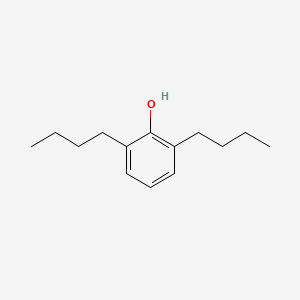
2,6-Dibutylphenol
- Klicken Sie auf QUICK INQUIRY, um ein Angebot von unserem Expertenteam zu erhalten.
- Mit qualitativ hochwertigen Produkten zu einem WETTBEWERBSFÄHIGEN Preis können Sie sich mehr auf Ihre Forschung konzentrieren.
Beschreibung
2,6-Dibutylphenol is a useful research compound. Its molecular formula is C14H22O and its molecular weight is 206.32 g/mol. The purity is usually 95%.
BenchChem offers high-quality this compound suitable for many research applications. Different packaging options are available to accommodate customers' requirements. Please inquire for more information about this compound including the price, delivery time, and more detailed information at info@benchchem.com.
Eigenschaften
CAS-Nummer |
62083-20-9 |
---|---|
Molekularformel |
C14H22O |
Molekulargewicht |
206.32 g/mol |
IUPAC-Name |
2,6-dibutylphenol |
InChI |
InChI=1S/C14H22O/c1-3-5-8-12-10-7-11-13(14(12)15)9-6-4-2/h7,10-11,15H,3-6,8-9H2,1-2H3 |
InChI-Schlüssel |
PZPVXSMCRLNVRD-UHFFFAOYSA-N |
Kanonische SMILES |
CCCCC1=C(C(=CC=C1)CCCC)O |
Herkunft des Produkts |
United States |
Haftungsausschluss und Informationen zu In-Vitro-Forschungsprodukten
Bitte beachten Sie, dass alle Artikel und Produktinformationen, die auf BenchChem präsentiert werden, ausschließlich zu Informationszwecken bestimmt sind. Die auf BenchChem zum Kauf angebotenen Produkte sind speziell für In-vitro-Studien konzipiert, die außerhalb lebender Organismen durchgeführt werden. In-vitro-Studien, abgeleitet von dem lateinischen Begriff "in Glas", beinhalten Experimente, die in kontrollierten Laborumgebungen unter Verwendung von Zellen oder Geweben durchgeführt werden. Es ist wichtig zu beachten, dass diese Produkte nicht als Arzneimittel oder Medikamente eingestuft sind und keine Zulassung der FDA für die Vorbeugung, Behandlung oder Heilung von medizinischen Zuständen, Beschwerden oder Krankheiten erhalten haben. Wir müssen betonen, dass jede Form der körperlichen Einführung dieser Produkte in Menschen oder Tiere gesetzlich strikt untersagt ist. Es ist unerlässlich, sich an diese Richtlinien zu halten, um die Einhaltung rechtlicher und ethischer Standards in Forschung und Experiment zu gewährleisten.