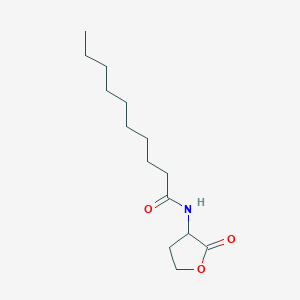
N-Decanoyl-DL-homoserine lactone
Descripción general
Descripción
N-Decanoyl-DL-homoserine lactone (C10-HSL) is a medium-chain acyl-homoserine lactone (AHL) quorum sensing (QS) molecule produced by diverse gram-negative bacteria, including Acinetobacter spp., Citrobacter freundii, and Methylobacterium species . Structurally, it consists of a 10-carbon acyl chain (decanoyl) linked to a homoserine lactone ring (molecular formula: C₁₄H₂₅NO₃; molecular weight: 255.35 g/mol) . C10-HSL is widely utilized as a reference standard in AHL detection assays due to its stability and moderate chain length, which allows efficient diffusion through bacterial membranes .
Métodos De Preparación
Chemical Synthesis of N-Decanoyl-DL-Homoserine Lactone
Carbodiimide-Mediated Coupling
The most widely adopted method for synthesizing C10-HSL involves carbodiimide-mediated coupling between decanoic acid and DL-homoserine lactone. As detailed in recent work, this approach employs N-(3-dimethylaminopropyl)-N′-ethylcarbodiimide (EDC) as a coupling agent and 4-dimethylaminopyridine (DMAP) as a catalyst .
Reaction Procedure :
-
Reagent Preparation : Decanoic acid (2a, 0.28 g, 1.65 mmol) is dissolved in anhydrous dichloromethane (DCM, 10 mL).
-
Activation : EDC (0.29 mL, 1.65 mmol) and DMAP (0.08 g, 0.66 mmol) are added sequentially under nitrogen atmosphere.
-
Coupling : DL-homoserine lactone (HSL, 0.20 g, 1.10 mmol) is introduced, and the mixture is stirred at room temperature for 24 hours.
-
Workup : The reaction is quenched with 5% HCl, and the organic layer is extracted with DCM (3 × 10 mL), dried over MgSO₄, and concentrated in vacuo.
Key Considerations :
-
Solvent Choice : DCM is preferred for its ability to dissolve both fatty acids and HSL while minimizing side reactions .
-
Catalyst Role : DMAP accelerates the formation of the reactive O-acylisourea intermediate, enhancing coupling efficiency .
Enzymatic Synthesis Using Bacterial Systems
LuxI Homologue-Mediated Biosynthesis
In Vibrio fischeri and related species, LuxI homologues catalyze the synthesis of AHLs via a two-step process: (i) acyl-acyl carrier protein (acyl-ACP) formation and (ii) lactonization of homoserine . While this method is less commonly used for C10-HSL, it offers stereochemical specificity.
Procedure :
-
Acyl-ACP Generation : Decanoyl-CoA is transferred to ACP via acyltransferase activity.
-
Lactone Formation : LuxI homologues condense decanoyl-ACP with S-adenosylmethionine (SAM)-derived homoserine lactone .
Limitations :
-
Yield : Enzymatic synthesis typically yields <10% due to substrate specificity and competing metabolic pathways .
-
Scalability : Requires optimized bacterial cultures and purification from complex biological matrices .
Purification and Isolation Techniques
Silica Gel Column Chromatography
Crude C10-HSL is purified using silica gel chromatography with dichloromethane/ethyl acetate (19:1 v/v) as the eluent . This method achieves >95% purity, as confirmed by thin-layer chromatography (TLC) .
Optimization Insights :
-
Eluent Polarity : A 19:1 DCM/EtOAc ratio balances resolution and run time, separating C10-HSL from unreacted decanoic acid and HSL .
-
Load Capacity : Up to 500 mg of crude product can be processed on a 40 cm × 2 cm column without peak broadening .
Recrystallization
Alternative purification involves recrystallization from cold ethyl acetate/hexane (1:3 v/v), yielding needle-like crystals with 99% purity .
Analytical Characterization
Spectroscopic Validation
Nuclear Magnetic Resonance (NMR) :
-
¹H NMR (100 MHz, CDCl₃) : δ 6.06 (1H, s, -NH-), 2.20–2.04 (4H, m, -CH₂-), 0.96 (3H, t, J = 7.2 Hz, terminal -CH₃) .
