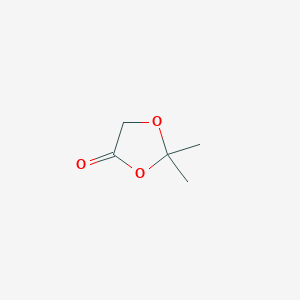
2,2-Dimethyl-1,3-dioxolan-4-one
Descripción general
Descripción
2,2-Dimethyl-1,3-dioxolan-4-one is a cyclic carbonate derivative characterized by a five-membered 1,3-dioxolane ring with methyl substituents at the 2-position and a ketone group at the 4-position. This compound serves as a versatile intermediate in organic synthesis, particularly in the preparation of esters, polymers, and electrolytes. Its structural rigidity and functional groups enable applications in materials science, such as in thermotropic liquid crystals and sustainable electrolytes for energy storage devices .
Key properties include:
- Thermal behavior: Esters of this compound, such as (2,2-dimethyl-1,3-dioxolan-4-yl)methyl palmitate, exhibit thermotropic liquid-crystalline mesophases, with melting points between 30–55°C and mesophase transitions indicative of smectic-like ordering .
- Synthetic utility: It is synthesized via acetalization reactions, often catalyzed by acid-activated clays or transition metal complexes, yielding high selectivity under mild conditions .
Mecanismo De Acción
Target of Action
2,2-Dimethyl-1,3-dioxolan-4-one is a chemical compound that is primarily used in the synthesis of various N,N-disubstituted amides of glycolic acid . These amides can have a wide range of applications, depending on their specific structures and properties .
Mode of Action
The compound is prepared via a condensation reaction between glycolic acid and acetone in the presence of P2O5 in diethyl ether . It can then be used in the synthesis of various N,N-disubstituted amides of glycolic acid, such as N,N-diethyl-2-hydroxyacetamide, N,N-di(n-butyl)-2-hydroxyacetamide, N,N-di(n-hexyl)-2-hydroxyacetamide, 4-hydroxyacetylpiperidine, 4-hydroxyacetylmorpholine, and N-ethyl-N-phenyl-2-hydroxyacetamide .
Pharmacokinetics
Its physical properties such as boiling point (41 °c) and density (0989 g/mL at 25 °C) have been reported .
Result of Action
The result of the action of this compound is the synthesis of various N,N-disubstituted amides of glycolic acid . These amides can have a wide range of applications, depending on their specific structures and properties .
Action Environment
The action of this compound is influenced by environmental factors such as temperature and the presence of other chemicals. For example, its synthesis requires the presence of P2O5 in diethyl ether . Furthermore, the compound’s stability and efficacy can be affected by factors such as pH and the presence of other chemicals in the environment .
Actividad Biológica
2,2-Dimethyl-1,3-dioxolan-4-one is a cyclic organic compound that has garnered interest in various fields of research due to its potential biological activities. This article presents a comprehensive overview of the biological properties of this compound, including its mechanisms of action, therapeutic applications, and relevant case studies.
Chemical Structure and Properties
This compound features a dioxolane ring structure with two methyl groups on the second carbon. This structural configuration contributes to its chemical reactivity and biological activity.
Biological Activity Overview
The biological activities of this compound can be categorized into several key areas:
1. Antioxidant Activity
Research indicates that derivatives of this compound exhibit significant antioxidant properties. These compounds can scavenge reactive oxygen species (ROS), thereby protecting cells from oxidative stress. For instance, studies have shown that certain derivatives can inhibit the production of pro-inflammatory cytokines such as TNF-α and NF-κB in animal models of inflammation .
2. Anti-inflammatory Effects
A notable study investigated the effects of Se-[(2,2-dimethyl-1,3-dioxolan-4-yl)methyl] 4-chlorobenzoselenolate (Se-DMC) in a model of rheumatoid arthritis. The findings demonstrated that Se-DMC significantly reduced paw edema and inflammatory markers when compared to controls. Histopathological analyses revealed decreased lymphatic vessel dilation and overall inflammation in treated mice .
3. Antitumor Activity
The compound has shown promise in cancer research, particularly in inhibiting the growth of leukemia cells. In vitro assays indicated that this compound derivatives could inhibit cell proliferation at low concentrations . The mechanism appears to involve apoptosis induction and cell cycle arrest.
The mechanisms underlying the biological activities of this compound are multifaceted:
Antioxidant Mechanism:
The antioxidant activity is primarily attributed to the inhibition of ROS production and enhancement of endogenous antioxidant defenses like superoxide dismutase (SOD) activity .
Anti-inflammatory Mechanism:
The anti-inflammatory effects are mediated through the inhibition of key inflammatory pathways involving NF-κB and TNF-α signaling. This modulation leads to reduced expression of pro-inflammatory mediators .
