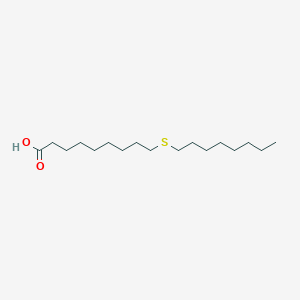
Ácido 10-tiasteárico
Descripción general
Descripción
Aplicaciones Científicas De Investigación
El ácido 10-tiasteárico tiene varias aplicaciones de investigación científica:
Mecanismo De Acción
El ácido 10-tiasteárico ejerce sus efectos inhibiendo la enzima estearoil CoA desaturasa, que es responsable de la desaturación del estearato a oleato . Esta inhibición conduce a una reducción en los niveles de ácidos grasos monoinsaturados, que son componentes importantes de las membranas celulares y las moléculas de señalización. El efecto hipolipemiante del ácido 10-tiasteárico se asocia con su capacidad para modular el metabolismo lipídico y reducir la acumulación de lípidos en las células .
Compuestos similares:
Ácido esteárico: Un ácido graso saturado sin sustitución de azufre.
Ácido oleico: Un ácido graso monoinsaturado con un doble enlace pero sin sustitución de azufre.
Ácidos grasos tia: Otros ácidos grasos con sustituciones de azufre en diferentes posiciones.
Singularidad: El ácido 10-tiasteárico es único debido a su sustitución específica de azufre, que le confiere propiedades químicas y biológicas distintas. A diferencia de los ácidos esteárico y oleico, el ácido 10-tiasteárico puede inhibir el proceso de desaturación, convirtiéndolo en una herramienta valiosa para estudiar el metabolismo lipídico y desarrollar agentes terapéuticos para trastornos metabólicos .
Análisis Bioquímico
Biochemical Properties
10-Thiastearic acid interacts with enzymes involved in the desaturation of fatty acids. Specifically, it inhibits the enzyme stearoyl CoA desaturase , which is responsible for the conversion of stearate to oleate . This interaction disrupts the normal process of fatty acid desaturation, influencing the distribution of fatty acids within phospholipid pools .
Cellular Effects
In cellular processes, 10-Thiastearic acid has been observed to have a significant impact on rat hepatocytes and hepatoma cells . It inhibits the desaturation of radiolabeled stearate to oleate in these cells by more than 80% at a concentration of 25 µM . This suggests that 10-Thiastearic acid can influence cell function by altering lipid metabolism.
Molecular Mechanism
At the molecular level, 10-Thiastearic acid exerts its effects by binding to and inhibiting the enzyme stearoyl CoA desaturase . This enzyme is responsible for the conversion of stearate to oleate, a process that is crucial for the normal functioning of lipid metabolism . By inhibiting this enzyme, 10-Thiastearic acid disrupts the normal process of fatty acid desaturation.
Metabolic Pathways
10-Thiastearic acid is involved in the fatty acid metabolism pathway, specifically in the process of desaturation of stearate to oleate . It interacts with the enzyme stearoyl CoA desaturase, inhibiting its activity and thereby disrupting the normal process of fatty acid desaturation .
Métodos De Preparación
Rutas sintéticas y condiciones de reacción: El ácido 10-tiasteárico se puede sintetizar mediante la sustitución de un átomo de azufre en la cadena del ácido esteárico. La ruta sintética específica implica la reacción de octiltiol con un derivado de ácido nonanoico en condiciones controladas . La reacción generalmente requiere un catalizador y se lleva a cabo en una atmósfera inerte para evitar la oxidación.
Métodos de producción industrial: Si bien los métodos de producción industrial detallados no están ampliamente documentados, la síntesis de ácido 10-tiasteárico a mayor escala probablemente implicaría condiciones de reacción similares a las de la síntesis de laboratorio, con optimizaciones para el rendimiento y la pureza. El uso de reactores de flujo continuo y técnicas de purificación avanzadas, como la cromatografía, podría emplearse para mejorar la eficiencia de la producción.
Análisis De Reacciones Químicas
Tipos de reacciones: El ácido 10-tiasteárico sufre diversas reacciones químicas, que incluyen:
Oxidación: El átomo de azufre en el compuesto se puede oxidar para formar sulfoxidos o sulfonas.
Reducción: El grupo ácido carboxílico se puede reducir a un alcohol.
Sustitución: El átomo de azufre puede participar en reacciones de sustitución nucleofílica.
Reactivos y condiciones comunes:
Oxidación: Se pueden utilizar reactivos como peróxido de hidrógeno o ácido m-cloroperbenzoico en condiciones suaves.
Reducción: El hidruro de litio y aluminio o el borano se pueden utilizar para la reducción del grupo ácido carboxílico.
Sustitución: Los nucleófilos como los tioles o las aminas se pueden utilizar en presencia de una base.
Productos principales:
Oxidación: Sulfoxidos y sulfonas.
Reducción: Derivados de alcohol.