-
¹³C NMR (26 MHz, CDCl₃) : δ 175.57 (C=O, lactone), 173.50 (C=O, amide), 22.41 (-CH₂-), 13.35 (-CH₃) .
Infrared Spectroscopy (IR) :
-
Peaks at 3315 cm⁻¹ (-NH stretch), 1775 cm⁻¹ (lactone C=O), and 1643 cm⁻¹ (amide C=O) confirm structural integrity .
High-Resolution Mass Spectrometry (HRMS)
Comparative Analysis of Synthesis Routes
Parameter | Chemical Synthesis | Enzymatic Synthesis |
---|---|---|
Yield | 59–77% | <10% |
Purity | >95% | 70–80% |
Reaction Time | 24 hours | 48–72 hours |
Stereoselectivity | Racemic (DL) | L-specific |
Advantages of Chemical Synthesis :
Análisis De Reacciones Químicas
Types of Reactions: N-Decanoyl-DL-homoserine lactone undergoes various chemical reactions, including:
Oxidation: This compound can be oxidized to form this compound oxides.
Reduction: Reduction reactions can convert it into corresponding alcohols.
Substitution: It can undergo nucleophilic substitution reactions where the lactone ring is opened and substituted with different nucleophiles.
Common Reagents and Conditions:
Oxidation: Common oxidizing agents include potassium permanganate (KMnO4) and hydrogen peroxide (H2O2).
Reduction: Reducing agents such as lithium aluminum hydride (LiAlH4) or sodium borohydride (NaBH4) are used.
Substitution: Nucleophiles like amines or thiols can be used under basic conditions.
Major Products:
Oxidation: this compound oxides.
Reduction: Corresponding alcohols.
Substitution: Various substituted lactones depending on the nucleophile used.
Aplicaciones Científicas De Investigación
Quorum Sensing and Microbial Communication
Quorum Sensing Mechanism:
N-C10-HSL is primarily recognized for its role in quorum sensing, a process that allows bacteria to communicate and coordinate behavior based on population density. This mechanism is pivotal in regulating virulence factors, biofilm formation, and bioluminescence in various bacterial species.
- Virulence Regulation: In pathogenic bacteria, AHLs like N-C10-HSL can regulate the expression of virulence genes. For instance, studies have shown that the presence of N-C10-HSL enhances the pathogenicity of certain strains by upregulating exoproteases and other virulence factors .
- Biofilm Formation: N-C10-HSL has been implicated in biofilm development, which is critical for bacterial survival in hostile environments. Biofilms provide protection against antibiotics and the host immune system. Research indicates that N-C10-HSL can stimulate biofilm formation in various bacterial species such as Pseudomonas aeruginosa and Escherichia coli .
Environmental Applications
Bioremediation:
N-C10-HSL has potential applications in bioremediation processes. By enhancing microbial communication and cooperation, it can improve the degradation of pollutants in contaminated environments.
- Case Study: In a study involving the treatment of wastewater containing pentachlorophenol (PCP), the addition of N-C10-HSL improved the dechlorination efficiency of anaerobic bacteria by facilitating interspecies communication within biofilms . This led to a significant increase in chemical oxygen demand (COD) removal rates.
Industrial Biotechnology
Bioprocess Optimization:
In industrial settings, N-C10-HSL can be used to optimize fermentation processes by modulating microbial behavior.
- Example: In the production of enzymes or metabolites, adding N-C10-HSL can enhance yield by promoting cell growth and metabolic activity through improved quorum sensing mechanisms . This application is particularly relevant in industries focused on enzyme production for food processing or biocatalysis.
Medical Applications
Infection Control:
Given its role in regulating virulence factors, N-C10-HSL has potential applications in developing strategies to control infections caused by pathogenic bacteria.
- Antimicrobial Strategies: Research indicates that compounds interfering with AHL signaling pathways could serve as novel antimicrobial agents. For instance, furanones have been shown to inhibit AHL-mediated biofilm formation, thus reducing infection rates associated with biofilms .
