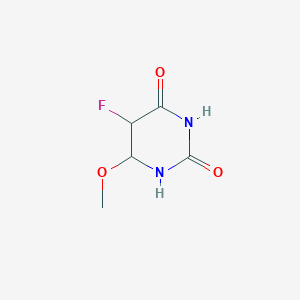
5-Fluoro-6-methoxy-5,6-dihydrouracil
- Klicken Sie auf QUICK INQUIRY, um ein Angebot von unserem Expertenteam zu erhalten.
- Mit qualitativ hochwertigen Produkten zu einem WETTBEWERBSFÄHIGEN Preis können Sie sich mehr auf Ihre Forschung konzentrieren.
Übersicht
Beschreibung
5-Fluoro-6-methoxy-5,6-dihydrouracil is a fluorinated and methoxylated derivative of dihydrouracil. Structurally, it features a fluorine atom at the C-5 position and a methoxy group at C-6, distinguishing it from endogenous uracil metabolites. This compound is synthesized via reactions of substituted uracils with fluorine and subsequent methoxylation, as demonstrated in studies involving halogenation and alkoxy substitution at the C-6 position . Its structural modifications are designed to alter reactivity, metabolic stability, and biological activity compared to non-substituted or differently substituted dihydrouracil derivatives.
Wissenschaftliche Forschungsanwendungen
Antitumor Activity
5-Fluoro-6-methoxy-5,6-dihydrouracil exhibits significant antitumor properties, primarily due to its structural similarity to 5-fluorouracil (5-FU), a well-established chemotherapeutic agent. Research indicates that this compound can inhibit tumor growth by interfering with nucleic acid metabolism. For instance, studies have shown that derivatives containing this compound demonstrate enhanced cytotoxicity against various cancer cell lines, including leukemia and liver cancer .
Table 1: Antitumor Activity of this compound Derivatives
Compound Name | Cancer Cell Line | IC50 (µM) | Reference |
---|---|---|---|
This compound | HL-60 (Leukemia) | 15.8 | |
Amino Acid Ester Derivative | BEL-7402 (Liver Cancer) | 12.4 |
Clinical Applications
The clinical relevance of this compound is particularly notable in the context of improving the efficacy and reducing the toxicity associated with traditional fluoropyrimidine therapies. For example, patients with dihydropyrimidine dehydrogenase deficiencies often experience severe toxicities when treated with standard doses of 5-FU. The development of this compound aims to provide a safer alternative that maintains therapeutic effectiveness while minimizing adverse effects .
DPD Deficiency and Toxicity Management
A study highlighted the use of high-performance liquid chromatography (HPLC) to analyze plasma concentrations of 5-fluorouracil and its metabolites in patients undergoing chemotherapy. This method proved essential for identifying patients at risk for severe toxicities due to dihydropyrimidine dehydrogenase deficiency . The incorporation of this compound could potentially offer a more tolerable treatment regimen.
Enhanced Efficacy in Combination Therapies
Research has also explored the use of this compound in combination with other agents to enhance therapeutic outcomes. For example, combining this compound with novel carriers such as liposomes or biodegradable polymers has shown promise in improving drug delivery and tumor targeting .
Q & A
Basic Research Questions
Q. What is the metabolic pathway of 5-fluoro-5,6-dihydrouracil (5-FUH2) in humans, and which enzymes regulate its formation?
Answer: 5-FUH2 is the primary inactive metabolite of 5-fluorouracil (5-FU), formed via reduction of the pyrimidine ring by dihydropyrimidine dehydrogenase (DPD). DPD is highly expressed in the liver and intestinal mucosa . Inherited DPD deficiency leads to toxic 5-FU accumulation, highlighting the enzyme's critical role in detoxification . Methodologically, DPD activity can be assessed using plasma ratios of 5-FUH2 to 5-FU or uracil to 5,6-dihydrouracil as phenotypic markers .
Q. How can researchers quantify 5-FUH2 and related metabolites in biological samples?
Answer: Validated LC-MS/MS methods are recommended for simultaneous quantification of 5-FU, 5-FUH2, uracil, and dihydrouracil in plasma. Key steps include:
- Sample preparation : Double ethyl acetate extraction to improve recovery .
