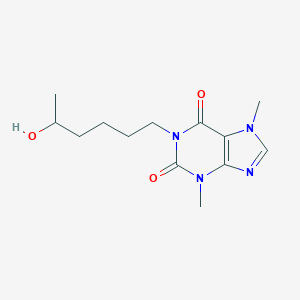
(+/-)-Lisofylline
Descripción general
Descripción
(+/-)-Lisofylline (LSF), chemically known as 1-(5-hydroxyhexyl)-3,7-dimethylxanthine, is a racemic mixture of the enantiomers (R)-(−)-LSF and (S)-(+)-LSF. It is an active metabolite of pentoxifylline, a xanthine derivative used for peripheral vascular diseases . LSF has garnered attention for its anti-inflammatory and antioxidant properties, which are mediated through distinct mechanisms:
- Inhibition of pro-inflammatory cytokines: LSF suppresses interleukin-1β (IL-1β), IL-6, and tumor necrosis factor-α (TNF-α) production, key drivers of inflammation .
- Modulation of lipid signaling: It inhibits phosphatidic acid synthesis in neutrophils, reducing oxidant-mediated vascular damage .
- Protection against oxidative stress: LSF decreases lipid peroxidation and mitigates hyperoxia-induced lung injury by targeting CREB activation .
Preclinical studies highlight its efficacy in autoimmune diseases, particularly Type 1 diabetes, where it preserves pancreatic β-cell function and delays diabetes onset in non-obese diabetic (NOD) mice .
Métodos De Preparación
Chemoenzymatic Deracemization Strategy
The most advanced preparation method for (±)-LSF involves a one-pot two-step chemoenzymatic deracemization protocol combining non-selective oxidation and stereoselective bioreduction . This approach addresses the limitations of traditional kinetic resolution methods, which often suffer from low yields (<50%) .
Oxidation Step: Laccase/TEMPO-Mediated Aerobic Conversion
The first step converts racemic LSF into pentoxifylline (PTX), a prochiral ketone intermediate, using laccase from Trametes versicolor (LTv) and 2,2,6,6-tetramethylpiperidinyloxy (TEMPO) as a redox mediator. Key parameters include:
-
Solvent optimization : Acetone (20% v/v) in citrate buffer (pH 5.0) achieved 40% conversion of racemic LSF after 96 hours (Table 1) .
-
Catalyst loading : Sequential additions of LTv (14.5 U mL⁻¹) and TEMPO (165 mol%) over 120 hours increased conversion to 95% .
Solvent | LogPᵇ | Co-Solvent (% v/v) | Conversion (%)ᵈ |
---|---|---|---|
Acetone | 0.20 | 50 | 16 |
Ethyl acetate | 0.29 | 20 | 16 |
THF | 0.40 | 20 | 12 |
Table 1 : Solvent effects on LTv/TEMPO-mediated oxidation of racemic LSF .
Bioreduction Step: Alcohol Dehydrogenase (ADH)-Catalyzed Stereoselection
The second step employs recombinant ADHs from Rhodococcus ruber (ADH-A) and Lactobacillus kefiri (LK-ADH) to reduce PTX into enantiopure LSF :
-
ADH-A : Produces (S)-LSF with 93% conversion and 67% yield.
-
LK-ADH : Generates (R)-LSF with 94% conversion and 65% yield.
This dual-enzyme system achieves >99% enantiomeric excess (ee), surpassing earlier methods like microbial bioreduction (≤80% ee) .
Optimization of Oxidation Conditions
Co-Solvent and Reaction Time
Increasing acetone concentration to 20% (v/v) improved substrate solubility and reaction homogeneity, boosting conversion from 16% to 40% (Table 2) . Prolonged reaction times (120 hours) and staggered catalyst additions mitigated enzyme inhibition by residual substrates.
Acetone (% v/v) | Reaction Time (h) | Conversion (%) |
---|---|---|
10 | 96 | 30 |
20 | 96 | 40 |
50 | 96 | 28 |
Table 2 : Impact of acetone concentration on LSF oxidation .