Case Study 1: Anti-inflammatory Effects in Arthritis
In a controlled study using a complete Freund's adjuvant (CFA)-induced arthritis model, Se-DMC was administered at 5 mg/kg for 14 days. Results showed a significant reduction in paw edema and pain sensitivity compared to meloxicam treatment. The compound also restored SOD activity levels altered by CFA .
Case Study 2: Antitumor Activity in Leukemia
In vitro studies demonstrated that derivatives of this compound could inhibit L1210 mouse leukemia cell growth with an ID50 as low as 0.5 ng/ml. Further investigations into the structure-activity relationship revealed that specific modifications to the dioxolane structure enhanced cytotoxicity against cancer cells .
Data Table: Summary of Biological Activities
Aplicaciones Científicas De Investigación
Chemical Synthesis
1. Synthesis of Amides and Esters
2,2-Dimethyl-1,3-dioxolan-4-one is utilized in synthesizing N,N-disubstituted amides of glycolic acid. It acts as a key intermediate for compounds like N,N-diethyl-2-hydroxyacetamide and other derivatives. This application is significant in producing pharmaceuticals and agrochemicals .
2. Formation of Bio-based Solvents
Recent research highlights the potential of this compound derivatives as bio-based solvents. One notable derivative is methyl(2,2-dimethyl-1,3-dioxolan-4-yl)methyl carbonate (MMC), which can be synthesized from glycerol. This compound has been studied for its properties as an alternative aprotic solvent, addressing sustainability and toxicity concerns associated with traditional solvents .
3. Synthesis of Glyceryl Phosphates
The compound is also involved in synthesizing glyceryl phosphates, which are important in biochemistry and medicinal chemistry. Its ability to form stable intermediates makes it a valuable reagent in various synthetic pathways .
Biological Applications
1. Pharmaceutical Development
this compound has been applied in the synthesis of biologically active compounds. For instance, it has been utilized in the synthesis of nelfinavir, an HIV protease inhibitor. This demonstrates its utility in developing therapeutic agents .
2. Toxicity Studies
The compound’s derivatives have been evaluated for their toxicity profiles. For example, studies have shown that certain derivatives can cause skin irritation and serious eye damage; thus, understanding these properties is crucial for safe handling and application in pharmaceuticals .
Case Studies
Q & A
Basic Research Questions
Q. What are the optimal synthetic routes for 2,2-dimethyl-1,3-dioxolan-4-one, and how can reaction conditions be systematically optimized?
The compound can be synthesized via glycerol acetalization with acetone under acid catalysis, yielding solketal (2,2-dimethyl-1,3-dioxolan-4-yl methanol) as a primary product. A novel method involves using sulfuric acid as a catalyst in refluxing acetone, achieving ~76% yield for derivatives . For optimization, experimental design matrices (e.g., varying temperature, catalyst concentration, and solvent ratios) combined with statistical tools like the Statgraphics Centurion package are recommended. DRIFT spectroscopy (Perkin Elmer Frontier with an environmental chamber) can monitor reaction progress and intermediate stability .
Q. How can the purity and structural integrity of this compound derivatives be validated?
Use nuclear magnetic resonance (NMR) spectroscopy (e.g., ¹H and ¹³C NMR at 400 MHz and 101 MHz, respectively) to confirm regiochemistry and functional group retention. For example, allyl-functionalized derivatives show characteristic peaks for methoxy (δ 3.84 ppm) and dioxolane carbonyl (δ 170.63 ppm) groups . Size exclusion chromatography (SEC) with refractive index detection is critical for assessing molecular weight distribution in polymerization products, though oligomerization risks require iterative catalyst screening (e.g., TfOH vs. neopentanol) .
Advanced Research Questions
Q. What methodological challenges arise in achieving high molecular weight polymers from this compound, and how can they be resolved?
Bulk polymerization using organocatalysts like triflic acid (TfOH) often yields low-molecular-weight oligomers (Mn < 2000 Da) due to chain-transfer reactions. Advanced strategies include:
- Catalyst screening : Testing Brønsted/Lewis acids (e.g., H2SO4, TiCl4) to balance reactivity and side reactions.
- Solvent-free conditions : Mitigating chain termination by eliminating solvent-induced steric hindrance.
- Initiator ratios : Adjusting initiator:catalyst:monomer ratios (e.g., 1:10:200) to control propagation kinetics .
SEC chromatograms with multi-angle light scattering (MALS) detectors provide real-time molecular weight tracking .