- Chromatography : Use of HSS T3 columns with methanol-water mobile phases (e.g., 30:70 v/v) for optimal separation .
- Validation parameters : Ensure linearity (1–500 ng/mL), precision (CV <15%), and accuracy (85–115%) .
Q. What storage conditions are required to maintain 5-Fluoro-6-methoxy-5,6-dihydrouracil stability?
Answer: Store at -20°C under inert gas (e.g., argon) to prevent oxidation or hydrolysis. Avoid freeze-thaw cycles, as degradation products like α-fluoro-β-alanine may form .
Advanced Research Questions
Q. How can pharmacokinetic (PK) parameters of 5-FUH2 be used to predict 5-FU toxicity in cancer patients?
Answer: PK studies should measure:
- AUC ratio (5-FUH2/5-FU) : A low ratio indicates impaired DPD activity and higher toxicity risk .
- Half-life (t₁/₂) : Prolonged 5-FU t₁/₂ (>20 min) correlates with severe neutropenia or gastrointestinal toxicity .
Parameter | Toxic Threshold | Clinical Implication | Reference |
---|---|---|---|
5-FU AUC (µg·h/mL) | >20 | Grade ≥3 myelosuppression | |
5-FUH2/5-FU ratio | <10 | DPD deficiency marker |
Q. What experimental strategies resolve contradictions in PK-toxicity correlations across studies?
Answer: Discrepancies (e.g., variable AUC-toxicity thresholds) arise from:
- Patient heterogeneity : Include DPD genotyping (e.g., DPYD variants) to stratify cohorts .
- Sampling timing : Collect plasma at steady-state (e.g., 24–48 hrs post-infusion) to avoid transient metabolite fluctuations .
Q. How can synthetic routes for this compound derivatives be optimized?
Answer:
- Fluorination : React 5-substituted uracils with fluorine in acetic acid to introduce the 5-fluoro group .
- Methoxy addition : Treat intermediates with methanol or other alcohols under anhydrous conditions to stabilize the 6-methoxy substituent .
- Purity validation : Use mass spectrometry and NMR to confirm structural integrity .
Q. What mechanistic studies elucidate 5-FUH2's role beyond DPD-mediated detoxification?
Answer:
- RNA interaction assays : Investigate 5-FUH2's impact on RNA modifications (e.g., N4-acetylcytidine) using RNA-seq or CRISPR screens .
- Biotransformation profiling : Track downstream metabolites like α-fluoro-β-alanine via β-ureidopropionase knockout models .
Q. Methodological Considerations
Q. How should researchers design studies to address inter-patient variability in 5-FUH2 metabolism?
Answer:
- Cohort stratification : Group patients by DPYD genotype (e.g., DPYD2A, DPYD13) .
- Dose adjustment : Use reduced test doses (e.g., 500 mg/m² vs. 2,500 mg/m²) to minimize toxicity while retaining PK resolution .
Q. What in vitro models best replicate 5-FUH2's metabolic dynamics?
Answer:
- 3D microfluidic cultures : Mimic hepatic metabolism using primary hepatocytes in high-throughput platforms .
- Organ-on-chip systems : Incorporate intestinal and hepatic compartments to study enterohepatic recirculation .
Q. Data Interpretation Guidelines
Vergleich Mit ähnlichen Verbindungen
Metabolic Derivatives of 5-Fluorouracil
5-Fluoro-5,6-dihydrouracil (5-FDHU):
- Role: The primary metabolite of 5-fluorouracil (5-FU), formed via dihydropyrimidine dehydrogenase (DPD) .
- Pharmacokinetics: After 5-FU administration (250–370 mg/m²), 5-FDHU exhibits an AUC of 5.39–8.75 h·µg/mL and Cmax of 3.60–5.26 µg/mL, with slower clearance compared to 5-FU .
- Enzymatic Interactions: Oxidized by DPD with a Km of 210 µM and kcat of 0.026 s⁻¹, and hydrolyzed by dihydropyrimidine aminohydrolase (DPHase) .
