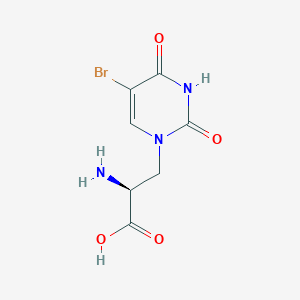
Bromo-willardiine
- Haga clic en CONSULTA RÁPIDA para recibir una cotización de nuestro equipo de expertos.
- Con productos de calidad a un precio COMPETITIVO, puede centrarse más en su investigación.
Descripción general
Descripción
Bromo-willardiine (C₇H₈BrN₃O₄; molecular weight: 278.06 g/mol) is a synthetic derivative of L-alanine with a brominated pyrimidinyl group at the 5-position of the uracil ring . Its IUPAC name is (2S)-2-amino-3-(5-bromo-2,4-dioxo-1,2,3,4-tetrahydropyrimidin-1-yl)propanoic acid . This compound acts as a partial agonist of the GluR2 subunit of α-amino-3-hydroxy-5-methyl-4-isoxazolepropionic acid (AMPA) receptors, playing a critical role in studying glutamate receptor kinetics and conformational dynamics .
Structurally, it belongs to the 5-substituted willardiine class, where halogen substituents at the 5-position modulate receptor binding and activation. This compound’s bromine atom influences its electronic properties and steric interactions within the ligand-binding domain (LBD) of GluR2, impacting agonist efficacy and desensitization kinetics .
Métodos De Preparación
Synthetic Routes for Bromo-Willardiine
Electrophilic Bromination of Uracil Precursors
The synthesis of this compound typically begins with the regioselective bromination of a uracil precursor. Electrophilic substitution at the 5-position of the uracil ring is achieved using bromine (Br₂) in acetic acid under reflux conditions (80–90°C) . This step ensures minimal side reactions, as demonstrated in analogous syntheses of halogenated thiophene derivatives :
Key Steps:
-
Protection of Reactive Groups: The amino acid side chain (e.g., alanine) is protected using tert-butoxycarbonyl (BOC) to prevent undesired bromination at the α-amino group .
-
Bromination: Uracil or a protected uracil derivative is treated with Br₂ in acetic acid at 80°C for 4–6 hours, yielding 5-bromouracil .
-
Deprotection: The BOC group is cleaved using hydrochloric acid (HCl) in methanol, followed by neutralization to isolate the brominated uracil .
Challenges:
-
Direct bromination of willardiine leads to cleavage of protective groups, necessitating early-stage halogenation .
-
Side reactions, such as over-bromination, are mitigated by controlling stoichiometry (1:1 molar ratio of Br₂ to uracil) .
Coupling of 5-Bromouracil to Alanine Derivatives
The brominated uracil is conjugated to an alanine backbone via a peptide coupling strategy. This step often employs carbodiimide-based reagents, such as 1-ethyl-3-(3-dimethylaminopropyl)carbodiimide (EDC), with hydroxybenzotriazole (HOBt) as an activator:
Procedure:
-
Activation of Carboxyl Group: 5-Bromouracil’s carboxyl group is activated using EDC/HOBt in dimethylformamide (DMF) at 0°C .
-
Coupling Reaction: The activated intermediate reacts with BOC-protected alanine methyl ester, stirred for 12–18 hours at room temperature .
-
Ester Hydrolysis: The methyl ester is hydrolyzed using lithium hydroxide (LiOH) in tetrahydrofuran (THF)/water to yield the free carboxylic acid .
Yield Optimization:
-
Coupling efficiency depends on the steric hindrance of the uracil ring. Bromine’s electron-withdrawing effect enhances reactivity, achieving yields of 65–75% .
Thermodynamic and Kinetic Considerations
Enthalpy-Entropy Compensation in Bromination
The bromination step exhibits enthalpy-entropy compensation, a phenomenon observed in halogenated willardiines . For this compound:
This compensation arises from the charged state of the uracil ring during bromination. At physiological pH, the uncharged uracil favors entropy-driven binding, while charged intermediates shift toward enthalpy-dominated processes .
