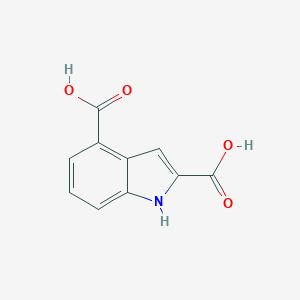
Ácido 1H-indol-2,4-dicarboxílico
Descripción general
Descripción
1H-Indole-2,4-dicarboxylic acid is an organic compound belonging to the indole family, characterized by a fused benzene and pyrrole ring structure. This compound is notable for its two carboxylic acid groups positioned at the 2 and 4 locations on the indole ring. Indole derivatives are significant in various biological and chemical contexts due to their presence in natural products and pharmaceuticals .
Aplicaciones Científicas De Investigación
1H-Indole-2,4-dicarboxylic acid has diverse applications in scientific research:
Mecanismo De Acción
Target of Action
1H-Indole-2,4-dicarboxylic acid, like many indole derivatives, has been found to bind with high affinity to multiple receptors . One specific target that has been identified is the HIV-1 integrase . This enzyme plays a critical role in the viral replication process, inserting a double-stranded viral DNA copy of the viral RNA genome into the host genome .
Mode of Action
The compound has been shown to effectively inhibit the strand transfer of HIV-1 integrase . The indole core and C2 carboxyl group of the compound chelate the two Mg2+ ions within the active site of the integrase . This interaction disrupts the enzyme’s function, inhibiting the integration of the viral DNA into the host genome .
Biochemical Pathways
Indole derivatives are metabolites produced by the metabolism of tryptophan catalyzed by intestinal microorganisms . They maintain intestinal homeostasis and impact liver metabolism and the immune response
Pharmacokinetics
It has been suggested that indole derivatives generally have high gastrointestinal absorption
Result of Action
The result of the action of 1H-Indole-2,4-dicarboxylic acid is the inhibition of the strand transfer of HIV-1 integrase, which disrupts the viral replication process . This can potentially lead to a decrease in the viral load in the body.
Action Environment
The action of 1H-Indole-2,4-dicarboxylic acid, like many other indole derivatives, can be influenced by various environmental factors. For instance, the presence of other compounds, pH levels, and temperature can affect the stability and efficacy of the compound. Additionally, the compound’s action can be influenced by the specific biological environment in which it is present, such as the presence of other metabolites or enzymes .
Métodos De Preparación
Synthetic Routes and Reaction Conditions: 1H-Indole-2,4-dicarboxylic acid can be synthesized through several methods. One common approach involves the Fischer indole synthesis, where phenylhydrazine reacts with a ketone or aldehyde under acidic conditions to form the indole structure
Industrial Production Methods: Industrial production of indole derivatives often involves catalytic processes to ensure high yield and purity. For instance, the use of ruthenium(II)-catalyzed tandem ortho-C–H functionalization reactions has been reported for synthesizing various indole derivatives .
Análisis De Reacciones Químicas
Types of Reactions: 1H-Indole-2,4-dicarboxylic acid undergoes several types of chemical reactions, including:
Oxidation: The compound can be oxidized to form quinonoid structures.
Reduction: Reduction reactions can convert the carboxylic acid groups to alcohols or other functional groups.
Substitution: Electrophilic substitution reactions are common due to the electron-rich nature of the indole ring.
Common Reagents and Conditions:
Oxidation: Reagents like potassium permanganate or chromium trioxide.
Reduction: Catalysts such as palladium on carbon (Pd/C) in the presence of hydrogen gas.
Substitution: Electrophiles like halogens or nitro groups under acidic or basic conditions.
Major Products: The major products formed from these reactions include various substituted indoles, quinonoid derivatives, and reduced forms of the compound .
Comparación Con Compuestos Similares
1H-Indole-3-carboxylic acid: Another indole derivative with a carboxylic acid group at the 3 position.
1H-Indole-2-carboxylic acid: Similar structure but with only one carboxylic acid group at the 2 position.
1H-Indole-5-carboxylic acid: Carboxylic acid group at the 5 position.
Uniqueness: 1H-Indole-2,4-dicarboxylic acid is unique due to the presence of two carboxylic acid groups, which can significantly influence its chemical reactivity and biological activity.
Actividad Biológica
1H-Indole-2,4-dicarboxylic acid (IDCA) is a compound of significant interest due to its diverse biological activities, particularly as an antiviral agent. This article explores the biological activity of IDCA, focusing on its role as an inhibitor of HIV-1 integrase, its structural modifications, and the resulting enhancements in activity.
Overview of 1H-Indole-2,4-Dicarboxylic Acid
IDCA is a derivative of indole, a bicyclic structure known for its presence in various natural products and pharmaceuticals. The dicarboxylic acid moiety enhances its potential for biological interactions, particularly in chelation with metal ions—an essential feature for many enzymatic processes.
Antiviral Properties
Recent studies have demonstrated that IDCA and its derivatives exhibit potent inhibitory effects against HIV-1 integrase, an enzyme crucial for the viral replication cycle. The mechanism involves the chelation of magnesium ions (Mg²⁺) within the active site of integrase, disrupting the enzyme's function.
-
Inhibition of Integrase Activity :
- IDCA has been shown to inhibit the strand transfer activity of HIV-1 integrase with varying potency. For instance, one study reported an IC50 value of 32.37 μM for IDCA itself .
- Structural optimizations have led to derivatives with significantly improved activities. For example, compound 17a demonstrated an IC50 value of 3.11 μM, indicating a tenfold increase in inhibitory potency compared to IDCA .
