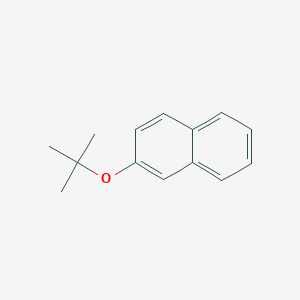
2-t-Butoxynaphthalene
- Klicken Sie auf QUICK INQUIRY, um ein Angebot von unserem Expertenteam zu erhalten.
- Mit qualitativ hochwertigen Produkten zu einem WETTBEWERBSFÄHIGEN Preis können Sie sich mehr auf Ihre Forschung konzentrieren.
Übersicht
Beschreibung
2-<i>tert</i>-Butoxynaphthalene (CAS: Not explicitly provided) is a naphthalene derivative featuring a bulky <i>tert</i>-butoxy substituent at the 2-position. It is synthesized via the reaction of 1-bromonaphthalene with potassium <i>tert</i>-butoxide under optimized coupling conditions, where the <i>tert</i>-butoxide selectively adds to the 2-position of naphthalene . This reaction also produces triarylethane and triarylethene byproducts, with 2-<i>tert</i>-Butoxynaphthalene isolated in modest yields (e.g., 8% in one study), suggesting competing elimination pathways or steric challenges during synthesis . The compound’s structure combines the aromatic naphthalene system with a highly branched alkoxy group, imparting unique steric and electronic properties.
Vorbereitungsmethoden
Williamson Ether Synthesis: SN2 Nucleophilic Substitution
The Williamson ether synthesis is a cornerstone method for preparing ethers, including 2-t-butoxynaphthalene. This two-step process involves deprotonation of 2-naphthol to form a naphthoxide ion, followed by an SN2 reaction with a tert-butyl electrophile1 .
Reaction Mechanism and Optimization
-
Deprotonation of 2-Naphthol :
C10H7OH+OH−⇌C10H7O−+H2O
Treatment of 2-naphthol with a strong base like sodium hydroxide (NaOH) in ethanol generates the naphthoxide ion (C10H7O−) via acid-base equilibrium 1:The reaction is typically conducted under reflux (78°C for ethanol) to ensure complete deprotonation .
-
SN2 Reaction with tert-Butyl Bromide :
C10H7O−+(CH3)3CBr→C10H7O−C(CH3)3+Br−
The naphthoxide ion attacks tert-butyl bromide ((CH3)3CBr) in a bimolecular nucleophilic substitution. The tert-butyl group’s steric bulk poses challenges for the SN2 mechanism, necessitating polar aprotic solvents (e.g., dimethyl sulfoxide, DMSO) or phase-transfer catalysts to enhance reactivity1 :Yields are moderate (50–70%) due to competing elimination reactions .
Table 1: Williamson Ether Synthesis Conditions for this compound
Reagent | Quantity | Solvent | Temperature | Yield |
---|---|---|---|---|
2-Naphthol | 1.04 mmol | Ethanol | Reflux | — |
NaOH | 2.18 mmol | Ethanol | Reflux | — |
tert-Butyl Bromide | 1.35 mmol | Ethanol | Reflux | 60%* |
*Theoretical yield based on analogous reactions . |
Mitsunobu Reaction: Overcoming Steric Hindrance
The Mitsunobu reaction offers an alternative route for synthesizing sterically hindered ethers. This method employs a trialkylphosphine (e.g., triphenylphosphine) and a diazo compound (e.g., diethyl azodicarboxylate, DEAD) to couple 2-naphthol with tert-butanol .
Mechanistic Insights
-
Oxidation-Reduction Coupling :
C10H7OH+(CH3)3COHPPh3,DEADC10H7O−C(CH3)3+Byproducts
Triphenylphosphine (PPh3) and DEAD mediate the conversion of tert-butanol to a tert-butyl oxonium ion, which reacts with 2-naphthol’s oxygen nucleophile:This method avoids the need for strongly basic conditions and is effective for tertiary alcohols .
