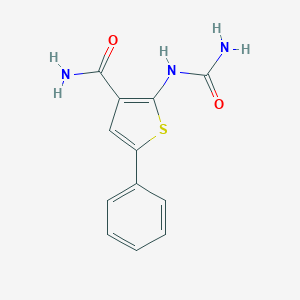
5-Phenyl-2-Ureidothiophene-3-Carboxamide
Descripción general
Descripción
Mecanismo De Acción
El Inhibidor de IKK2 VI ejerce sus efectos inhibiendo selectivamente la actividad de IKK2. Esta inhibición previene la fosforilación y la posterior degradación de las proteínas IκB, lo que a su vez evita la activación de NF-κB. NF-κB es un factor de transcripción que regula la expresión de varios genes involucrados en la inflamación, la respuesta inmune y la supervivencia celular. Al bloquear esta vía, el Inhibidor de IKK2 VI puede reducir la inflamación y potencialmente ralentizar la progresión de enfermedades asociadas con la activación aberrante de NF-κB .
Análisis Bioquímico
Biochemical Properties
IKK-2 Inhibitor VI acts as part of an IKK complex in the canonical NF-κB pathway, phosphorylating inhibitors of NF-κB (IκBs) to initiate signaling . It is an ureido-thiophenecarboxamide compound that exhibits potent inhibitory activity against IKK-2 . The compound interacts with IKK-2 in an ATP-competitive manner, binding tightly with a relatively slow off rate .
Cellular Effects
IKK-2 Inhibitor VI has been shown to have profound effects on various types of cells and cellular processes. It down-regulates p-IκBα protein expression in a concentration-dependent manner, causing growth inhibition and an increase in G0/G1 cells, but does not induce apoptosis . It also prevents vincristine-induced nuclear translocation of the p65 subunit of NF-κB .
Molecular Mechanism
The molecular mechanism of IKK-2 Inhibitor VI involves its interaction with IKK-2, leading to the inhibition of the NF-κB pathway . It acts as a potent, cell-permeable, reversible, and ATP-competitive inhibitor of IKK-2 . This results in the prevention of IκB phosphorylation and degradation, thereby inhibiting the activation of NF-κB .
Temporal Effects in Laboratory Settings
In laboratory settings, IKK-2 Inhibitor VI has been shown to have long-term effects on cellular function. For instance, it has been demonstrated that the compound leads to synergistic enhancement of vincristine cytotoxicity in lymphoma over time
Dosage Effects in Animal Models
For instance, it was found to enhance CHOP anti-tumor activity significantly in the WSU-DLCL2-SCID model .
Metabolic Pathways
IKK-2 Inhibitor VI is involved in the NF-κB signaling pathway . It interacts with IKK-2, leading to the inhibition of the NF-κB pathway
Métodos De Preparación
La síntesis del Inhibidor de IKK2 VI implica la formación de una estructura de ureido-tiofeno-carboxamida. La ruta sintética normalmente incluye la reacción de ácido 5-feniltiofeno-3-carboxílico con una amina adecuada para formar la amida correspondiente. Los métodos de producción industrial pueden implicar la optimización de las condiciones de reacción para mejorar el rendimiento y la pureza, como el control de la temperatura, el pH y el tiempo de reacción.
Análisis De Reacciones Químicas
El Inhibidor de IKK2 VI sufre varios tipos de reacciones químicas, incluyendo:
Oxidación: El anillo de tiofeno puede oxidarse bajo condiciones específicas.
Reducción: El grupo nitro, si está presente, puede reducirse a una amina.
Sustitución: El anillo fenilo puede sufrir reacciones de sustitución aromática electrofílica. Los reactivos comunes utilizados en estas reacciones incluyen agentes oxidantes como el permanganato de potasio, agentes reductores como el hidrógeno gaseoso con un catalizador de paladio y electrófilos como el bromo para reacciones de sustitución.
Aplicaciones Científicas De Investigación
El Inhibidor de IKK2 VI tiene una amplia gama de aplicaciones de investigación científica:
Química: Se utiliza como un compuesto de herramienta para estudiar la vía de señalización de NF-κB.
Biología: Ayuda a comprender el papel de IKK2 en procesos celulares como la inflamación y la respuesta inmune.
Medicina: Investigado por sus posibles efectos terapéuticos en el tratamiento de enfermedades inflamatorias y ciertos tipos de cáncer.