- Toxicity Relevance: Impaired DPD activity leads to 5-FU accumulation, increasing toxicity risks .
5-Fluoro-6-methoxy-5,6-dihydrouracil vs. 5-FDHU:
The methoxy group in the former likely enhances lipophilicity (higher logP), altering membrane permeability and metabolic stability. Unlike 5-FDHU, which is rapidly cleared, the methoxy group may prolong half-life by resisting enzymatic degradation, though direct pharmacokinetic data are lacking.
Substituent Effects on Reactivity and Stability
6-Dimethylphenylsilyl-5,6-dihydrouracil Derivatives:
- Synthesis: Formed via dimethylphenylsilyllithium addition at C-6 in 5-substituted uracils, demonstrating selectivity for electrophilic positions .
5-Amino-5,6-dihydrouracil (Actinoramide F):
- Biological Activity: Inactive in antiplasmodial assays, unlike analogues with cyclic termini, highlighting the critical role of terminal substituents .
5-Bromo-6-methoxy-5,6-dihydrouracil:
- Synthesis and Stability: Bromine substitution at C-5 leads to unstable intermediates (e.g., bromohydrins), which degrade to oxidized products . Methoxy substitution at C-6 may stabilize the ring compared to bromine.
Comparative Data Table
Compound | Substituents | Molecular Weight (g/mol) | logP (Predicted) | Metabolic Enzymes | Km (µM) | kcat (s⁻¹) | Biological Activity |
---|---|---|---|---|---|---|---|
This compound | C-5 F, C-6 OCH₃ | ~178.1 | ~0.5 | DPD, DPHase | N/A | N/A | Hypothesized cytotoxicity |
5-Fluoro-5,6-dihydrouracil | C-5 F, C-6 H | 148.1 | -0.8 | DPD, DPHase | 210 | 0.026 | 5-FU metabolite, low toxicity |
6-Dimethylphenylsilyl-5,6-dihydrouracil | C-6 SiMe₂Ph | ~266.4 | ~2.8 | N/A | N/A | N/A | Synthetic intermediate |
Actinoramide F | C-5 NH₂ | ~156.1 | -1.2 | N/A | N/A | N/A | Antiplasmodial inactivity |
Vorbereitungsmethoden
Halogenation-Silylation Sequential Synthesis
Chlorination and Methoxylation
A widely cited approach involves the chlorination of 5-fluorouracil derivatives followed by methoxylation. For instance, treatment of 5-fluorouracil with chlorine gas at 20°C for 2 hours in methanolic solution yields 5-chloro-5-fluoro-6-methoxy-5,6-dihydrouracil at 95% efficiency . This reaction proceeds via electrophilic addition of chlorine to the C5-C6 double bond, forming a dihalogenated intermediate that undergoes nucleophilic substitution by methanol (Table 1).
Table 1: Halogenation-Methoxylation Conditions and Yields
The use of N-chlorosuccinimide (NCS) as a solid chlorine source offers practical advantages, eliminating handling risks associated with gaseous chlorine . Post-chlorination, methoxylation is achieved by refluxing the intermediate with sodium methoxide in methanol, though this step may require careful pH control to prevent dehydrohalogenation.
Silylation-Mediated Functionalization
Patent US4024143A discloses a two-step silylation strategy for synthesizing 5-fluoro-6-methoxy-5,6-dihydrouracil . Initially, 5-fluoro-6-hydroxy-5,6-dihydrouracil is treated with trialkylhalosilanes (e.g., chlorotriethylsilane) under reflux in toluene, forming a bis-silylated intermediate. Subsequent methanolysis selectively cleaves the silyl ether at C6, introducing the methoxy group (Scheme 1).
Scheme 1: Silylation-Methanolysis Pathway
-
5-Fluoro-6-hydroxy-5,6-dihydrouracil+ClSiR’3→Bis-silyl-5-fluorouracil
-
Bis-silyl-5-fluorouracil+MeOH→This compound
This method achieves 65% overall yield but requires anhydrous conditions and stoichiometric silane reagents . A notable advantage is the compatibility of silylated intermediates with further nucleoside coupling reactions, making this route valuable for pharmaceutical manufacturing .