Mecanismo De Acción
N-Decanoyl-DL-homoserine lactone exerts its effects through quorum sensing. It diffuses across bacterial cell membranes and binds to specific receptor proteins inside the cell. This binding activates the receptor, which then regulates the expression of target genes involved in various cellular processes such as virulence, biofilm formation, and antibiotic resistance. The molecular targets include LuxR-type receptors and the pathways involved are those regulating gene expression in response to cell density .
Comparación Con Compuestos Similares
Structural and Functional Comparison with Similar AHLs
AHLs vary in acyl chain length (C4–C14) and substitutions (e.g., 3-oxo or 3-hydroxy groups), which critically influence their biological activity and receptor specificity. Below is a comparative analysis of C10-HSL with key analogs:
Key Findings:
Chain Length and Cytotoxicity :
- Shorter AHLs (C4–C6) generally lack cytotoxicity, while longer chains (C10–C12) exhibit dose-dependent toxicity. For example, C10-HSL reduces viability in macrophages (BMDMs) and epithelial cells (HEp-2, A549) at 50 µM, unlike C6-HSL or C4-HSL .
- OH-dDHL (C12-HSL) triggers ER- and mitochondria-mediated apoptosis, demonstrating that chain length and substitutions amplify cytotoxic effects .
Functional Roles in Bacterial Behavior :
- Biofilm Regulation : C10-HSL is a dominant AHL in anaerobic membrane bioreactors (AnMBRs), alongside C6-HSL and C8-HSL, suggesting its role in biofilm maturation .
- Gene Regulation : C10-HSL enhances ftsQ2p transcription in E. coli, while C6-HSL upregulates acid resistance genes (gadA) .
Ecological Significance :
- In marine and plant-associated bacteria (e.g., Citrobacter freundii), C10-HSL is co-produced with C8-HSL, indicating its role in phycosphere carbon cycling and interspecies signaling .
Detection and Stability Profiles
C10-HSL is detectable by biosensor strains like Agrobacterium tumefaciens NT1 (responsive to C6–C12 AHLs) . Its moderate chain length balances solubility and membrane permeability, unlike longer AHLs (e.g., C12-HSL), which may aggregate, or shorter ones (C4-HSL), which diffuse rapidly but degrade faster .
Actividad Biológica
N-Decanoyl-DL-homoserine lactone (C10-HSL) is a member of the acyl-homoserine lactones (AHLs), which are crucial signaling molecules in bacterial quorum sensing. These compounds play significant roles in regulating various biological processes, including biofilm formation, virulence factor production, and intercellular communication among bacteria. This article focuses on the biological activities of C10-HSL, exploring its effects on both bacterial and eukaryotic systems.
Structure and Synthesis
C10-HSL consists of a homoserine lactone ring linked to a decanoyl side chain. The general structure of AHLs includes a conserved lactone ring and an acyl chain, which can vary in length and saturation. The specific structure of C10-HSL influences its biological activity, particularly in relation to its interactions with various receptors and signaling pathways.
1. Quorum Sensing and Intercellular Communication
C10-HSL is primarily recognized for its role in bacterial quorum sensing. In a study involving Agrobacterium tumefaciens, it was shown that C10-HSL effectively induced transcription of tra genes, which are essential for conjugative transfer of plasmids, demonstrating its importance in bacterial communication and genetic exchange .
2. Effects on Plant Systems
Research has indicated that C10-HSL can influence plant growth and development. In Vigna radiata (mung bean), C10-HSL promoted adventitious root formation and the expression of auxin-response genes. This effect is mediated through the generation of reactive oxygen species (ROS) such as hydrogen peroxide (H2O2) and nitric oxide (NO), which are vital for root development .
3. Impact on Immune Responses
C10-HSL has been shown to modulate immune responses in mammalian cells. It acts as a chemoattractant for neutrophils, enhancing their migration in a dose-dependent manner. This activity is linked to actin remodeling and calcium mobilization within these immune cells . Furthermore, C10-HSL has demonstrated anti-inflammatory properties by reducing the secretion of pro-inflammatory cytokines like TNF-α while increasing anti-inflammatory cytokines such as IL-10 in macrophages .
Case Study 1: Root Development in Mung Beans
In an experimental setup, mung bean explants treated with C10-HSL exhibited a significant increase in adventitious root formation compared to controls. The study highlighted that the signaling pathways activated by C10-HSL involve both H2O2 and NO, suggesting a complex interplay between these molecules during root development .