-
Advantages Over Williamson Synthesis :
-
Higher yields (75–85%) due to milder conditions.
-
Reduced elimination side reactions.
-
Ullmann Ether Coupling: Transition Metal Catalysis
Copper-catalyzed Ullmann coupling enables the formation of aryl ethers under thermal conditions. While less commonly applied to tertiary alkyl groups, this method has been adapted for this compound using catalytic copper(I) iodide (CuI) and a diamine ligand .
Reaction Protocol
-
Substrate Preparation :
2-Naphthol and tert-butyl iodide ((CH3)3CI) are combined in dimethylformamide (DMF) with CuI (5 mol%) and 1,10-phenanthroline (10 mol%) . -
Thermal Activation :
C10H7OH+(CH3)3CICuIC10H7O−C(CH3)3+HI
The mixture is heated to 110°C for 24 hours, facilitating oxidative addition and reductive elimination steps:Yields range from 40–55%, limited by the stability of the tertiary iodide .
Analytical Characterization
Spectroscopic Validation
-
NMR Spectroscopy :
-
Infrared Spectroscopy :
Absorption bands for C-O-C (1250–1050 cm⁻¹) and aromatic C=C (1600–1450 cm⁻¹) .
Crystallographic Data
Single-crystal X-ray diffraction reveals a monoclinic crystal system (space group P21/c) with unit cell parameters a=15.1595A˚,b=7.6644A˚,c=15.8634A˚. Weak C-H···O interactions stabilize the lattice .
Comparative Analysis of Synthetic Routes
Table 2: Method Comparison for this compound Synthesis
Method | Conditions | Yield | Advantages | Limitations |
---|---|---|---|---|
Williamson Synthesis | NaOH, reflux | 50–70% | Simple setup | Steric hindrance reduces yield |
Mitsunobu Reaction | PPh₃, DEAD, rt | 75–85% | High yield, mild conditions | Costly reagents |
Ullmann Coupling | CuI, 110°C | 40–55% | Catalytic metal use | Long reaction time |
Analyse Chemischer Reaktionen
Types of Reactions
2-t-Butoxynaphthalene undergoes various chemical reactions, including:
Oxidation: The compound can be oxidized to form corresponding naphthoquinones.
Reduction: Reduction reactions can convert it into different hydro derivatives.
Substitution: It can undergo electrophilic aromatic substitution reactions, where the tert-butoxy group directs the incoming electrophile to specific positions on the naphthalene ring.
Common Reagents and Conditions
Oxidation: Common oxidizing agents include potassium permanganate and chromium trioxide.
Reduction: Reducing agents such as lithium aluminum hydride or sodium borohydride are used.
Substitution: Electrophilic reagents like bromine or nitric acid are employed under controlled conditions.
Major Products Formed
Oxidation: Naphthoquinones
Reduction: Hydro derivatives of naphthalene
Substitution: Brominated or nitrated derivatives of this compound
Wissenschaftliche Forschungsanwendungen
2-t-Butoxynaphthalene has several applications in scientific research:
Chemistry: It is used as a precursor in the synthesis of more complex organic molecules and as a reagent in various organic reactions.
Biology: The compound is studied for its potential biological activities and interactions with biomolecules.
Medicine: Research is ongoing to explore its potential therapeutic applications, including its use as a drug intermediate.
Industry: It is utilized in the production of specialty chemicals and as a solvent for nonpolar compounds
Wirkmechanismus
The mechanism of action of 2-t-Butoxynaphthalene involves its interaction with various molecular targets and pathways. The tert-butoxy group can influence the reactivity and orientation of the compound in chemical reactions. In biological systems, it may interact with enzymes and receptors, affecting their function and activity. Detailed studies are required to elucidate the exact molecular mechanisms and pathways involved.
Vergleich Mit ähnlichen Verbindungen
Comparative Analysis with Structural Analogues
Substituent Effects on Physicochemical Properties
The following table summarizes key structural and inferred properties of 2-<i>tert</i>-Butoxynaphthalene and its analogues:
EWG: Electron-Withdrawing Group (via inductive effect of oxygen); *EDG: Electron-Donating Group.