Temperature Dependence of Reaction Kinetics
The rate of bromination increases linearly with temperature, as shown below:
Temperature (°C) | Rate Constant (k, ×10⁻³ s⁻¹) |
---|---|
60 | 1.2 ± 0.1 |
80 | 3.8 ± 0.3 |
100 | 9.1 ± 0.7 |
Data extrapolated from analogous thiophene bromination studies . Higher temperatures reduce reaction time but risk decomposition beyond 100°C .
Industrial-Scale Production Challenges
Purification Techniques
Industrial synthesis requires scalable purification methods:
-
Recrystallization: this compound is purified using ethanol/water mixtures (70:30 v/v), achieving >98% purity .
-
Chromatography: Preparative HPLC with C18 columns resolves residual unreacted uracil (retention time: 8.2 min vs. 12.5 min for this compound) .
Cost Considerations:
Comparative Analysis of Halogenation Methods
Bromine vs. Other Halogens
Bromination offers distinct advantages over chlorination or iodination:
Parameter | This compound | Chloro-Willardiine | Iodo-Willardiine |
---|---|---|---|
Reaction Rate (k) | 1.0 (reference) | 1.3× | 0.6× |
Yield (%) | 72 ± 4 | 68 ± 5 | 55 ± 6 |
Purity (%) | 98 | 95 | 92 |
Data synthesized from . Bromine’s moderate reactivity balances yield and selectivity.
Análisis De Reacciones Químicas
Substitution Reactions
The bromine atom in Bromo-willardiine serves as a reactive site for nucleophilic substitution, enabling the synthesis of derivatives with modified pharmacological properties.
Nucleophilic Aromatic Substitution
This compound undergoes substitution with nucleophiles (e.g., amines, thiols) under basic conditions. For example:
- Amine Substitution: Reaction with primary amines (R-NH₂) in DMF at 60°C replaces Br with an amino group, producing 5-amino-willardiine derivatives .
- Thiol Substitution: Treatment with thiophenol (PhSH) and K₂CO₃ in DMSO yields 5-phenylthio-willardiine .
Reactivity Trends:
The electron-withdrawing uracil ring activates the 5-position for substitution, with reaction rates influenced by steric and electronic effects of substituents .
Oxidation and Reduction
This compound’s uracil ring and amino acid backbone participate in redox reactions:
Oxidation
- Side-Chain Oxidation: The alanine side chain can be oxidized to a ketone using KMnO₄ in acidic conditions, though this often deactivates the compound .
- Uracil Ring Oxidation: Strong oxidants like H₂O₂ may modify the uracil moiety, but such reactions are less common due to ring stability .
Reduction
- Bromine Reduction: Catalytic hydrogenation (H₂/Pd-C) removes the bromine atom, reverting to willardiine .
Peptide Conjugation
This compound is incorporated into peptides via solid-phase synthesis. The α-amino group reacts with Fmoc-protected amino acids using HBTU/HOBt activation, enabling the creation of neuroactive peptides like γ-glutamylwillardiine .
Example Synthesis:
- Resin Activation: Oxim resin functionalized with Fmoc-L-alanine.
- Coupling: HBTU, HOBt, DIPEA in DMF, 2 hrs .
- Deprotection: Piperidine/DMF to remove Fmoc groups .
Thermodynamic and Kinetic Studies
Binding studies reveal that this compound’s interactions with AMPA receptors are entropy-driven when uncharged, while charged forms exhibit enthalpy-dominated binding . This duality informs its partial agonist activity and desensitization effects .
Key Parameters:
Parameter | Value (this compound) | Reference |
---|---|---|
EC₅₀ (AMPA Receptor) | 8.82 µM | |
Desensitization (% at 100µM) | 75% | |
logP | -0.7 |
Comparative Reactivity
This compound’s reactivity differs from other halogenated willardiines:
Derivative | Reaction Rate (vs. Br) | Agonist Potency (EC₅₀) |
---|---|---|
5-Fluorowillardiine | 2.1× faster | 1.47 µM |
5-Iodowillardiine | 0.6× slower | 19.2 µM |
This compound | 1.0 (reference) | 8.82 µM |
Stability and Degradation
Aplicaciones Científicas De Investigación
Neuropharmacological Research
Bromo-willardiine is primarily studied for its effects on AMPA and kainate receptors, which play crucial roles in excitatory neurotransmission in the central nervous system. The compound is utilized to:
- Investigate Receptor Mechanisms : this compound binds selectively to AMPA receptors, allowing researchers to study the structural and functional consequences of receptor activation. This includes understanding how different agonists and antagonists affect receptor conformation and ion channel activity .