Industria: Utilizado en el desarrollo de nuevos fármacos dirigidos a la vía de NF-κB
Comparación Con Compuestos Similares
El Inhibidor de IKK2 VI se compara con otros compuestos similares como:
Inhibidor de IKK2 IV: Otro potente inhibidor de IKK2 con una estructura química diferente.
Inhibidor de IKK2 VIII: Conocido por su alta selectividad hacia IKK2.
SC-514: Un inhibidor selectivo de IKK2 con un mecanismo de acción diferente. El Inhibidor de IKK2 VI es único debido a su estructura específica de ureido-tiofeno-carboxamida, que proporciona alta potencia y permeabilidad celular
Actividad Biológica
5-Phenyl-2-Ureidothiophene-3-Carboxamide, also known as IKK-2 Inhibitor VI, is a compound that has garnered attention for its significant biological activity, particularly as an inhibitor of the IκB kinase (IKK) complex. This article explores its biological activity, mechanisms of action, and potential therapeutic applications based on diverse research findings.
Target and Inhibition
The primary target of this compound is the IκB kinase 2 (IKK-2), which plays a crucial role in the NF-κB signaling pathway. By inhibiting IKK-2, this compound prevents the phosphorylation of IκB proteins, leading to the suppression of NF-κB activation. This inhibition effectively dampens inflammatory responses and modulates various cellular processes related to immune response and tumor progression.
Biochemical Pathways
The inhibition of IKK-2 by this compound disrupts canonical NF-κB signaling, which is vital for cell survival and proliferation during inflammatory processes. The compound acts as a reversible and ATP-competitive inhibitor, demonstrating potent effects on various cell types.
Biological Activity
Cellular Effects
Research indicates that this compound down-regulates phosphorylated IκBα protein expression in a concentration-dependent manner. This results in growth inhibition in several cancer cell lines without inducing apoptosis, suggesting its potential utility in cancer therapies.
Cytotoxicity Studies
In cytotoxicity assays conducted on mouse hippocampal neuronal cells (HT-22) and microglial cells (BV-2), the compound exhibited varying degrees of cytotoxicity depending on concentration. Notably, certain derivatives showed no significant decrease in cell viability at concentrations up to 10 µM, indicating a favorable safety profile .
Research Findings and Case Studies
In Vivo Studies
In animal models, particularly the WSU-DLCL2-SCID model, this compound has been shown to enhance the anti-tumor activity of CHOP (a chemotherapy regimen) significantly. This suggests that it may not only inhibit tumor growth directly but also potentiate the effects of existing cancer treatments.
Impact on Osteoblast Differentiation
A study explored the compound's effects on osteoblast differentiation by inhibiting the IKK-NF-κB pathway. The results indicated that by preventing β-catenin degradation—a key protein in bone formation—the compound promotes osteoblast differentiation, which could have implications for treating bone-related disorders .
Summary of Biological Activities
Activity | Description |
---|---|
IKK-2 Inhibition | Potent inhibitor affecting NF-κB signaling pathway |
Cytotoxicity | Varies with concentration; some derivatives maintain cell viability |
Tumor Growth Inhibition | Enhances anti-tumor effects when combined with CHOP therapy |
Osteoblast Differentiation | Promotes differentiation by stabilizing β-catenin |
Propiedades
IUPAC Name |
2-(carbamoylamino)-5-phenylthiophene-3-carboxamide | |
---|---|---|
Source | PubChem | |
URL | https://pubchem.ncbi.nlm.nih.gov | |
Description | Data deposited in or computed by PubChem | |
InChI |
InChI=1S/C12H11N3O2S/c13-10(16)8-6-9(7-4-2-1-3-5-7)18-11(8)15-12(14)17/h1-6H,(H2,13,16)(H3,14,15,17) | |
Source | PubChem | |
URL | https://pubchem.ncbi.nlm.nih.gov | |
Description | Data deposited in or computed by PubChem | |
InChI Key |
PSVUSJKZJQMCSP-UHFFFAOYSA-N | |
Source | PubChem | |
URL | https://pubchem.ncbi.nlm.nih.gov | |
Description | Data deposited in or computed by PubChem | |
Canonical SMILES |
C1=CC=C(C=C1)C2=CC(=C(S2)NC(=O)N)C(=O)N | |
Source | PubChem | |
URL | https://pubchem.ncbi.nlm.nih.gov | |
Description | Data deposited in or computed by PubChem | |
Molecular Formula |
C12H11N3O2S | |
Source | PubChem | |
URL | https://pubchem.ncbi.nlm.nih.gov | |
Description | Data deposited in or computed by PubChem | |
DSSTOX Substance ID |
DTXSID40423557 | |
Record name | IKK-2 Inhibitor VI | |
Source | EPA DSSTox | |
URL | https://comptox.epa.gov/dashboard/DTXSID40423557 | |
Description | DSSTox provides a high quality public chemistry resource for supporting improved predictive toxicology. | |
Molecular Weight |
261.30 g/mol | |
Source | PubChem | |
URL | https://pubchem.ncbi.nlm.nih.gov | |
Description | Data deposited in or computed by PubChem | |
CAS No. |
354811-10-2 | |
Record name | IKK-2 Inhibitor VI | |
Source | EPA DSSTox | |
URL | https://comptox.epa.gov/dashboard/DTXSID40423557 | |
Description | DSSTox provides a high quality public chemistry resource for supporting improved predictive toxicology. | |
Synthesis routes and methods
Procedure details
Retrosynthesis Analysis
AI-Powered Synthesis Planning: Our tool employs the Template_relevance Pistachio, Template_relevance Bkms_metabolic, Template_relevance Pistachio_ringbreaker, Template_relevance Reaxys, Template_relevance Reaxys_biocatalysis model, leveraging a vast database of chemical reactions to predict feasible synthetic routes.