Case Study 2: Neutrophil Response Modulation
A study investigating the effects of C10-HSL on neutrophils revealed that this compound enhances their migratory response towards sites of inflammation. The mechanism was linked to changes in cytoskeletal dynamics and intracellular calcium levels, emphasizing the potential role of AHLs in modulating immune cell behavior .
Research Findings Summary
The following table summarizes key research findings related to the biological activity of this compound:
Q & A
Basic Research Questions
Q. What are the optimal storage and handling protocols for C10-HSL in experimental settings?
C10-HSL should be stored as a powder at -20°C (stable for 3 years) or 4°C (2 years). When dissolved in solvents (e.g., DMSO), store at -80°C (6 months) or -20°C (1 month). Avoid repeated freeze-thaw cycles to prevent lactone ring hydrolysis. For safety, use chemical-resistant gloves, safety goggles, and NIOSH-approved respiratory protection during handling .
Q. How can researchers prepare stable stock solutions of C10-HSL for bacterial quorum-sensing studies?
Dissolve C10-HSL in inert solvents like DMSO or dimethylformamide (solubility ≈30 mg/mL). Avoid primary alcohols (e.g., ethanol) to prevent lactone ring degradation. For aqueous solutions (e.g., PBS, pH 7.2), directly dissolve the compound (solubility ≈10 mg/mL), but use within 24 hours to minimize hydrolysis .
Q. What methodologies enable sensitive detection of C10-HSL in bacterial cultures?
Surface-enhanced Raman spectroscopy (SERS) detects C10-HSL at sub-nanomolar concentrations, offering high sensitivity for low-abundance QS signal quantification. Alternative methods include HPLC with UV/FLD detection or biosensor assays using Agrobacterium tumefaciens NTL4(pZLR4) strains .
Q. How is C10-HSL implicated in Gram-negative bacterial quorum sensing?
C10-HSL binds to LuxR-type transcriptional regulators, activating virulence gene expression (e.g., proteases, siderophores) in species like Aeromonas sobria. Dose-response studies require titration (e.g., 1–100 µM) in culture media, with activity validated via β-galactosidase reporter assays .
Advanced Research Questions
Q. How do structural modifications of C10-HSL influence its stability and bioactivity?
The decanoyl chain length and lactone ring are critical for receptor binding. Enzymatic hydrolysis by evolved lactonases (e.g., GKL E101G/R230C mutant) disrupts QS by cleaving the lactone ring, reducing kcat/KM by 32-fold for C10-HSL compared to wild-type enzymes .
Q. What experimental strategies address contradictory effects of C10-HSL across bacterial species?
In Fibrobacter succinogenes, C10-HSL inhibits growth (IC50 ≈10 µM) and cellulase activity, contradicting its role as a QS activator in pathogens. Use RNA-seq to compare transcriptional profiles and identify species-specific regulatory networks .
Q. How can researchers model C10-HSL’s role in host-pathogen interactions?
C10-HSL induces apoptosis in bone marrow-derived macrophages via ER/mitochondrial pathways, evidenced by caspase-3/9 activation and cytochrome c release. Validate using flow cytometry (Annexin V/PI staining) and immunoblotting for PARP cleavage .
Q. What methodologies quantify C10-HSL’s cross-kingdom signaling in plant systems?
C10-HSL primes jasmonic acid (JA) signaling in Arabidopsis thaliana, enhancing resistance to Botrytis cinerea. Use mutant lines (e.g., coi1-1) and LC-MS/MS to measure JA-Ile accumulation post-treatment (e.g., 10 µM C10-HSL root exposure) .
Q. How does C10-HSL modulate biofilm dynamics in estuarine bacteria under salinity stress?
In brackish environments (salinity 5–25), C10-HSL (1–50 µM) upregulates biofilm-associated genes (e.g., algD) in Vibrio spp. via LuxR homologs. Confocal microscopy with SYTO9/propidium iodide quantifies biofilm biomass under varying ionic conditions .
Q. Methodological Notes
- Dosage Conversion : For in vivo studies, adjust doses using body surface area (BSA) normalization. Example: A murine dose of 20 mg/kg converts to 10 mg/kg in rats (BSA Km factors: 3 for mice, 6 for rats) .