Catalyst Stoichiometry
A linear relationship exists between TEMPO loading and conversion efficiency (Table 3). However, excessive TEMPO (>165 mol%) reduced isolated yields due to competing side reactions .
TEMPO (mol%) | LTv (U mL⁻¹) | Reaction Time (h) | Conversion (%) | Yield (%) |
---|---|---|---|---|
33 | 2.9 | 48 | 55 | 48 |
132 | 11.6 | 96 | 87 | 75 |
165 | 14.5 | 120 | 95 | 68 |
Table 3 : Catalyst optimization for LSF oxidation .
Scalability and Industrial Considerations
Large-Scale Deracemization
At 0.16 mmol scale, the optimized protocol achieved 95% conversion and 68% isolated yield of PTX (Table 4). Challenges included enzyme inactivation at high substrate concentrations and purification losses due to residual TEMPO .
Scale (mmol) | LTv (U mL⁻¹) | TEMPO (mol%) | Yield (%) |
---|---|---|---|
0.08 | 14.5 | 165 | 68 |
0.16 | 14.5 | 165 | 56 |
Table 4 : Scalability of LTv/TEMPO-mediated LSF oxidation .
Comparative Analysis with Alternative Methods
Traditional synthetic routes for LSF face limitations:
-
Chemical oxidation : Requires stoichiometric toxic oxidants (e.g., CrO₃), generating hazardous waste .
-
Lipase-catalyzed kinetic resolution : Limited to ≤50% yield due to racemization .
-
Cytochrome P450 monooxygenases : Low enantioselectivity (≤50% ee) .
In contrast, the chemoenzymatic approach offers greener conditions (aqueous media, O₂ as oxidant) and superior stereochemical outcomes.
Análisis De Reacciones Químicas
Lisofylline undergoes several types of chemical reactions, including oxidation and reduction. One notable reaction is the chemoenzymatic deracemization of lisofylline, which involves a tandem bi-enzymatic oxidation-reduction reaction sequence. This process uses laccase from Trametes versicolor and 2,2,6,6-tetramethylpiperidinyloxy radical as a redox mediator for the oxidation step, followed by stereoselective bioreduction using alcohol dehydrogenases . Common reagents used in these reactions include laccase, TEMPO, and alcohol dehydrogenases. The major products formed from these reactions are enantiomerically pure forms of lisofylline.
Aplicaciones Científicas De Investigación
Diabetes Management
Mechanism of Action:
LSF exhibits anti-inflammatory properties that protect pancreatic beta cells from cytokine-induced damage, a critical factor in type 1 diabetes. It suppresses pro-inflammatory cytokines, thereby preserving insulin secretion and promoting beta cell survival .
Clinical Studies and Findings:
- Beta Cell Protection: LSF has been shown to restore insulin secretion in INS-1 cells exposed to inflammatory cytokines such as IL-1β and TNFα. In studies, LSF treatment significantly increased both basal and glucose-stimulated insulin release compared to untreated controls .
- Animal Models: In diabetic mouse models, LSF demonstrated a stable reduction in glucose levels when administered in a self-assembling micellar form, which enhanced its bioavailability and half-life .
Autoimmune Disorders
Potential Applications:
LSF has been proposed as a therapeutic agent for various autoimmune conditions beyond diabetes, including autoimmune recurrence following islet transplantation. Its ability to inhibit IL-12 signaling suggests a broader immunomodulatory role that could be beneficial in managing autoimmune responses .
Case Studies:
- Autoimmunity Prevention: Research indicates that LSF may help maintain functional beta cell mass during islet isolation, potentially improving outcomes for patients undergoing islet transplantation .
Cancer Therapy Support
Role in Chemotherapy:
Initially developed as an adjunct therapy during cancer treatment, LSF was designed to reduce mortality associated with infections during chemotherapy. Its immunomodulatory effects may enhance the overall efficacy of cancer treatments by mitigating inflammatory responses that can compromise patient health during such therapies .