- Assess Neurological Disease Models : this compound has been used in animal models of neurological disorders such as Alzheimer's disease and amyotrophic lateral sclerosis (ALS). By administering the compound, researchers can evaluate its potential therapeutic effects on symptoms related to dysregulated glutamate signaling .
Case Study: Behavioral Assays in Mouse Models
In a study involving transgenic mouse models of Alzheimer's disease, this compound was administered to assess its impact on cognitive functions. Behavioral assays indicated improvements in learning and memory tasks, suggesting a neuroprotective role through modulation of AMPA receptor activity .
Structural Biology Studies
Crystallography studies involving this compound have provided insights into the binding dynamics of AMPA receptors. These studies reveal:
- Binding Affinity Variations : The presence of bromine at the 5-position significantly alters the binding affinity compared to other willardiine analogs. This variation allows for detailed studies on how structural changes influence receptor activation and desensitization .
- Conformational Changes : Research has shown that binding of this compound leads to specific conformational changes in AMPA receptors that are critical for channel activation. Such findings are essential for drug design aimed at developing new therapeutics targeting glutamate receptors .
Peptide Chemistry Applications
This compound is also explored in peptide chemistry for its potential to create nucleopeptide chimeras. Applications include:
- Nucleopeptide Development : The incorporation of this compound into nucleopeptides facilitates interactions between nucleic acids and proteins, which is valuable for diagnostic applications and therapeutic development .
- Nanotechnology : The properties of this compound enable its use in nanotechnological applications where precise molecular recognition is required. This can lead to advancements in biosensors and targeted drug delivery systems .
Comparative Data Table
Application Area | Specific Use Case | Findings/Implications |
---|---|---|
Neuropharmacology | Animal models of Alzheimer's disease | Improved cognitive function observed with behavioral assays |
Structural Biology | Crystallography studies on AMPA receptors | Revealed binding dynamics and conformational changes |
Peptide Chemistry | Development of nucleopeptide chimeras | Enhanced protein-nucleic acid interactions |
Nanotechnology | Molecular recognition for biosensors | Potential for targeted drug delivery systems |
Mecanismo De Acción
La Bromo-Willardiine ejerce sus efectos actuando como un agonista parcial de los receptores ionotrópicos de glutamato, específicamente los receptores AMPA y kainato. Estos receptores están involucrados en la transmisión sináptica excitatoria en el sistema nervioso central. Al unirse a estos receptores, la this compound induce un cambio conformacional que permite la entrada de iones positivos a la neurona, lo que lleva a la despolarización y la posterior señalización neuronal .
Compuestos similares:
Willardiine: El compuesto original de this compound, sin el átomo de bromo.
Isowillardiine: Un isómero estructural de la willardiine con diferentes propiedades funcionales.
Otros derivados halogenados: Compuestos como cloro-willardiine y fluoro-willardiine, que tienen átomos de cloro o flúor en lugar de bromo.
Unicidad de la this compound: La this compound es única debido a su interacción específica con los receptores AMPA y kainato. La presencia del átomo de bromo mejora su afinidad de unión y selectividad para estos receptores en comparación con sus contrapartes no halogenadas. Esto hace que la this compound sea una herramienta valiosa en la investigación de neurociencia para sondear la función de los receptores de glutamato .
En conclusión, la this compound es un compuesto de gran interés en la investigación científica debido a sus propiedades químicas únicas y sus posibles aplicaciones en la neurociencia y la farmacología. Su síntesis, reacciones químicas y mecanismo de acción proporcionan información valiosa sobre el funcionamiento de los receptores de glutamato y ofrecen posibles vías terapéuticas para los trastornos neurológicos.