Sustitución: Varios tioéteres sustituidos.
Comparación Con Compuestos Similares
Stearic Acid: A saturated fatty acid with no sulfur substitution.
Oleic Acid: A monounsaturated fatty acid with a double bond but no sulfur substitution.
Thia Fatty Acids: Other fatty acids with sulfur substitutions at different positions.
Uniqueness: 10-Thiastearic acid is unique due to its specific sulfur substitution, which imparts distinct chemical and biological properties. Unlike stearic and oleic acids, 10-Thiastearic acid can inhibit the desaturation process, making it a valuable tool for studying lipid metabolism and developing therapeutic agents for metabolic disorders .
Actividad Biológica
10-Thiastearic acid (TSA), a heteroatom-substituted fatty acid, has garnered attention for its diverse biological activities, particularly in the fields of pharmacology and biochemistry. This article delves into the biological activity of TSA, highlighting its mechanisms, effects on various pathogens, and potential therapeutic applications.
10-Thiastearic acid is an analog of stearic acid, where the carbon atom at the 10th position is replaced by a sulfur atom. This modification influences its interaction with biological systems. TSA primarily acts as a fatty acid desaturase inhibitor, which plays a crucial role in lipid metabolism.
- Inhibition of Fatty Acid Desaturation : TSA has been shown to inhibit the conversion of stearate to oleate in rat hepatocytes by over 80% at a concentration of 25 µM . This inhibition can disrupt normal lipid metabolism, leading to various physiological effects.
- Hypolipidemic Effects : The compound exhibits hypolipidemic properties, making it a candidate for anti-obesity therapies . It modulates lipid profiles and may influence the distribution of fatty acids within phospholipid pools.
Biological Activity Against Pathogens
TSA has demonstrated significant antifungal and antiparasitic activities, particularly against pathogenic fungi and protozoa.
Antifungal Activity
- Histoplasma capsulatum : TSA has been tested for its inhibitory effects on H. capsulatum, a pathogenic fungus responsible for histoplasmosis. Studies indicate that TSA has a minimum inhibitory concentration (MIC) of 1.25 µM against this organism . The compound's mechanism appears to involve targeting fatty acid desaturation pathways, which are essential for fungal growth and survival.
- Lipid Metabolic Pathways : Research utilizing lipidomic analyses revealed that TSA affects sphingolipid biosynthesis pathways, which are critical for the integrity of fungal cell membranes . By disrupting these pathways, TSA reduces fungal virulence and intracellular infection rates in macrophage cell lines.
Antiparasitic Activity
TSA also exhibits activity against protozoan parasites such as Trypanosoma brucei and Leishmania spp., which rely heavily on fatty acids for energy during specific life cycle stages . The inhibition of dihydrosterculic acid biosynthesis in these organisms has been documented, with a reported 50% inhibition observed at 4 µM concentration .
Case Studies and Research Findings
Several studies have explored the biological activities of TSA in detail:
- Study on H. capsulatum : A comprehensive study highlighted TSA's role in inhibiting growth through its action on fatty acid desaturases. The study employed genetic complementation techniques to validate the pathways affected by TSA .
- Lipidomic Analysis : Research utilizing advanced lipidomic techniques showed that TSA significantly altered lipid profiles in treated cells, leading to decreased levels of critical ceramide species involved in cell signaling and membrane integrity .
- Hypolipidemic Effects : Another investigation focused on the hypolipidemic effects of TSA, demonstrating its potential as a therapeutic agent for obesity management by modulating lipid metabolism .