Q. How can researchers resolve contradictions in reaction outcomes when derivatizing this compound with nucleophiles?
Discrepancies in amidation or esterification yields may stem from steric hindrance at the dioxolane ring or competing ring-opening pathways. To address this:
- Kinetic studies : Monitor reaction progress via in situ FTIR to detect intermediate species (e.g., glycolic acid derivatives).
- Computational modeling : Density functional theory (DFT) can predict regioselectivity in nucleophilic attacks.
- Protecting group strategies : Introduce temporary groups (e.g., benzyl phosphate) to stabilize reactive sites during functionalization .
Q. What advanced characterization techniques are critical for analyzing complex reaction mixtures involving this compound?
- High-resolution mass spectrometry (HRMS) : Resolve isobaric intermediates in multicomponent systems (e.g., copolymerization with vinyl monomers).
- X-ray crystallography : Confirm stereochemistry of crystalline derivatives, though the compound’s low melting point may require cryogenic conditions.
- Thermogravimetric analysis (TGA) : Assess thermal stability of polymers, particularly for applications in biodegradable polyesters .
Q. How can researchers design experiments to study the compound’s reactivity in solvent-free, organocatalytic systems?
- Phase behavior analysis : Use differential scanning calorimetry (DSC) to determine monomer-catalyst miscibility.
- Reaction calorimetry : Quantify exothermicity to optimize safety and scalability.
- Alfrey-Price copolymerization parameters : Predict monomer reactivity ratios (e.g., with acrylates) to tailor copolymer properties .
Q. Methodological Tables
Catalyst | Polymer Mn (Da) | PDI | Conditions | Reference |
---|---|---|---|---|
TfOH | 1800 | 1.2 | Bulk, 100°C | |
Neopentanol | Oligomers only | N/A | Solvent-free |
Comparación Con Compuestos Similares
Comparison with Structural Analogs
Substituted 1,3-Dioxolan-4-one Derivatives
Table 1: Structural and Functional Comparison
Métodos De Preparación
Solvent-Free Synthesis via Intermediate Oxidation
A prominent method for synthesizing 2,2-dimethyl-1,3-dioxolan-4-one involves a two-step process starting with glycerol. In the first step, glycerol reacts with 2,2-propanal dimethyl acetal under solvent-free conditions using p-toluenesulfonic acid (TsOH) as a catalyst. This reaction forms 2,2-dimethyl-1,3-dioxolane-4-methanol as an intermediate with a yield of 99.3% . The absence of solvents minimizes waste and simplifies purification, as byproducts like methanol are removed via distillation during the reaction .
Ketal Cyclization of Carboxylic Acid Monoglycerides
An alternative industrial approach involves ketal cyclization of carboxylic acid monoglycerides. Glycerol reacts with carboxylic acids (e.g., stearic acid) under acidic catalysis to form monoglyceride esters, which then undergo cyclization with acetone . This method achieves 2,2-dimethyl-4-hydroxymethyl-1,3-dioxolane with high selectivity, though hydrolysis and subsequent oxidation steps are required to convert the hydroxymethyl group to a ketone .
Key parameters for this method include:
-
Catalyst selection : Sulfonic acid resins or mineral acids (e.g., H₂SO₄) optimize cyclization efficiency.
-
Temperature control : Reactions conducted at 20–50°C prevent side reactions such as over-oxidation .
Direct Cyclocondensation of Glycerol Derivatives
Direct cyclocondensation of glycerol with ketones or aldehydes offers a streamlined route. For example, glycerol and acetone react in the presence of acidic catalysts (e.g., TsOH or Amberlyst-15) to form 2,2-dimethyl-1,3-dioxolane derivatives . While this method typically yields the hydroxymethyl or aldehyde variants, modifying the stoichiometry of acetone and introducing oxidative agents (e.g., TEMPO/NaClO) could shift the pathway toward ketone formation.
Comparative Analysis of Methodologies
Challenges and Optimization Strategies
-
Selectivity issues : Over-oxidation to carboxylic acids or undesired ring-opening byproducts remains a hurdle. Using milder oxidants (e.g., Dess-Martin periodinane) or kinetic control via low-temperature reactions can mitigate this .
-
Catalyst recovery : Homogeneous catalysts like TsOH require neutralization and separation, increasing costs. Heterogeneous alternatives (e.g., sulfonated carbon) enhance recyclability .