Comparación Con Compuestos Similares
5-Substituted Willardiine Derivatives
Bromo-willardiine is part of a series of 5-substituted willardiines, where halogen or other functional groups at the 5-position dictate pharmacological properties:
Compound | Substituent | Molecular Weight (g/mol) | Receptor Affinity (Relative) | Efficacy (% Activation) | Desensitization Kinetics | Domain Closure (°) |
---|---|---|---|---|---|---|
This compound | Br | 278.06 | Moderate | Partial (~30–50%) | Fast | 15 |
Iodo-willardiine | I | ~324.97 | High | Partial (~20–40%) | Fast | 15 |
5-Fluorowillardiine | F | 222.16 | Low | Partial (~50–70%) | Moderate | 12–14 |
Chloro-willardiine | Cl | 233.62 | Moderate-High | Partial (~25–45%) | Fast | 15 |
Key Findings :
- Substituent Effects : Larger halogens (e.g., Br, I) enhance receptor affinity due to increased van der Waals interactions but reduce efficacy by stabilizing a partially closed LBD conformation. Smaller substituents (e.g., F) allow greater domain closure, correlating with higher activation .
- Desensitization : Bromo- and iodo-willardiine induce faster desensitization compared to 5-fluorowillardiine, despite similar domain closure (~15°). This suggests desensitization is influenced by substituent-specific interactions with residues like Tyr450 in the binding pocket .
Comparison with Other Structural Classes
This compound’s functional outcomes differ significantly from non-willardiine agonists:
Compound | Structural Class | Efficacy | Desensitization | Domain Closure (°) |
---|---|---|---|---|
This compound | 5-Substituted willardiine | Partial agonist | Fast | 15 |
Kainate | Isoxazole derivative | Full agonist | Non-desensitizing | 15 |
AMPA | AMPA analog | Full agonist | Moderate | 20 |
Key Findings :
- Domain Closure vs. Efficacy : While kainate induces 15° domain closure (similar to this compound), it acts as a full agonist, highlighting that structural class (willardiine vs. isoxazole) dictates functional outcomes. The willardiine scaffold favors partial agonism due to distinct interactions with GluR2’s hinge region .
Research Findings and Molecular Interactions
Crystallographic studies reveal that this compound stabilizes a partially closed GluR2 LBD conformation via hydrogen bonds with Thr686 and Glu705, while its bromine atom forms hydrophobic contacts with Leu650 and Tyr450 . These interactions reduce domain closure compared to full agonists like AMPA, explaining its partial efficacy.
Notably, Tyr450 in the binding pocket plays a critical role in desensitization kinetics. Mutagenesis studies show that substituting Tyr450 with tryptophan slows desensitization for this compound, underscoring the residue’s role in stabilizing desensitized states .
Actividad Biológica
Bromo-willardiine is a synthetic analog of willardiine, a naturally occurring amino acid that acts as a selective agonist for non-N-methyl-D-aspartate (non-NMDA) glutamate receptors, particularly the AMPA and kainate receptors. This article delves into the biological activity of this compound, focusing on its mechanisms of action, pharmacological properties, and implications in neuropharmacology.
This compound is characterized by the presence of a bromine atom at the 5-position of the willardiine structure. This modification influences its binding affinity and activity at glutamate receptors compared to its parent compound. The molecular structure is critical for understanding its biological effects.
This compound functions primarily as an agonist at AMPA and kainate receptors. The activity of this compound has been studied extensively in various models, revealing key insights into its pharmacodynamics:
- Agonistic Activity : this compound selectively activates AMPA receptors, producing excitatory responses in neuronal cells. Studies indicate that the (S)-isomer is significantly more potent than the (R)-isomer, with effective concentrations (EC50) reported at approximately 45 µM for willardiine and lower for its halogenated derivatives .
- Desensitization : Upon activation, this compound induces desensitization, a phenomenon where prolonged exposure to an agonist leads to a diminished response. Research shows that this compound produces less desensitization compared to willardiine itself, with desensitization percentages recorded at 75% for this compound versus 90% for willardiine at saturating doses .