- EPR Spectroscopy : To study Mn<sup>2+</sup>-C10-HSL interactions, reconstitute enzymes with Mn<sup>2+</sup> and analyze hyperfine splitting (≈8.3 mT) at g ≈2.0 to identify mixed-valence Mn clusters .
Propiedades
IUPAC Name |
N-(2-oxooxolan-3-yl)decanamide | |
---|---|---|
Source | PubChem | |
URL | https://pubchem.ncbi.nlm.nih.gov | |
Description | Data deposited in or computed by PubChem | |
InChI |
InChI=1S/C14H25NO3/c1-2-3-4-5-6-7-8-9-13(16)15-12-10-11-18-14(12)17/h12H,2-11H2,1H3,(H,15,16) | |
Source | PubChem | |
URL | https://pubchem.ncbi.nlm.nih.gov | |
Description | Data deposited in or computed by PubChem | |
InChI Key |
TZWZKDULKILUPV-UHFFFAOYSA-N | |
Source | PubChem | |
URL | https://pubchem.ncbi.nlm.nih.gov | |
Description | Data deposited in or computed by PubChem | |
Canonical SMILES |
CCCCCCCCCC(=O)NC1CCOC1=O | |
Source | PubChem | |
URL | https://pubchem.ncbi.nlm.nih.gov | |
Description | Data deposited in or computed by PubChem | |
Molecular Formula |
C14H25NO3 | |
Source | PubChem | |
URL | https://pubchem.ncbi.nlm.nih.gov | |
Description | Data deposited in or computed by PubChem | |
DSSTOX Substance ID |
DTXSID30469949 | |
Record name | N-Decanoyl-DL-homoserine lactone | |
Source | EPA DSSTox | |
URL | https://comptox.epa.gov/dashboard/DTXSID30469949 | |
Description | DSSTox provides a high quality public chemistry resource for supporting improved predictive toxicology. | |
Molecular Weight |
255.35 g/mol | |
Source | PubChem | |
URL | https://pubchem.ncbi.nlm.nih.gov | |
Description | Data deposited in or computed by PubChem | |
CAS No. |
106983-36-2 | |
Record name | N-Decanoyl-DL-homoserine lactone | |
Source | EPA DSSTox | |
URL | https://comptox.epa.gov/dashboard/DTXSID30469949 | |
Description | DSSTox provides a high quality public chemistry resource for supporting improved predictive toxicology. | |
Retrosynthesis Analysis
AI-Powered Synthesis Planning: Our tool employs the Template_relevance Pistachio, Template_relevance Bkms_metabolic, Template_relevance Pistachio_ringbreaker, Template_relevance Reaxys, Template_relevance Reaxys_biocatalysis model, leveraging a vast database of chemical reactions to predict feasible synthetic routes.
One-Step Synthesis Focus: Specifically designed for one-step synthesis, it provides concise and direct routes for your target compounds, streamlining the synthesis process.
Accurate Predictions: Utilizing the extensive PISTACHIO, BKMS_METABOLIC, PISTACHIO_RINGBREAKER, REAXYS, REAXYS_BIOCATALYSIS database, our tool offers high-accuracy predictions, reflecting the latest in chemical research and data.
Strategy Settings
Precursor scoring | Relevance Heuristic |
---|---|
Min. plausibility | 0.01 |
Model | Template_relevance |
Template Set | Pistachio/Bkms_metabolic/Pistachio_ringbreaker/Reaxys/Reaxys_biocatalysis |
Top-N result to add to graph | 6 |
Feasible Synthetic Routes
Descargo de responsabilidad e información sobre productos de investigación in vitro
Tenga en cuenta que todos los artículos e información de productos presentados en BenchChem están destinados únicamente con fines informativos. Los productos disponibles para la compra en BenchChem están diseñados específicamente para estudios in vitro, que se realizan fuera de organismos vivos. Los estudios in vitro, derivados del término latino "in vidrio", involucran experimentos realizados en entornos de laboratorio controlados utilizando células o tejidos. Es importante tener en cuenta que estos productos no se clasifican como medicamentos y no han recibido la aprobación de la FDA para la prevención, tratamiento o cura de ninguna condición médica, dolencia o enfermedad. Debemos enfatizar que cualquier forma de introducción corporal de estos productos en humanos o animales está estrictamente prohibida por ley. Es esencial adherirse a estas pautas para garantizar el cumplimiento de los estándares legales y éticos en la investigación y experimentación.