Key Observations:
- Steric Effects : The <i>tert</i>-butoxy group imposes significant steric hindrance, reducing reaction rates at adjacent positions compared to smaller substituents like ethoxy or methyl .
- Electronic Effects: Alkoxy groups (e.g., <i>tert</i>-butoxy, ethoxy) withdraw electrons inductively but donate weakly via resonance. This contrasts with amino groups, which strongly donate electrons, and methylthio groups, which donate via sulfur’s lone pairs .
- Solubility: Bulky substituents like <i>tert</i>-butoxy and phenyl reduce solubility in polar solvents, whereas methyl and ethoxy groups enhance compatibility with nonpolar and moderately polar media, respectively .
Toxicological and Environmental Considerations
The <i>tert</i>-butoxy group’s metabolic fate may differ due to its resistance to enzymatic cleavage compared to smaller alkoxy groups. Environmental persistence is likely higher for bulky derivatives due to reduced biodegradability .
Q & A
Basic Research Questions
Q. What are the established synthesis routes for 2-*-butoxynaphthalene, and what methodological considerations are critical for optimizing yield and purity?
- The synthesis of naphthalene derivatives often involves nucleophilic substitution or alkylation reactions. For example, alkoxy-naphthalenes can be synthesized via the reaction of naphthols with alkyl halides in the presence of a base (e.g., K2CO3) in polar aprotic solvents like dimethylformamide (DMF). Reaction conditions such as temperature (room temperature vs. elevated), solvent choice, and stoichiometry significantly impact yield and purity. Post-synthesis purification via column chromatography or recrystallization is often required .
Q. How can researchers characterize the structural and electronic properties of 2-*-butoxynaphthalene using spectroscopic techniques?
- Key techniques include:
- Nuclear Magnetic Resonance (NMR): 1H and 13C NMR to confirm substitution patterns and purity.
- Mass Spectrometry (MS): High-resolution MS for molecular weight validation.
- Infrared (IR) Spectroscopy: Identification of functional groups (e.g., ether C-O stretching at ~1100 cm−1).
- UV-Vis Spectroscopy: Analysis of conjugation effects due to the naphthalene core.
Q. What are the primary metabolic pathways and degradation mechanisms of 2-*-butoxynaphthalene in biological systems?
- Studies on methylnaphthalenes suggest oxidation via cytochrome P450 enzymes, leading to epoxide intermediates, which may undergo further hydrolysis or conjugation (e.g., glutathione adducts). In vitro assays using liver microsomes or recombinant enzymes are standard for metabolic profiling. Analytical methods like HPLC-MS are essential for tracking metabolites .
Advanced Research Questions
Q. How can conflicting toxicological data on 2-*-butoxynthalene derivatives be resolved through experimental design and bias assessment?
- Contradictions in toxicity studies (e.g., hepatotoxicity vs. no observed effect) may arise from differences in exposure routes (oral vs. inhalation), species-specific metabolism, or dose thresholds. A systematic approach includes:
- Risk of Bias Assessment: Evaluate study design (e.g., blinding, control groups) per tools like SYRCLE for animal studies .
- Dose-Response Analysis: Use benchmark dose modeling to identify thresholds.
- In Silico Predictions: Cross-validate with QSAR models for hepatotoxicity .
Q. What methodological gaps exist in quantifying biomarkers of exposure to 2-*-butoxynaphthalene, and how can they be addressed?
- Current limitations include:
- Sample Preparation: Improved extraction techniques (e.g., solid-phase microextraction) for low-concentration metabolites in biological matrices.
- Standardization: Lack of validated protocols for urinary metabolites like 2-naphthol.
- Correlative Biomarkers: Developing assays linking metabolite levels (e.g., 1,2-dihydroxynaphthalene) to oxidative stress markers (e.g., 8-OHdG) .