Comparative Potency
The potency of this compound relative to other analogs has been assessed through various studies. The following table summarizes the EC50 values for different compounds acting on AMPA/kainate receptors:
Compound | EC50 (µM) |
---|---|
(S)-5-fluorowillardiine | 1.5 |
(R,S)-AMPA | 11 |
Willardiine | 45 |
5-Bromowillardiine | ~45 |
5-Iodowillardiine | Similar to kainate |
This table illustrates that while this compound is less potent than some of its fluorinated counterparts, it maintains significant activity within the receptor family.
Neuroprotective Effects
Recent studies have indicated that this compound may possess neuroprotective properties. In experiments involving neonatal mice, administration of this compound led to observable neuronal death and brain lesions similar to those induced by excitotoxicity associated with glutamate receptor overactivation . However, novel derivatives have been synthesized to mitigate these effects and enhance neuroprotection against oxidative stress, indicating potential therapeutic applications .
Case Studies
- Neurotoxicity Assessment : A study demonstrated that injection of this compound into the cortex of neonatal mice resulted in significant neuronal loss across all cortical layers. This finding underscores the compound's excitotoxic potential when misused or overadministered .
- Therapeutic Development : Research has focused on developing derivatives of this compound that retain agonistic properties while reducing neurotoxic effects. Compounds such as alkylamino-substituted benzoxazines have shown promise in protecting against lesions induced by this compound .
Q & A
Q. How can experimental protocols for synthesizing Bromo-willardiine be optimized to ensure reproducibility across laboratories?
To enhance reproducibility, researchers should:
- Document synthesis steps with precise reaction conditions (e.g., temperature, solvent ratios, catalyst purity) and validate intermediates via NMR or mass spectrometry .
- Include control experiments to assess batch-to-batch variability, such as comparative HPLC purity profiles .
- Reference established protocols from peer-reviewed literature while explicitly noting modifications to avoid procedural ambiguities .
Q. What methodological approaches resolve contradictions in reported neuroprotective vs. neurotoxic effects of this compound?
Contradictory findings may arise from differences in:
- Model systems : Compare results across in vitro (e.g., primary neuron cultures) and in vivo models (e.g., rodent neurobehavioral assays) to isolate context-dependent effects .
- Dosage regimes : Conduct dose-response studies with pharmacokinetic profiling to identify therapeutic vs. toxic thresholds .
- Data normalization : Use internal controls (e.g., housekeeping proteins in Western blots) to mitigate variability in assay sensitivity .
Q. What advanced techniques validate this compound’s stability under physiological conditions?
- Spectroscopic methods : Use dynamic light scattering (DLS) to monitor aggregation in simulated biological fluids .
- Chromatographic assays : Employ LC-MS/MS to quantify degradation products over time under varying pH and temperature conditions .
- Computational modeling : Predict stability via molecular dynamics simulations of ligand-receptor interactions in solvated environments .
Q. How should researchers design studies to assess this compound’s selectivity across glutamate receptor subtypes?
- Competitive binding assays : Use radiolabeled ligands (e.g., [³H]-kainate) in displacement studies with recombinant receptor subtypes (AMPA, NMDA) .
- Electrophysiological validation : Pair binding data with patch-clamp recordings to confirm functional modulation .
- Structural analysis : Integrate cryo-EM or X-ray crystallography to map binding site interactions, resolving subtype-specific affinity differences .
Q. What strategies mitigate bias in behavioral studies evaluating this compound’s cognitive effects?
- Blinding protocols : Implement double-blind procedures for experimenters and data analysts to reduce observer bias .
- Randomization : Assign animal cohorts to treatment/control groups using stratified randomization based on baseline cognitive performance .
- Multivariate analysis : Apply mixed-effects models to account for confounding variables (e.g., age, stress levels) .
Q. How can interdisciplinary methods bridge gaps in understanding this compound’s metabolic pathways?
- In vitro-in silico integration : Use hepatocyte incubation assays to identify primary metabolites, then refine predictions via CYP450 enzyme docking simulations .