Research Insights:
Mecanismo De Acción
Lisofylline exerts its effects primarily by inhibiting the production of inflammatory cytokines, which play a crucial role in the body’s immune response . It blocks interleukin-12 signaling and STAT-4 activation in target cells and tissues, pathways that are important in inflammation and autoimmune damage to insulin-producing cells . By improving cellular mitochondrial function and reducing glucose-induced changes in cells, lisofylline helps protect against inflammation and autoimmune damage.
Comparación Con Compuestos Similares
Structural and Functional Analogues
Pentoxifylline
- Structure : Parent compound of LSF; differs by a hydroxyl group at the N1 position.
- Mechanism : Broad anti-inflammatory effects via TNF-α downregulation and improved erythrocyte flexibility .
- Metabolism : Converted to LSF enantiomers in human liver microsomes via CYP1A2, though inefficiently (40% conversion) .
- Clinical Use : Approved for peripheral artery disease and alcoholic hepatitis, unlike LSF, which remains experimental .
Theophylline and Caffeine
- Structure : Xanthine derivatives lacking the N1 hydroxyhexyl group of LSF.
- Mechanism: Primarily act as phosphodiesterase inhibitors and adenosine receptor antagonists.
- Key Difference : Neither inhibits FTO, an RNA demethylase linked to adipogenesis. LSF inhibits FTO with an IC50 of 2.2 µM, attributed to its N1 alkyl chain .
LSF Analogues
- Structural Modifications: Analogues with 5-aza-7-deazaxanthine or non-xanthine skeletons show improved metabolic stability and apoptosis protection in β-cells .
- Enantiomers : (S)-LSF, synthesized via chemoenzymatic deracemization, exhibits 94% enantiomeric excess (ee) and superior bioactivity compared to the racemic mixture .
Pharmacokinetic and Pharmacodynamic Comparisons
Research Findings and Clinical Implications
- Anti-Diabetic Efficacy: LSF reduces insulitis and diabetes incidence in NOD mice by 50%, outperforming pentoxifylline, which lacks targeted β-cell protection .
- FTO Inhibition : LSF’s potency against FTO (IC50 = 2.2 µM) is unmatched by other xanthines, making it a candidate for obesity-related disorders .
Actividad Biológica
(+/-)-Lisofylline (LSF) is a xanthine derivative that has garnered attention for its diverse biological activities, particularly in the context of anti-inflammatory responses and protection against cellular damage. This article synthesizes findings from various studies to present a comprehensive overview of the biological activity of LSF, focusing on its mechanisms of action, therapeutic potential, and relevant case studies.
LSF exerts its biological effects primarily through the modulation of inflammatory pathways and mitochondrial function.
- Anti-inflammatory Properties : LSF has been shown to inhibit the effects of proinflammatory cytokines such as IL-1β, TNFα, and IFNγ on pancreatic β-cells. In a study involving INS-1 cells, LSF restored insulin secretion and cell viability that were otherwise diminished by cytokine exposure. This protective effect is linked to enhanced mitochondrial metabolism and reduced nuclear DNA breakage in treated cells .
- Mitochondrial Metabolism : The compound promotes mitochondrial function, which is crucial for maintaining cellular energy levels and viability. The study indicated that LSF treatment increased MTT metabolism and ATP levels in INS-1 cells, suggesting improved mitochondrial activity .
- Extracellular Matrix Regulation : In human mesangial cells exposed to high glucose levels or angiotensin II, LSF significantly reduced the secretion of extracellular matrix proteins such as fibronectin and TGF-β. This effect is attributed to the modulation of signaling pathways involving cAMP-responsive binding elements and p38 MAPK, indicating a potential role in preventing diabetic nephropathy .