Data Summary Table
Biological Activity | Target Organism | Mechanism | MIC (µM) | Effect Observed |
---|---|---|---|---|
Inhibition of Fatty Acid Desaturation | Rat Hepatocytes | Inhibits conversion of stearate to oleate | 25 | >80% inhibition |
Antifungal Activity | Histoplasma capsulatum | Targets fatty acid desaturation | 1.25 | Reduced growth and intracellular infection |
Antiparasitic Activity | Trypanosoma brucei | Inhibits dihydrosterculic acid biosynthesis | 4 | 50% inhibition |
Hypolipidemic Effects | Various | Modulates lipid profiles | N/A | Potential anti-obesity therapeutic |
Propiedades
IUPAC Name |
9-octylsulfanylnonanoic acid | |
---|---|---|
Source | PubChem | |
URL | https://pubchem.ncbi.nlm.nih.gov | |
Description | Data deposited in or computed by PubChem | |
InChI |
InChI=1S/C17H34O2S/c1-2-3-4-5-9-12-15-20-16-13-10-7-6-8-11-14-17(18)19/h2-16H2,1H3,(H,18,19) | |
Source | PubChem | |
URL | https://pubchem.ncbi.nlm.nih.gov | |
Description | Data deposited in or computed by PubChem | |
InChI Key |
OMIBNTWPNMKIGZ-UHFFFAOYSA-N | |
Source | PubChem | |
URL | https://pubchem.ncbi.nlm.nih.gov | |
Description | Data deposited in or computed by PubChem | |
Canonical SMILES |
CCCCCCCCSCCCCCCCCC(=O)O | |
Source | PubChem | |
URL | https://pubchem.ncbi.nlm.nih.gov | |
Description | Data deposited in or computed by PubChem | |
Molecular Formula |
C17H34O2S | |
Source | PubChem | |
URL | https://pubchem.ncbi.nlm.nih.gov | |
Description | Data deposited in or computed by PubChem | |
DSSTOX Substance ID |
DTXSID70146944 | |
Record name | 10-Thiastearic acid | |
Source | EPA DSSTox | |
URL | https://comptox.epa.gov/dashboard/DTXSID70146944 | |
Description | DSSTox provides a high quality public chemistry resource for supporting improved predictive toxicology. | |
Molecular Weight |
302.5 g/mol | |
Source | PubChem | |
URL | https://pubchem.ncbi.nlm.nih.gov | |
Description | Data deposited in or computed by PubChem | |
CAS No. |
105099-89-6 | |
Record name | 10-Thiastearic acid | |
Source | ChemIDplus | |
URL | https://pubchem.ncbi.nlm.nih.gov/substance/?source=chemidplus&sourceid=0105099896 | |
Description | ChemIDplus is a free, web search system that provides access to the structure and nomenclature authority files used for the identification of chemical substances cited in National Library of Medicine (NLM) databases, including the TOXNET system. | |
Record name | 10-Thiastearic acid | |
Source | EPA DSSTox | |
URL | https://comptox.epa.gov/dashboard/DTXSID70146944 | |
Description | DSSTox provides a high quality public chemistry resource for supporting improved predictive toxicology. | |
Retrosynthesis Analysis
AI-Powered Synthesis Planning: Our tool employs the Template_relevance Pistachio, Template_relevance Bkms_metabolic, Template_relevance Pistachio_ringbreaker, Template_relevance Reaxys, Template_relevance Reaxys_biocatalysis model, leveraging a vast database of chemical reactions to predict feasible synthetic routes.
One-Step Synthesis Focus: Specifically designed for one-step synthesis, it provides concise and direct routes for your target compounds, streamlining the synthesis process.
Accurate Predictions: Utilizing the extensive PISTACHIO, BKMS_METABOLIC, PISTACHIO_RINGBREAKER, REAXYS, REAXYS_BIOCATALYSIS database, our tool offers high-accuracy predictions, reflecting the latest in chemical research and data.
Strategy Settings
Precursor scoring | Relevance Heuristic |
---|---|
Min. plausibility | 0.01 |
Model | Template_relevance |
Template Set | Pistachio/Bkms_metabolic/Pistachio_ringbreaker/Reaxys/Reaxys_biocatalysis |
Top-N result to add to graph | 6 |
Feasible Synthetic Routes
Q1: How does 10-Thiastearic acid exert its antifungal activity in Histoplasma capsulatum?
A1: While the exact mechanism of action isn't fully detailed in the provided abstracts, research suggests that 10-Thiastearic acid, being a fatty acid analog, likely disrupts lipid metabolism in H. capsulatum []. This disruption likely stems from its interference with fatty acid desaturases, enzymes crucial for introducing double bonds into fatty acids []. These double bonds are essential for the proper structure and function of cell membranes and other lipid-dependent processes in the fungus. By inhibiting these enzymes, 10-Thiastearic acid may lead to the accumulation of saturated fatty acids and a deficiency of unsaturated fatty acids, ultimately impairing fungal growth.
Q2: What are the potential applications of 10-Thiastearic acid in combating fungal infections?
A2: The research highlights 10-Thiastearic acid's potential as a lead compound for developing new antifungal drugs []. Its efficacy against H. capsulatum, a fungus causing the potentially fatal disease histoplasmosis, suggests it could be further explored for treating this infection []. Furthermore, its mechanism of action, targeting fatty acid desaturases, might offer a broader spectrum of activity against other fungi, making it a promising candidate for further investigation and drug development.
Descargo de responsabilidad e información sobre productos de investigación in vitro
Tenga en cuenta que todos los artículos e información de productos presentados en BenchChem están destinados únicamente con fines informativos. Los productos disponibles para la compra en BenchChem están diseñados específicamente para estudios in vitro, que se realizan fuera de organismos vivos. Los estudios in vitro, derivados del término latino "in vidrio", involucran experimentos realizados en entornos de laboratorio controlados utilizando células o tejidos. Es importante tener en cuenta que estos productos no se clasifican como medicamentos y no han recibido la aprobación de la FDA para la prevención, tratamiento o cura de ninguna condición médica, dolencia o enfermedad. Debemos enfatizar que cualquier forma de introducción corporal de estos productos en humanos o animales está estrictamente prohibida por ley. Es esencial adherirse a estas pautas para garantizar el cumplimiento de los estándares legales y éticos en la investigación y experimentación.