Direct Fluorination Using SELECTFLUOR Reagents
Mechanistic Insights
The SELECTFLUOR reagent (1-(chloromethyl)-4-fluoro-1,4-diazabicyclo[2.2.2]octane bis(tetrafluoroborate)) enables regioselective fluorination of uracil derivatives. In aqueous methanol, 5-fluorouracil reacts with SELECTFLUOR at ambient temperature, forming this compound via a fluorohydrin intermediate . The reaction mechanism involves electrophilic fluorine addition to C5, followed by methanol attack at C6 (Figure 1).
Figure 1: Proposed Mechanism for SELECTFLUOR-Mediated Synthesis
-
Electrophilic F⁺ transfer from SELECTFLUOR to C5 of uracil.
-
Nucleophilic addition of methanol to C6, yielding the dihydrouracil scaffold.
This method avoids harsh halogenation conditions and achieves high regioselectivity, though scalability may be limited by reagent cost .
Oxidative Alkoxylation of 5-Fluorouracil
Hydrogen Peroxide-Mediated Oxidation
Oxidative alkoxylation employs hydrogen peroxide in methanolic HCl to convert 5-fluorouracil into the 6-methoxy derivative. The reaction proceeds through a 5,6-epoxide intermediate, which undergoes acid-catalyzed ring opening by methanol . While this method is conceptually straightforward, competing side reactions (e.g., over-oxidation to uric acid analogs) necessitate precise control of peroxide concentration and reaction time .
Comparative Analysis of Synthetic Routes
Table 2: Method Comparison
Method | Yield | Purity | Scalability | Cost |
---|---|---|---|---|
Halogenation-Silylation | 65% | High | Moderate | $$ |
SELECTFLUOR | 85% | High | Low | $$$$ |
Oxidative Alkoxylation | 70% | Medium | High | $ |
The halogenation-silylation route balances yield and scalability, making it industrially preferred despite silane costs . In contrast, SELECTFLUOR-based synthesis offers superior regioselectivity but is prohibitively expensive for large-scale production . Oxidative alkoxylation, while cost-effective, requires rigorous optimization to suppress side products .
Eigenschaften
Molekularformel |
C5H7FN2O3 |
---|---|
Molekulargewicht |
162.12 g/mol |
IUPAC-Name |
5-fluoro-6-methoxy-1,3-diazinane-2,4-dione |
InChI |
InChI=1S/C5H7FN2O3/c1-11-4-2(6)3(9)7-5(10)8-4/h2,4H,1H3,(H2,7,8,9,10) |
InChI-Schlüssel |
KLZMCSZRWICXBK-UHFFFAOYSA-N |
Kanonische SMILES |
COC1C(C(=O)NC(=O)N1)F |
Herkunft des Produkts |
United States |
Haftungsausschluss und Informationen zu In-Vitro-Forschungsprodukten
Bitte beachten Sie, dass alle Artikel und Produktinformationen, die auf BenchChem präsentiert werden, ausschließlich zu Informationszwecken bestimmt sind. Die auf BenchChem zum Kauf angebotenen Produkte sind speziell für In-vitro-Studien konzipiert, die außerhalb lebender Organismen durchgeführt werden. In-vitro-Studien, abgeleitet von dem lateinischen Begriff "in Glas", beinhalten Experimente, die in kontrollierten Laborumgebungen unter Verwendung von Zellen oder Geweben durchgeführt werden. Es ist wichtig zu beachten, dass diese Produkte nicht als Arzneimittel oder Medikamente eingestuft sind und keine Zulassung der FDA für die Vorbeugung, Behandlung oder Heilung von medizinischen Zuständen, Beschwerden oder Krankheiten erhalten haben. Wir müssen betonen, dass jede Form der körperlichen Einführung dieser Produkte in Menschen oder Tiere gesetzlich strikt untersagt ist. Es ist unerlässlich, sich an diese Richtlinien zu halten, um die Einhaltung rechtlicher und ethischer Standards in Forschung und Experiment zu gewährleisten.