Case Studies
Several studies have highlighted the therapeutic potential of LSF in various conditions:
- Type 1 Diabetes : In non-obese diabetic (NOD) mice models, LSF demonstrated protective effects against Th1 cytokine-induced dysfunction in pancreatic β-cells. This suggests that LSF could be a candidate for preventing or treating type 1 diabetes by preserving insulin secretion capabilities .
- Sepsis-Induced Lung Injury : A study involving minipigs showed that LSF administration prior to sepsis induction led to significant improvements in pulmonary hypertension and systemic hypotension. The treatment reduced neutrophil counts and plasma TNF-alpha levels, indicating its potential utility in managing sepsis-related complications .
- Diabetic Nephropathy : Research indicates that LSF may offer therapeutic benefits for diabetic nephropathy by mitigating ECM accumulation in renal cells. By regulating TGF-β production and related signaling pathways, LSF could help prevent kidney damage associated with diabetes .
Summary of Biological Activities
The following table summarizes key findings regarding the biological activities of this compound:
Q & A
Basic Research Questions
Q. What are the standard experimental protocols for synthesizing enantiomerically pure (R)- and (S)-lisofylline using chemoenzymatic approaches?
- Methodological Answer : A two-step redox-driven chemoenzymatic deracemization route employs Trametes versicolor laccase (LTv) with TEMPO as a redox mediator for non-selective oxidation of racemic lisofylline, followed by stereoselective reduction using recombinant alcohol dehydrogenases (ADHs). Optimal conditions include 50 mM lisofylline in pH 5.0 citrate buffer with 10–50% acetonitrile, 30°C, and 120-hour oxidation to achieve >95% conversion. ADHs like E. coli/ADH-A yield (S)-lisofylline (94% enantiomeric excess) .
Q. How does lisofylline modulate IL-12/STAT4 signaling in Th1 differentiation?
- Methodological Answer : In vitro assays using murine splenic T cells treated with IL-12 (10 ng/mL) and lisofylline (10–40 µM) demonstrate inhibition of STAT4 phosphorylation via flow cytometry. This suppresses Th1 differentiation, validated by reduced IFN-γ production and IL-12-mediated STAT4 activation. Ex vivo rat islet studies further confirm preservation of insulin secretion under IL-1β stress .
Q. What pharmacokinetic models are used to characterize lisofylline distribution in preclinical studies?
- Methodological Answer : Physiologically based pharmacokinetic (PBPK) modeling in mice after intravenous administration shows biphasic clearance, with arterial blood concentration declining rapidly (t1/2α = 5.2 min) followed by slower elimination (t1/2β = 34.5 min). Parameters like volume of distribution (Vd = 0.6 L/kg) and clearance (Cl = 0.03 L/min/kg) are derived from compartmental analysis .
Advanced Research Questions
Q. How can researchers resolve contradictions between lisofylline’s preclinical efficacy in diabetes models and its failure in ARDS clinical trials?
- Methodological Answer : Preclinical success in NOD mice (e.g., 70% diabetes prevention at 40 mg/kg) contrasts with null outcomes in ARDS trials (28-day mortality: 31.9% vs. 24.7% placebo). Factors include differences in disease pathophysiology, dosing regimens (3 mg/kg in humans vs. higher preclinical doses), and endpoint selection (organ failure vs. cytokine suppression). Mechanistic studies integrating tissue-specific STAT4 inhibition and pharmacokinetic-pharmacodynamic (PK/PD) bridging are critical .
Q. What strategies optimize the LTv/TEMPO system for scalable lisofylline oxidation?
- Methodological Answer : Increasing LTv activity (14.5 U/mL) and TEMPO loading (165 mol%) enhances conversion rates to >95%. Co-solvent screening (e.g., acetonitrile > methanol) improves substrate solubility, while metal ions (Cu<sup>2+</sup>, Zn<sup>2+</sup>) show negligible catalytic enhancement. Reaction scalability requires iterative enzyme replenishment and oxygen saturation monitoring .