-
Structure-Activity Relationship (SAR) :
- Modifications at the C3 position of the indole core have been particularly effective in enhancing activity. The introduction of hydrophobic substituents has improved interactions with the hydrophobic cavity near the integrase active site .
- A notable derivative, compound 20a, exhibited an outstanding IC50 value of 0.13 μM due to optimized interactions with key residues in the integrase active site .
Data Table: Biological Activity of IDCA Derivatives
Compound | IC50 (μM) | Structural Modification |
---|---|---|
IDCA | 32.37 | None |
Compound 17a | 3.11 | C6 halogenated benzene |
Compound 20a | 0.13 | Long branch at C3 |
Case Study 1: Structural Optimization
In a series of experiments aimed at optimizing IDCA derivatives, researchers focused on modifying the C2 and C3 positions of the indole core. The introduction of long-chain substituents significantly improved integrase inhibition:
- Experiment : Derivatives were synthesized and evaluated for their ability to inhibit HIV-1 integrase.
- Findings : Compounds with long-chain p-trifluorophenyl or o-fluorophenyl groups at C3 showed improvements in activity by factors ranging from 5.3 to 6.5 times compared to IDCA .
Case Study 2: Molecular Docking Studies
Molecular docking studies have provided insights into how IDCA and its derivatives interact with HIV-1 integrase:
- Docking Analysis : The binding modes revealed that the indole core and carboxyl groups effectively chelate Mg²⁺ ions, establishing a critical interaction necessary for inhibitory activity.
- Results : The study highlighted that modifications at C6 could enhance π-π stacking interactions with viral DNA, further stabilizing the inhibitor-enzyme complex .
Propiedades
IUPAC Name |
1H-indole-2,4-dicarboxylic acid | |
---|---|---|
Source | PubChem | |
URL | https://pubchem.ncbi.nlm.nih.gov | |
Description | Data deposited in or computed by PubChem | |
InChI |
InChI=1S/C10H7NO4/c12-9(13)5-2-1-3-7-6(5)4-8(11-7)10(14)15/h1-4,11H,(H,12,13)(H,14,15) | |
Source | PubChem | |
URL | https://pubchem.ncbi.nlm.nih.gov | |
Description | Data deposited in or computed by PubChem | |
InChI Key |
GBQRSEVKSZQWFF-UHFFFAOYSA-N | |
Source | PubChem | |
URL | https://pubchem.ncbi.nlm.nih.gov | |
Description | Data deposited in or computed by PubChem | |
Canonical SMILES |
C1=CC(=C2C=C(NC2=C1)C(=O)O)C(=O)O | |
Source | PubChem | |
URL | https://pubchem.ncbi.nlm.nih.gov | |
Description | Data deposited in or computed by PubChem | |
Molecular Formula |
C10H7NO4 | |
Source | PubChem | |
URL | https://pubchem.ncbi.nlm.nih.gov | |
Description | Data deposited in or computed by PubChem | |
DSSTOX Substance ID |
DTXSID90564762 | |
Record name | 1H-Indole-2,4-dicarboxylic acid | |
Source | EPA DSSTox | |
URL | https://comptox.epa.gov/dashboard/DTXSID90564762 | |
Description | DSSTox provides a high quality public chemistry resource for supporting improved predictive toxicology. | |
Molecular Weight |
205.17 g/mol | |
Source | PubChem | |
URL | https://pubchem.ncbi.nlm.nih.gov | |
Description | Data deposited in or computed by PubChem | |
CAS No. |
103027-96-9 | |
Record name | 1H-Indole-2,4-dicarboxylic acid | |
Source | EPA DSSTox | |
URL | https://comptox.epa.gov/dashboard/DTXSID90564762 | |
Description | DSSTox provides a high quality public chemistry resource for supporting improved predictive toxicology. | |
Retrosynthesis Analysis
AI-Powered Synthesis Planning: Our tool employs the Template_relevance Pistachio, Template_relevance Bkms_metabolic, Template_relevance Pistachio_ringbreaker, Template_relevance Reaxys, Template_relevance Reaxys_biocatalysis model, leveraging a vast database of chemical reactions to predict feasible synthetic routes.
One-Step Synthesis Focus: Specifically designed for one-step synthesis, it provides concise and direct routes for your target compounds, streamlining the synthesis process.
Accurate Predictions: Utilizing the extensive PISTACHIO, BKMS_METABOLIC, PISTACHIO_RINGBREAKER, REAXYS, REAXYS_BIOCATALYSIS database, our tool offers high-accuracy predictions, reflecting the latest in chemical research and data.
Strategy Settings
Precursor scoring | Relevance Heuristic |
---|---|
Min. plausibility | 0.01 |
Model | Template_relevance |
Template Set | Pistachio/Bkms_metabolic/Pistachio_ringbreaker/Reaxys/Reaxys_biocatalysis |
Top-N result to add to graph | 6 |
Feasible Synthetic Routes
Descargo de responsabilidad e información sobre productos de investigación in vitro
Tenga en cuenta que todos los artículos e información de productos presentados en BenchChem están destinados únicamente con fines informativos. Los productos disponibles para la compra en BenchChem están diseñados específicamente para estudios in vitro, que se realizan fuera de organismos vivos. Los estudios in vitro, derivados del término latino "in vidrio", involucran experimentos realizados en entornos de laboratorio controlados utilizando células o tejidos. Es importante tener en cuenta que estos productos no se clasifican como medicamentos y no han recibido la aprobación de la FDA para la prevención, tratamiento o cura de ninguna condición médica, dolencia o enfermedad. Debemos enfatizar que cualquier forma de introducción corporal de estos productos en humanos o animales está estrictamente prohibida por ley. Es esencial adherirse a estas pautas para garantizar el cumplimiento de los estándares legales y éticos en la investigación y experimentación.