One-Step Synthesis Focus: Specifically designed for one-step synthesis, it provides concise and direct routes for your target compounds, streamlining the synthesis process.
Accurate Predictions: Utilizing the extensive PISTACHIO, BKMS_METABOLIC, PISTACHIO_RINGBREAKER, REAXYS, REAXYS_BIOCATALYSIS database, our tool offers high-accuracy predictions, reflecting the latest in chemical research and data.
Strategy Settings
Precursor scoring | Relevance Heuristic |
---|---|
Min. plausibility | 0.01 |
Model | Template_relevance |
Template Set | Pistachio/Bkms_metabolic/Pistachio_ringbreaker/Reaxys/Reaxys_biocatalysis |
Top-N result to add to graph | 6 |
Feasible Synthetic Routes
Q1: What is the primary mechanism of action of IKK-2 Inhibitor VI and how does it impact osteoblast differentiation?
A1: IKK-2 Inhibitor VI specifically targets and inhibits the IKK-NF-κB signaling pathway. [] This pathway is activated by various stimuli, including traumatic force and inflammatory signals like Pg.LPS. [] Upon activation, IKK-NF-κB promotes the degradation of β-catenin, a key protein in the Wnt signaling pathway essential for osteoblast differentiation and bone formation. [] By inhibiting IKK-NF-κB, the inhibitor prevents β-catenin degradation, allowing the Wnt pathway to function and promote osteoblast differentiation. []
Q2: Are there any in vivo studies demonstrating the efficacy of IKK-2 Inhibitor VI in mitigating bone loss?
A2: Yes, a study using a rat model of occlusal trauma (a form of mechanical stress) showed that IKK-2 Inhibitor VI administration significantly reduced bone loss. [] This protective effect was linked to the inhibition of IKK-NF-κB signaling and the subsequent upregulation of osteogenic markers like β-catenin, osteocalcin (OCN), and Runx2. []
Q3: Besides its potential in bone health, has IKK-2 Inhibitor VI been studied for other therapeutic applications?
A3: Research suggests that IKK-2 Inhibitor VI could be utilized for topical pain management. [] By inhibiting the NF-κB family of transcription factors, which play a role in inflammation and pain signaling, topical administration of the compound may provide localized pain relief. [] This approach aims to minimize potential systemic side effects associated with systemic administration, such as immunosuppression. []
Descargo de responsabilidad e información sobre productos de investigación in vitro
Tenga en cuenta que todos los artículos e información de productos presentados en BenchChem están destinados únicamente con fines informativos. Los productos disponibles para la compra en BenchChem están diseñados específicamente para estudios in vitro, que se realizan fuera de organismos vivos. Los estudios in vitro, derivados del término latino "in vidrio", involucran experimentos realizados en entornos de laboratorio controlados utilizando células o tejidos. Es importante tener en cuenta que estos productos no se clasifican como medicamentos y no han recibido la aprobación de la FDA para la prevención, tratamiento o cura de ninguna condición médica, dolencia o enfermedad. Debemos enfatizar que cualquier forma de introducción corporal de estos productos en humanos o animales está estrictamente prohibida por ley. Es esencial adherirse a estas pautas para garantizar el cumplimiento de los estándares legales y éticos en la investigación y experimentación.