished, reprinted and otherwise used freely by anyone without the need to obtain permission from FDA. Credit to the U.S. Food and Drug Administration as the source is appreciated but not required. | |
Record name | 1,7-Dimethyluric acid | |
Source | Human Metabolome Database (HMDB) | |
URL | http://www.hmdb.ca/metabolites/HMDB0011103 | |
Description | The Human Metabolome Database (HMDB) is a freely available electronic database containing detailed information about small molecule metabolites found in the human body. | |
Explanation | HMDB is offered to the public as a freely available resource. Use and re-distribution of the data, in whole or in part, for commercial purposes requires explicit permission of the authors and explicit acknowledgment of the source material (HMDB) and the original publication (see the HMDB citing page). We ask that users who download significant portions of the database cite the HMDB paper in any resulting publications. | |
Retrosynthesis Analysis
AI-Powered Synthesis Planning: Our tool employs the Template_relevance Pistachio, Template_relevance Bkms_metabolic, Template_relevance Pistachio_ringbreaker, Template_relevance Reaxys, Template_relevance Reaxys_biocatalysis model, leveraging a vast database of chemical reactions to predict feasible synthetic routes.
One-Step Synthesis Focus: Specifically designed for one-step synthesis, it provides concise and direct routes for your target compounds, streamlining the synthesis process.
Accurate Predictions: Utilizing the extensive PISTACHIO, BKMS_METABOLIC, PISTACHIO_RINGBREAKER, REAXYS, REAXYS_BIOCATALYSIS database, our tool offers high-accuracy predictions, reflecting the latest in chemical research and data.
Strategy Settings
Precursor scoring | Relevance Heuristic |
---|---|
Min. plausibility | 0.01 |
Model | Template_relevance |
Template Set | Pistachio/Bkms_metabolic/Pistachio_ringbreaker/Reaxys/Reaxys_biocatalysis |
Top-N result to add to graph | 6 |
Feasible Synthetic Routes
Q & A
Q1: What is the origin of 1,7-Dimethyluric acid in the human body?
A1: 1,7-Dimethyluric acid is a primary metabolite of caffeine in humans. [, , , , ] It is formed through the 8-hydroxylation of 1,7-Dimethylxanthine (paraxanthine), another major caffeine metabolite. []
Q2: Which enzymes are primarily involved in the metabolic pathway leading to 1,7-Dimethyluric acid formation?
A2: Three main enzymes are involved:
Cytochrome P450 1A2 (CYP1A2): Catalyzes the initial 3-demethylation of caffeine to paraxanthine. [, , , ] * Cytochrome P450 2A6 (CYP2A6): Primarily responsible for the 8-hydroxylation of paraxanthine to 1,7-Dimethyluric acid. []* N-acetyltransferase 2 (NAT2):* Involved in a parallel pathway of caffeine metabolism, influencing the relative levels of certain metabolites used to assess CYP1A2 and CYP2A6 activity. [, , , ]
Q3: Can the levels of 1,7-Dimethyluric acid in urine be used to assess enzyme activity?
A3: Yes, urinary ratios of 1,7-Dimethyluric acid to other caffeine metabolites are used as non-invasive markers for the in vivo activity of CYP1A2 and CYP2A6. [, , , , ]
Q4: Which specific urinary metabolite ratios are used to estimate CYP1A2 and CYP2A6 activity?
A4:
CYP1A2: (1,7-Dimethylxanthine + 1,7-Dimethyluric acid) / Caffeine, [, ] or (5-Acetylamino-6-formylamino-3-methyluracil + 1-Methyluric acid + 1-Methylxanthine) / 1,7-Dimethyluric acid. [, ]* CYP2A6:* 1,7-Dimethyluric acid / 1,7-Dimethylxanthine [, ] or 1,7-Dimethyluric acid / 1-Methylxanthine. [, ]
Q5: Why are multiple ratios used for assessing CYP1A2 and CYP2A6 activity?
A5: Different ratios offer varying levels of sensitivity and reliability depending on factors like:* Time of urine collection (spot vs. 24-hour). [, ]* Controlled vs. uncontrolled caffeine intake. [, ] * The presence of interfering substances or medications. [, ]
Q6: What is the molecular formula and weight of 1,7-Dimethyluric acid?
A8: Its molecular formula is C7H8N4O3, and its molecular weight is 196.16 g/mol. []
Q7: What analytical methods are used to quantify 1,7-Dimethyluric acid in biological samples?
A9: High-performance liquid chromatography (HPLC) coupled with various detection methods, such as UV-visible absorbance and tandem mass spectrometry (MS/MS), is commonly used. [, , , , , ]
Q8: Are there specific challenges in analyzing 1,7-Dimethyluric acid?
A10:
* Achieving adequate separation from other caffeine metabolites. [, ]* Sensitivity limitations of some detection methods, particularly for low metabolite concentrations. []* Interference from co-eluting compounds in complex biological matrices. []
Q9: Is 1,7-Dimethyluric acid found in the environment?
A13: Yes, 1,7-Dimethyluric acid has been detected in municipal wastewater, likely originating from human caffeine consumption. []
Q10: What are the implications of finding 1,7-Dimethyluric acid in wastewater?
A14: Its presence can be used to estimate population-level caffeine consumption. [] This information can contribute to understanding caffeine consumption patterns and their potential impacts on public health and the environment.
Descargo de responsabilidad e información sobre productos de investigación in vitro
Tenga en cuenta que todos los artículos e información de productos presentados en BenchChem están destinados únicamente con fines informativos. Los productos disponibles para la compra en BenchChem están diseñados específicamente para estudios in vitro, que se realizan fuera de organismos vivos. Los estudios in vitro, derivados del término latino "in vidrio", involucran experimentos realizados en entornos de laboratorio controlados utilizando células o tejidos. Es importante tener en cuenta que estos productos no se clasifican como medicamentos y no han recibido la aprobación de la FDA para la prevención, tratamiento o cura de ninguna condición médica, dolencia o enfermedad. Debemos enfatizar que cualquier forma de introducción corporal de estos productos en humanos o animales está estrictamente prohibida por ley. Es esencial adherirse a estas pautas para garantizar el cumplimiento de los estándares legales y éticos en la investigación y experimentación.