Q. How can genetic data (e.g., GWAS) inform lisofylline repurposing for coronary artery disease (CAD)?
- Methodological Answer : PharmGWAS leverages gene-expression connectivity (CMap 2.0) to link lisofylline’s anti-inflammatory profile (WTCS = −5) with CAD-associated pathways (e.g., atherosclerosis). Validated via suppression of serum-free fatty acids and parallels to pentoxifylline’s therapeutic benefits, this approach prioritizes in vivo validation using ApoE<sup>−/−</sup> mouse models .
Q. What experimental designs address lisofylline’s enantiomer-specific bioactivity in autoimmune disease models?
- Methodological Answer : Comparative studies of (R)- and (S)-lisofylline in NOD mice reveal (R)-enantiomer specificity in STAT4 inhibition (IC50 = 0.6 µM) and diabetes prevention. Dose-response experiments (10–100 mg/kg) combined with splenocyte transfer assays validate immune modulation mechanisms. Stereochemical purity is confirmed via chiral HPLC .
Q. Data Contradiction Analysis
Q. Why do metal ions inconsistently affect LTv/TEMPO-mediated lisofylline oxidation?
- Analysis : Screening of Mg<sup>2+</sup>, Fe<sup>2+</sup>, Zn<sup>2+</sup>, and Co<sup>2+</sup> (1–5 mM) shows <10% conversion improvement, suggesting LTv’s insensitivity to metal cofactors. Contrasting results with other laccase systems may arise from differences in enzyme structure or TEMPO-mediated rate-limiting steps. Redox potential measurements and electron paramagnetic resonance (EPR) studies are needed to clarify mediator-enzyme interactions .
Q. Tables
Table 1. Co-solvent Screening for LTv/TEMPO-Catalyzed Lisofylline Oxidation
Co-solvent (v/v%) | Conversion (%) | Yield (%) |
---|---|---|
Acetonitrile (50%) | 95 | 68 |
Methanol (50%) | 82 | 55 |
DMSO (30%) | 65 | 42 |
Conditions: 50 mM rac-1, pH 5.0, 30°C, 120 h . |
Propiedades
IUPAC Name |
1-(5-hydroxyhexyl)-3,7-dimethylpurine-2,6-dione | |
---|---|---|
Source | PubChem | |
URL | https://pubchem.ncbi.nlm.nih.gov | |
Description | Data deposited in or computed by PubChem | |
InChI |
InChI=1S/C13H20N4O3/c1-9(18)6-4-5-7-17-12(19)10-11(14-8-15(10)2)16(3)13(17)20/h8-9,18H,4-7H2,1-3H3 | |
Source | PubChem | |
URL | https://pubchem.ncbi.nlm.nih.gov | |
Description | Data deposited in or computed by PubChem | |
InChI Key |
NSMXQKNUPPXBRG-UHFFFAOYSA-N | |
Source | PubChem | |
URL | https://pubchem.ncbi.nlm.nih.gov | |
Description | Data deposited in or computed by PubChem | |
Canonical SMILES |
CC(CCCCN1C(=O)C2=C(N=CN2C)N(C1=O)C)O | |
Source | PubChem | |
URL | https://pubchem.ncbi.nlm.nih.gov | |
Description | Data deposited in or computed by PubChem | |
Molecular Formula |
C13H20N4O3 | |
Source | PubChem | |
URL | https://pubchem.ncbi.nlm.nih.gov | |
Description | Data deposited in or computed by PubChem | |
DSSTOX Substance ID |
DTXSID6046343 | |
Record name | (+/-)-Lisofylline | |
Source | EPA DSSTox | |
URL | https://comptox.epa.gov/dashboard/DTXSID6046343 | |
Description | DSSTox provides a high quality public chemistry resource for supporting improved predictive toxicology. | |
Molecular Weight |
280.32 g/mol | |
Source | PubChem | |
URL | https://pubchem.ncbi.nlm.nih.gov | |
Description | Data deposited in or computed by PubChem | |
CAS No. |
6493-06-7, 100324-81-0 | |
Record name | Penthydroxyfylline | |
Source | ChemIDplus | |
URL | https://pubchem.ncbi.nlm.nih.gov/substance/?source=chemidplus&sourceid=0006493067 | |
Description | ChemIDplus is a free, web search system that provides access to the structure and nomenclature authority files used for the identification of chemical substances cited in National Library of Medicine (NLM) databases, including the TOXNET system. | |
Record name | Lisofylline [USAN:INN] | |