Propiedades
IUPAC Name |
2,2-dimethyl-1,3-dioxolan-4-one | |
---|---|---|
Source | PubChem | |
URL | https://pubchem.ncbi.nlm.nih.gov | |
Description | Data deposited in or computed by PubChem | |
InChI |
InChI=1S/C5H8O3/c1-5(2)7-3-4(6)8-5/h3H2,1-2H3 | |
Source | PubChem | |
URL | https://pubchem.ncbi.nlm.nih.gov | |
Description | Data deposited in or computed by PubChem | |
InChI Key |
JKUCOZWILDFXMF-UHFFFAOYSA-N | |
Source | PubChem | |
URL | https://pubchem.ncbi.nlm.nih.gov | |
Description | Data deposited in or computed by PubChem | |
Canonical SMILES |
CC1(OCC(=O)O1)C | |
Source | PubChem | |
URL | https://pubchem.ncbi.nlm.nih.gov | |
Description | Data deposited in or computed by PubChem | |
Molecular Formula |
C5H8O3 | |
Source | PubChem | |
URL | https://pubchem.ncbi.nlm.nih.gov | |
Description | Data deposited in or computed by PubChem | |
DSSTOX Substance ID |
DTXSID60403513 | |
Record name | 2,2-Dimethyl-1,3-dioxolan-4-one | |
Source | EPA DSSTox | |
URL | https://comptox.epa.gov/dashboard/DTXSID60403513 | |
Description | DSSTox provides a high quality public chemistry resource for supporting improved predictive toxicology. | |
Molecular Weight |
116.11 g/mol | |
Source | PubChem | |
URL | https://pubchem.ncbi.nlm.nih.gov | |
Description | Data deposited in or computed by PubChem | |
CAS No. |
4158-86-5 | |
Record name | 2,2-Dimethyl-1,3-dioxolan-4-one | |
Source | EPA DSSTox | |
URL | https://comptox.epa.gov/dashboard/DTXSID60403513 | |
Description | DSSTox provides a high quality public chemistry resource for supporting improved predictive toxicology. | |
Record name | 2,2-Dimethyl-1,3-dioxolan-4-one | |
Source | European Chemicals Agency (ECHA) | |
URL | https://echa.europa.eu/information-on-chemicals | |
Description | The European Chemicals Agency (ECHA) is an agency of the European Union which is the driving force among regulatory authorities in implementing the EU's groundbreaking chemicals legislation for the benefit of human health and the environment as well as for innovation and competitiveness. | |
Explanation | Use of the information, documents and data from the ECHA website is subject to the terms and conditions of this Legal Notice, and subject to other binding limitations provided for under applicable law, the information, documents and data made available on the ECHA website may be reproduced, distributed and/or used, totally or in part, for non-commercial purposes provided that ECHA is acknowledged as the source: "Source: European Chemicals Agency, http://echa.europa.eu/". Such acknowledgement must be included in each copy of the material. ECHA permits and encourages organisations and individuals to create links to the ECHA website under the following cumulative conditions: Links can only be made to webpages that provide a link to the Legal Notice page. | |
Retrosynthesis Analysis
AI-Powered Synthesis Planning: Our tool employs the Template_relevance Pistachio, Template_relevance Bkms_metabolic, Template_relevance Pistachio_ringbreaker, Template_relevance Reaxys, Template_relevance Reaxys_biocatalysis model, leveraging a vast database of chemical reactions to predict feasible synthetic routes.
One-Step Synthesis Focus: Specifically designed for one-step synthesis, it provides concise and direct routes for your target compounds, streamlining the synthesis process.
Accurate Predictions: Utilizing the extensive PISTACHIO, BKMS_METABOLIC, PISTACHIO_RINGBREAKER, REAXYS, REAXYS_BIOCATALYSIS database, our tool offers high-accuracy predictions, reflecting the latest in chemical research and data.
Strategy Settings
Precursor scoring | Relevance Heuristic |
---|---|
Min. plausibility | 0.01 |
Model | Template_relevance |
Template Set | Pistachio/Bkms_metabolic/Pistachio_ringbreaker/Reaxys/Reaxys_biocatalysis |
Top-N result to add to graph | 6 |
Feasible Synthetic Routes
Descargo de responsabilidad e información sobre productos de investigación in vitro
Tenga en cuenta que todos los artículos e información de productos presentados en BenchChem están destinados únicamente con fines informativos. Los productos disponibles para la compra en BenchChem están diseñados específicamente para estudios in vitro, que se realizan fuera de organismos vivos. Los estudios in vitro, derivados del término latino "in vidrio", involucran experimentos realizados en entornos de laboratorio controlados utilizando células o tejidos. Es importante tener en cuenta que estos productos no se clasifican como medicamentos y no han recibido la aprobación de la FDA para la prevención, tratamiento o cura de ninguna condición médica, dolencia o enfermedad. Debemos enfatizar que cualquier forma de introducción corporal de estos productos en humanos o animales está estrictamente prohibida por ley. Es esencial adherirse a estas pautas para garantizar el cumplimiento de los estándares legales y éticos en la investigación y experimentación.