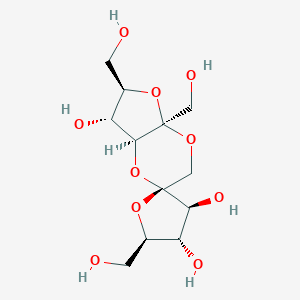
Difructose anhydride III
Descripción general
Descripción
El anhídrido de difructuosa III es un disacárido cíclico compuesto por dos residuos de fructosa. Es el disacárido cíclico más pequeño y es un hidrolisado de la inulina, un polisacárido natural. El anhídrido de difructuosa III se encuentra rara vez en la naturaleza y ha ganado interés industrial como sustituto del azúcar bajo en calorías debido a sus propiedades únicas y funciones fisiológicas .
Métodos De Preparación
Rutas de Síntesis y Condiciones de Reacción: El anhídrido de difructuosa III se produce principalmente a partir de la inulina mediante reacciones enzimáticas. La enzima fructotransferasa de inulina (despolimerizante) se utiliza para catalizar la conversión de la inulina a anhídrido de difructuosa III. Esta enzima escinde el polímero de inulina, lo que da como resultado la formación de anhídrido de difructuosa III .
Métodos de Producción Industrial: La producción a gran escala de anhídrido de difructuosa III implica el uso de fructotransferasa de inulina en biorreactores. La enzima a menudo se produce mediante microorganismos como las especies de Arthrobacter. El proceso incluye la extracción de inulina de fuentes como la achicoria, seguida de la conversión enzimática en un biorreactor de membrana de ultrafiltración para mejorar la eficiencia de la producción .
Análisis De Reacciones Químicas
Tipos de Reacciones: El anhídrido de difructuosa III experimenta varias reacciones químicas, incluida la hidrólisis y las reacciones de Maillard. Es relativamente estable en condiciones ácidas, pero se puede hidrolizar a fructosa en condiciones específicas .
Reactivos y Condiciones Comunes:
Hidrólisis: Las condiciones ácidas o enzimáticas pueden hidrolizar el anhídrido de difructuosa III a fructosa.
Reacción de Maillard: Bajo calor y en presencia de aminoácidos, el anhídrido de difructuosa III puede participar en reacciones de Maillard, lo que lleva a la formación de pigmentos marrones y compuestos de sabor.
Productos Principales:
Hidrólisis: Fructosa
Reacción de Maillard: Varios compuestos de sabor y pigmentos marrones.
Aplicaciones Científicas De Investigación
El anhídrido de difructuosa III tiene una amplia gama de aplicaciones en la investigación científica:
Química: Se utiliza como edulcorante bajo en calorías y como precursor de otros compuestos químicos.
Biología: Actúa como prebiótico, promoviendo el crecimiento de bacterias intestinales beneficiosas.
Medicina: Se ha investigado por su potencial para mejorar la absorción de minerales y reducir los niveles de colesterol.
Industria: Se utiliza en la industria alimentaria como edulcorante bajo en calorías y en la industria farmacéutica por sus beneficios para la salud
Mecanismo De Acción
El anhídrido de difructuosa III ejerce sus efectos principalmente a través de su función como prebiótico. No es digerible por las enzimas humanas, pero es fermentado por las bacterias intestinales beneficiosas, lo que lleva a la producción de ácidos grasos de cadena corta que promueven la salud intestinal. Además, mejora la absorción de minerales como el calcio y el magnesio en el intestino .
Compuestos Similares:
Anhidrido de difructuosa I: Otro disacárido cíclico formado a partir de la inulina.
Anhidrido de difructuosa IV: Producido a partir de levano, otro fructano.
Comparación: El anhídrido de difructuosa III es único debido a sus enlaces glucosídicos específicos y su capacidad para actuar como prebiótico. A diferencia del anhídrido de difructuosa I y IV, se ha demostrado que el anhídrido de difructuosa III tiene un efecto más pronunciado en la absorción de minerales y la salud intestinal .
Comparación Con Compuestos Similares
Difructose anhydride I: Another cyclic disaccharide formed from inulin.
Difructose anhydride IV: Produced from levan, another fructan.
Comparison: Difructose anhydride III is unique due to its specific glycosidic linkages and its ability to act as a prebiotic. Unlike difructose anhydride I and IV, this compound has been shown to have a more pronounced effect on mineral absorption and gut health .