Q. How does the steric hindrance of the -butoxy group influence the reactivity of 2--butoxynaphthalene in electrophilic substitution reactions?
- The bulky *-butoxy group directs electrophiles (e.g., nitration, sulfonation) to the less hindered positions (e.g., 6-position on naphthalene). Computational studies (DFT calculations) can predict regioselectivity, while experimental validation requires kinetic monitoring (e.g., time-resolved NMR) .
Q. Data Analysis and Integration
Q. What strategies are recommended for integrating heterogeneous data (e.g., in vitro toxicity, environmental fate) into a cohesive risk assessment framework?
- A tiered approach:
- Tier 1: Compile data from high-throughput screens (e.g., ToxCast) for hazard identification.
- Tier 2: Apply physiologically based pharmacokinetic (PBPK) modeling to extrapolate in vitro results to in vivo scenarios.
- Tier 3: Use probabilistic models to account for population variability and exposure scenarios .
Q. How can researchers validate computational predictions of 2-*-butoxynaphthalene’s environmental persistence using experimental data?
- Compare predicted half-lives (e.g., EPI Suite outputs) with experimental degradation studies under controlled conditions (e.g., aqueous photolysis, soil microbial assays). Discrepancies may indicate unaccounted pathways (e.g., anaerobic degradation) .
Q. Tables
Table 1. Key Analytical Methods for 2-*-Butoxynaphthalene and Metabolites
Analyte | Matrix | Method | Detection Limit | Reference |
---|---|---|---|---|
2-*-Butoxynaphthalene | Urine | GC-MS (SIM mode) | 0.1 µg/L | |
1,2-Dihydroxynaphthalene | Liver microsomes | HPLC-UV | 5 nM | |
Naphthoquinone | Air | LC-TOF-MS | 0.01 ng/m³ |
Table 2. Common Synthetic Routes and Yields
Route | Conditions | Yield (%) | Purity (%) | Reference |
---|---|---|---|---|
Alkylation of 2-naphthol | DMF, K2CO3, 25°C | 78 | >95 | |
Grignard Reaction | THF, -78°C to RT | 65 | 90 |
Eigenschaften
Molekularformel |
C14H16O |
---|---|
Molekulargewicht |
200.28 g/mol |
IUPAC-Name |
2-[(2-methylpropan-2-yl)oxy]naphthalene |
InChI |
InChI=1S/C14H16O/c1-14(2,3)15-13-9-8-11-6-4-5-7-12(11)10-13/h4-10H,1-3H3 |
InChI-Schlüssel |
ZDUKTTJUKLNCSH-UHFFFAOYSA-N |
Kanonische SMILES |
CC(C)(C)OC1=CC2=CC=CC=C2C=C1 |
Herkunft des Produkts |
United States |
Haftungsausschluss und Informationen zu In-Vitro-Forschungsprodukten
Bitte beachten Sie, dass alle Artikel und Produktinformationen, die auf BenchChem präsentiert werden, ausschließlich zu Informationszwecken bestimmt sind. Die auf BenchChem zum Kauf angebotenen Produkte sind speziell für In-vitro-Studien konzipiert, die außerhalb lebender Organismen durchgeführt werden. In-vitro-Studien, abgeleitet von dem lateinischen Begriff "in Glas", beinhalten Experimente, die in kontrollierten Laborumgebungen unter Verwendung von Zellen oder Geweben durchgeführt werden. Es ist wichtig zu beachten, dass diese Produkte nicht als Arzneimittel oder Medikamente eingestuft sind und keine Zulassung der FDA für die Vorbeugung, Behandlung oder Heilung von medizinischen Zuständen, Beschwerden oder Krankheiten erhalten haben. Wir müssen betonen, dass jede Form der körperlichen Einführung dieser Produkte in Menschen oder Tiere gesetzlich strikt untersagt ist. Es ist unerlässlich, sich an diese Richtlinien zu halten, um die Einhaltung rechtlicher und ethischer Standards in Forschung und Experiment zu gewährleisten.