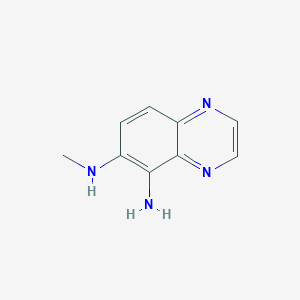
6-N-methylquinoxaline-5,6-diamine
Descripción general
Descripción
6-N-methylquinoxaline-5,6-diamine (6-N-MeQx-5,6-diamine) is an organic compound that has been studied for its potential applications in a variety of fields including pharmaceuticals, chemical synthesis, and biochemistry. 6-N-MeQx-5,6-diamine is a derivative of quinoxaline, a heterocyclic aromatic compound that is composed of two nitrogen atoms and four carbon atoms. 6-N-MeQx-5,6-diamine is a relatively new compound and has been the subject of several recent studies.
Aplicaciones Científicas De Investigación
Medicinal Chemistry
Quinoxaline derivatives have drawn immense attention in the field of medicinal chemistry due to their significant biological activities . They are considered as potential bioactive scaffolds in medicinal chemistry .
Antifungal Activity
Quinoxaline-based compounds have been reported to show antifungal activity . This makes them a potential candidate for the development of new antifungal drugs .
Antiviral Activity
Quinoxaline derivatives also exhibit antiviral properties . This suggests that they could be used in the development of antiviral medications .
Antidiabetic Activity
These compounds have been found to have antidiabetic properties . This opens up possibilities for their use in the treatment of diabetes .
Anticancer Activity
Quinoxaline derivatives have shown anticancer activity . For instance, synthesized compounds were tested against HepG2, MCF-7, PC-3, HCT-116 and HeLa cancer cell lines and showed strong EGFR inhibitory activity .
Anti-inflammatory Activity
Quinoxaline-based compounds have anti-inflammatory properties . This suggests their potential use in the treatment of inflammatory diseases .
Antibacterial Activity
These compounds have been reported to show antibacterial activity . This makes them a potential candidate for the development of new antibacterial drugs .
Antioxidant Activity
Quinoxaline derivatives also exhibit antioxidant properties . This suggests that they could be used in the development of antioxidant medications .
Mecanismo De Acción
Target of Action
N6-Methylquinoxaline-5,6-diamine is a diamine-based compound . Diamine-based compounds are known to inhibit copper-containing amine oxidases and flavoprotein polyamine oxidases . These enzymes play a crucial role in the metabolism of biogenic amines, including neurotransmitters and polyamines .
Mode of Action
The compound interacts with its targets (amine oxidases) by binding to their active sites, thereby inhibiting their activity . This inhibition can alter the normal metabolic processes regulated by these enzymes, leading to various downstream effects .
Biochemical Pathways
The inhibition of amine oxidases by N6-Methylquinoxaline-5,6-diamine can affect several biochemical pathways. For instance, the inhibition of polyamine oxidases can disrupt the normal metabolism of polyamines, which are involved in various cellular processes such as cell growth and differentiation .
Result of Action
The molecular and cellular effects of N6-Methylquinoxaline-5,6-diamine’s action largely depend on the specific biological context. By inhibiting amine oxidases, the compound can potentially influence various cellular processes regulated by these enzymes. The exact effects can vary depending on factors such as the specific cell type and the presence of other signaling molecules .
Propiedades
IUPAC Name |
6-N-methylquinoxaline-5,6-diamine | |
---|---|---|
Source | PubChem | |
URL | https://pubchem.ncbi.nlm.nih.gov | |
Description | Data deposited in or computed by PubChem | |
InChI |
InChI=1S/C9H10N4/c1-11-6-2-3-7-9(8(6)10)13-5-4-12-7/h2-5,11H,10H2,1H3 | |
Source | PubChem | |
URL | https://pubchem.ncbi.nlm.nih.gov | |
Description | Data deposited in or computed by PubChem | |
InChI Key |
ZKYWREIEORYBJU-UHFFFAOYSA-N | |
Source | PubChem | |
URL | https://pubchem.ncbi.nlm.nih.gov | |
Description | Data deposited in or computed by PubChem | |
Canonical SMILES |
CNC1=C(C2=NC=CN=C2C=C1)N | |
Source | PubChem | |
URL | https://pubchem.ncbi.nlm.nih.gov | |
Description | Data deposited in or computed by PubChem | |
Molecular Formula |
C9H10N4 | |
Source | PubChem | |
URL | https://pubchem.ncbi.nlm.nih.gov | |
Description | Data deposited in or computed by PubChem | |
Molecular Weight |
174.20 g/mol | |
Source | PubChem | |
URL | https://pubchem.ncbi.nlm.nih.gov | |
Description | Data deposited in or computed by PubChem | |
Product Name |
N6-methylquinoxaline-5,6-diamine |
Synthesis routes and methods
Procedure details
Retrosynthesis Analysis
AI-Powered Synthesis Planning: Our tool employs the Template_relevance Pistachio, Template_relevance Bkms_metabolic, Template_relevance Pistachio_ringbreaker, Template_relevance Reaxys, Template_relevance Reaxys_biocatalysis model, leveraging a vast database of chemical reactions to predict feasible synthetic routes.
One-Step Synthesis Focus: Specifically designed for one-step synthesis, it provides concise and direct routes for your target compounds, streamlining the synthesis process.
Accurate Predictions: Utilizing the extensive PISTACHIO, BKMS_METABOLIC, PISTACHIO_RINGBREAKER, REAXYS, REAXYS_BIOCATALYSIS database, our tool offers high-accuracy predictions, reflecting the latest in chemical research and data.
Strategy Settings
Precursor scoring | Relevance Heuristic |
---|---|
Min. plausibility | 0.01 |
Model | Template_relevance |
Template Set | Pistachio/Bkms_metabolic/Pistachio_ringbreaker/Reaxys/Reaxys_biocatalysis |
Top-N result to add to graph | 6 |
Feasible Synthetic Routes
Descargo de responsabilidad e información sobre productos de investigación in vitro
Tenga en cuenta que todos los artículos e información de productos presentados en BenchChem están destinados únicamente con fines informativos. Los productos disponibles para la compra en BenchChem están diseñados específicamente para estudios in vitro, que se realizan fuera de organismos vivos. Los estudios in vitro, derivados del término latino "in vidrio", involucran experimentos realizados en entornos de laboratorio controlados utilizando células o tejidos. Es importante tener en cuenta que estos productos no se clasifican como medicamentos y no han recibido la aprobación de la FDA para la prevención, tratamiento o cura de ninguna condición médica, dolencia o enfermedad. Debemos enfatizar que cualquier forma de introducción corporal de estos productos en humanos o animales está estrictamente prohibida por ley. Es esencial adherirse a estas pautas para garantizar el cumplimiento de los estándares legales y éticos en la investigación y experimentación.