Propiedades
IUPAC Name |
(2R,3'S,4'S,4aR,5'R,6R,7R,7aS)-4a,5',6-tris(hydroxymethyl)spiro[3,6,7,7a-tetrahydrofuro[2,3-b][1,4]dioxine-2,2'-oxolane]-3',4',7-triol | |
---|---|---|
Source | PubChem | |
URL | https://pubchem.ncbi.nlm.nih.gov | |
Description | Data deposited in or computed by PubChem | |
InChI |
InChI=1S/C12H20O10/c13-1-5-7(16)9(18)12(21-5)4-19-11(3-15)10(22-12)8(17)6(2-14)20-11/h5-10,13-18H,1-4H2/t5-,6-,7-,8-,9+,10+,11-,12-/m1/s1 | |
Source | PubChem | |
URL | https://pubchem.ncbi.nlm.nih.gov | |
Description | Data deposited in or computed by PubChem | |
InChI Key |
KSRQDWNGXKYIDO-TWOHWVPZSA-N | |
Source | PubChem | |
URL | https://pubchem.ncbi.nlm.nih.gov | |
Description | Data deposited in or computed by PubChem | |
Canonical SMILES |
C1C2(C(C(C(O2)CO)O)O)OC3C(C(OC3(O1)CO)CO)O | |
Source | PubChem | |
URL | https://pubchem.ncbi.nlm.nih.gov | |
Description | Data deposited in or computed by PubChem | |
Isomeric SMILES |
C1[C@]2([C@H]([C@@H]([C@H](O2)CO)O)O)O[C@H]3[C@@H]([C@H](O[C@]3(O1)CO)CO)O | |
Source | PubChem | |
URL | https://pubchem.ncbi.nlm.nih.gov | |
Description | Data deposited in or computed by PubChem | |
Molecular Formula |
C12H20O10 | |
Source | PubChem | |
URL | https://pubchem.ncbi.nlm.nih.gov | |
Description | Data deposited in or computed by PubChem | |
Molecular Weight |
324.28 g/mol | |
Source | PubChem | |
URL | https://pubchem.ncbi.nlm.nih.gov | |
Description | Data deposited in or computed by PubChem | |
CAS No. |
81129-73-9 | |
Record name | Difructose anhydride III | |
Source | ChemIDplus | |
URL | https://pubchem.ncbi.nlm.nih.gov/substance/?source=chemidplus&sourceid=0081129739 | |
Description | ChemIDplus is a free, web search system that provides access to the structure and nomenclature authority files used for the identification of chemical substances cited in National Library of Medicine (NLM) databases, including the TOXNET system. | |
Retrosynthesis Analysis
AI-Powered Synthesis Planning: Our tool employs the Template_relevance Pistachio, Template_relevance Bkms_metabolic, Template_relevance Pistachio_ringbreaker, Template_relevance Reaxys, Template_relevance Reaxys_biocatalysis model, leveraging a vast database of chemical reactions to predict feasible synthetic routes.
One-Step Synthesis Focus: Specifically designed for one-step synthesis, it provides concise and direct routes for your target compounds, streamlining the synthesis process.
Accurate Predictions: Utilizing the extensive PISTACHIO, BKMS_METABOLIC, PISTACHIO_RINGBREAKER, REAXYS, REAXYS_BIOCATALYSIS database, our tool offers high-accuracy predictions, reflecting the latest in chemical research and data.
Strategy Settings
Precursor scoring | Relevance Heuristic |
---|---|
Min. plausibility | 0.01 |
Model | Template_relevance |
Template Set | Pistachio/Bkms_metabolic/Pistachio_ringbreaker/Reaxys/Reaxys_biocatalysis |
Top-N result to add to graph | 6 |
Feasible Synthetic Routes
Descargo de responsabilidad e información sobre productos de investigación in vitro
Tenga en cuenta que todos los artículos e información de productos presentados en BenchChem están destinados únicamente con fines informativos. Los productos disponibles para la compra en BenchChem están diseñados específicamente para estudios in vitro, que se realizan fuera de organismos vivos. Los estudios in vitro, derivados del término latino "in vidrio", involucran experimentos realizados en entornos de laboratorio controlados utilizando células o tejidos. Es importante tener en cuenta que estos productos no se clasifican como medicamentos y no han recibido la aprobación de la FDA para la prevención, tratamiento o cura de ninguna condición médica, dolencia o enfermedad. Debemos enfatizar que cualquier forma de introducción corporal de estos productos en humanos o animales está estrictamente prohibida por ley. Es esencial adherirse a estas pautas para garantizar el cumplimiento de los estándares legales y éticos en la investigación y experimentación.