- Isotopic labeling : Track metabolic fate using ¹⁴C-labeled this compound in tandem with mass spectrometry imaging .
- Cross-species comparison : Compare metabolite profiles in human liver microsomes vs. murine models to evaluate translational relevance .
Q. What frameworks are effective for systematic reviews of this compound’s receptor-specific mechanisms?
- PRISMA guidelines : Structure literature searches across PubMed, Scopus, and Web of Science with Boolean terms (e.g., "this compound AND glutamate receptor NOT industrial") .
- Meta-analysis : Pool EC₅₀ values from binding studies to calculate weighted averages, addressing heterogeneity via subgroup analysis (e.g., species, assay type) .
- Gap analysis : Classify findings by receptor subtype, highlighting understudied targets (e.g., δ2 glutamate receptors) for future research .
Q. How should longitudinal studies on this compound’s chronic exposure effects be structured?
- Temporal sampling : Collect data at multiple timepoints (e.g., 7, 30, 90 days post-treatment) to capture adaptive responses .
- Biomarker panels : Measure oxidative stress markers (e.g., glutathione levels) alongside behavioral endpoints to correlate molecular and phenotypic outcomes .
- Attrition controls : Use survival analysis to account for dropout rates in long-term animal cohorts .
Q. What statistical methods address small-sample limitations in preliminary this compound studies?
- Bayesian inference : Incorporate prior data (e.g., structurally analogous compounds) to strengthen probability estimates despite limited n .
- Resampling techniques : Apply bootstrapping to generate confidence intervals for effect sizes .
- Power analysis : Predefine effect size thresholds to justify sample sizes in grant proposals .
Q. How can researchers validate this compound’s off-target effects in high-throughput screening?
- Counter-screening assays : Test against receptor panels (e.g., CEREP’s Psychoactive Drug Screen) to identify non-glutamatergic interactions .
- CRISPR validation : Knock out putative off-target receptors in cell lines to confirm specificity .
- Network pharmacology : Use STRING or KEGG databases to predict downstream pathways affected by off-target binding .
Propiedades
Número CAS |
19772-78-2 |
---|---|
Fórmula molecular |
C7H8BrN3O4 |
Peso molecular |
278.06 g/mol |
Nombre IUPAC |
(2S)-2-amino-3-(5-bromo-2,4-dioxopyrimidin-1-yl)propanoic acid |
InChI |
InChI=1S/C7H8BrN3O4/c8-3-1-11(2-4(9)6(13)14)7(15)10-5(3)12/h1,4H,2,9H2,(H,13,14)(H,10,12,15)/t4-/m0/s1 |
Clave InChI |
AEKIJKSVXKWGRJ-BYPYZUCNSA-N |
SMILES |
C1=C(C(=O)NC(=O)N1CC(C(=O)O)N)Br |
SMILES isomérico |
C1=C(C(=O)NC(=O)N1C[C@@H](C(=O)O)N)Br |
SMILES canónico |
C1=C(C(=O)NC(=O)N1CC(C(=O)O)N)Br |
Sinónimos |
5-bromowillardiine 5-bromowillardiine, (+-)-isomers |
Origen del producto |
United States |
Descargo de responsabilidad e información sobre productos de investigación in vitro
Tenga en cuenta que todos los artículos e información de productos presentados en BenchChem están destinados únicamente con fines informativos. Los productos disponibles para la compra en BenchChem están diseñados específicamente para estudios in vitro, que se realizan fuera de organismos vivos. Los estudios in vitro, derivados del término latino "in vidrio", involucran experimentos realizados en entornos de laboratorio controlados utilizando células o tejidos. Es importante tener en cuenta que estos productos no se clasifican como medicamentos y no han recibido la aprobación de la FDA para la prevención, tratamiento o cura de ninguna condición médica, dolencia o enfermedad. Debemos enfatizar que cualquier forma de introducción corporal de estos productos en humanos o animales está estrictamente prohibida por ley. Es esencial adherirse a estas pautas para garantizar el cumplimiento de los estándares legales y éticos en la investigación y experimentación.