Source | ChemIDplus | |
URL | https://pubchem.ncbi.nlm.nih.gov/substance/?source=chemidplus&sourceid=0100324810 | |
Description | ChemIDplus is a free, web search system that provides access to the structure and nomenclature authority files used for the identification of chemical substances cited in National Library of Medicine (NLM) databases, including the TOXNET system. | |
Record name | (+/-)-Lisofylline | |
Source | EPA DSSTox | |
URL | https://comptox.epa.gov/dashboard/DTXSID6046343 | |
Description | DSSTox provides a high quality public chemistry resource for supporting improved predictive toxicology. | |
Record name | LISOFYLLINE, (±)- | |
Source | FDA Global Substance Registration System (GSRS) | |
URL | https://gsrs.ncats.nih.gov/ginas/app/beta/substances/R99EE080JS | |
Description | The FDA Global Substance Registration System (GSRS) enables the efficient and accurate exchange of information on what substances are in regulated products. Instead of relying on names, which vary across regulatory domains, countries, and regions, the GSRS knowledge base makes it possible for substances to be defined by standardized, scientific descriptions. | |
Explanation | Unless otherwise noted, the contents of the FDA website (www.fda.gov), both text and graphics, are not copyrighted. They are in the public domain and may be republished, reprinted and otherwise used freely by anyone without the need to obtain permission from FDA. Credit to the U.S. Food and Drug Administration as the source is appreciated but not required. | |
Synthesis routes and methods
Procedure details
Retrosynthesis Analysis
AI-Powered Synthesis Planning: Our tool employs the Template_relevance Pistachio, Template_relevance Bkms_metabolic, Template_relevance Pistachio_ringbreaker, Template_relevance Reaxys, Template_relevance Reaxys_biocatalysis model, leveraging a vast database of chemical reactions to predict feasible synthetic routes.
One-Step Synthesis Focus: Specifically designed for one-step synthesis, it provides concise and direct routes for your target compounds, streamlining the synthesis process.
Accurate Predictions: Utilizing the extensive PISTACHIO, BKMS_METABOLIC, PISTACHIO_RINGBREAKER, REAXYS, REAXYS_BIOCATALYSIS database, our tool offers high-accuracy predictions, reflecting the latest in chemical research and data.
Strategy Settings
Precursor scoring | Relevance Heuristic |
---|---|
Min. plausibility | 0.01 |
Model | Template_relevance |
Template Set | Pistachio/Bkms_metabolic/Pistachio_ringbreaker/Reaxys/Reaxys_biocatalysis |
Top-N result to add to graph | 6 |
Feasible Synthetic Routes
Descargo de responsabilidad e información sobre productos de investigación in vitro
Tenga en cuenta que todos los artículos e información de productos presentados en BenchChem están destinados únicamente con fines informativos. Los productos disponibles para la compra en BenchChem están diseñados específicamente para estudios in vitro, que se realizan fuera de organismos vivos. Los estudios in vitro, derivados del término latino "in vidrio", involucran experimentos realizados en entornos de laboratorio controlados utilizando células o tejidos. Es importante tener en cuenta que estos productos no se clasifican como medicamentos y no han recibido la aprobación de la FDA para la prevención, tratamiento o cura de ninguna condición médica, dolencia o enfermedad. Debemos enfatizar que cualquier forma de introducción corporal de estos productos en humanos o animales está estrictamente prohibida por ley. Es esencial adherirse a estas pautas para garantizar el cumplimiento de los estándares legales y éticos en la investigación y experimentación.