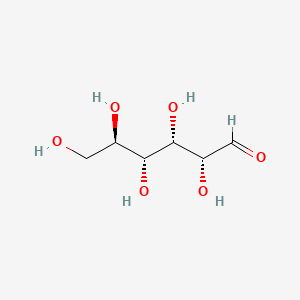
D(+)-Glucose
Übersicht
Beschreibung
D(+)-Glucose, also known as D-glucose, is a simple sugar and an essential carbohydrate in biology. It is a monosaccharide with six carbon atoms and an aldehyde group, making it an aldohexose. This compound is a primary source of energy for living organisms and plays a crucial role in various metabolic processes.
Vorbereitungsmethoden
Synthetic Routes and Reaction Conditions
The synthesis of D(+)-Glucose can be achieved through several methods. One common approach is the hydrolysis of starch or cellulose, which involves breaking down these polysaccharides into simpler sugars using acid or enzymatic hydrolysis. The reaction conditions typically include the use of dilute hydrochloric acid or specific enzymes like amylase at elevated temperatures.
Industrial Production Methods
Industrially, this compound is produced through the enzymatic hydrolysis of starch. This process involves the use of enzymes such as alpha-amylase and glucoamylase to convert starch into glucose. The reaction is carried out in large bioreactors under controlled conditions of temperature and pH to optimize the yield and purity of the product.
Analyse Chemischer Reaktionen
Types of Reactions
D(+)-Glucose undergoes various chemical reactions, including oxidation, reduction, and substitution.
Oxidation: This compound can be oxidized to form gluconic acid using mild oxidizing agents like bromine water.
Reduction: Reduction of this compound with sodium borohydride yields sorbitol, a sugar alcohol.
Substitution: It can undergo substitution reactions to form derivatives like glucosides when reacted with alcohols in the presence of an acid catalyst.
Common Reagents and Conditions
Oxidation: Bromine water, mild conditions.
Reduction: Sodium borohydride, aqueous or alcoholic medium.
Substitution: Alcohols, acid catalysts like hydrochloric acid.
Major Products
Oxidation: Gluconic acid.
Reduction: Sorbitol.
Substitution: Glucosides.
Wissenschaftliche Forschungsanwendungen
D(+)-Glucose has numerous applications in scientific research:
Chemistry: It is used as a standard for calibration in various analytical techniques like high-performance liquid chromatography (HPLC).
Biology: It serves as a primary energy source in cellular respiration and is involved in glycolysis and the citric acid cycle.
Medicine: It is used in the treatment of hypoglycemia and as a component in oral rehydration solutions.
Industry: It is a key ingredient in the food and beverage industry, particularly in the production of sweeteners and fermentation processes.
Wirkmechanismus
The primary mechanism of action of D(+)-Glucose involves its role in cellular metabolism. It is transported into cells via glucose transporters and undergoes glycolysis to produce pyruvate, which enters the citric acid cycle to generate adenosine triphosphate (ATP). This process provides energy for various cellular functions. The compound also participates in the pentose phosphate pathway, which generates reducing equivalents in the form of nicotinamide adenine dinucleotide phosphate (NADPH) and ribose-5-phosphate for nucleotide synthesis.
Vergleich Mit ähnlichen Verbindungen
D(+)-Glucose can be compared with other similar compounds such as:
Fructose: Unlike glucose, fructose is a ketohexose with a ketone group instead of an aldehyde group.
Galactose: Galactose is an epimer of glucose, differing in the configuration around the fourth carbon atom.
Mannose: Mannose is another epimer of glucose, differing in the configuration around the second carbon atom.
Uniqueness
The uniqueness of this compound lies in its central role in metabolism and its widespread presence in nature. It is the most abundant monosaccharide and a fundamental building block for more complex carbohydrates like starch, cellulose, and glycogen.
Eigenschaften
IUPAC Name |
(2R,3S,4R,5R)-2,3,4,5,6-pentahydroxyhexanal | |
---|---|---|
Source | PubChem | |
URL | https://pubchem.ncbi.nlm.nih.gov | |
Description | Data deposited in or computed by PubChem | |
InChI |
InChI=1S/C6H12O6/c7-1-3(9)5(11)6(12)4(10)2-8/h1,3-6,8-12H,2H2/t3-,4+,5+,6+/m0/s1 | |
Source | PubChem | |
URL | https://pubchem.ncbi.nlm.nih.gov | |
Description | Data deposited in or computed by PubChem | |
InChI Key |
GZCGUPFRVQAUEE-SLPGGIOYSA-N | |
Source | PubChem | |
URL | https://pubchem.ncbi.nlm.nih.gov | |
Description | Data deposited in or computed by PubChem | |
Canonical SMILES |
C(C(C(C(C(C=O)O)O)O)O)O | |
Source | PubChem | |
URL | https://pubchem.ncbi.nlm.nih.gov | |
Description | Data deposited in or computed by PubChem | |
Isomeric SMILES |
C([C@H]([C@H]([C@@H]([C@H](C=O)O)O)O)O)O | |
Source | PubChem | |
URL | https://pubchem.ncbi.nlm.nih.gov | |
Description | Data deposited in or computed by PubChem | |
Molecular Formula |
C6H12O6 | |
Source | PubChem | |
URL | https://pubchem.ncbi.nlm.nih.gov | |
Description | Data deposited in or computed by PubChem | |
Related CAS |
25191-16-6 | |
Record name | Polyglucose | |
Source | CAS Common Chemistry | |
URL | https://commonchemistry.cas.org/detail?cas_rn=25191-16-6 | |
Description | CAS Common Chemistry is an open community resource for accessing chemical information. Nearly 500,000 chemical substances from CAS REGISTRY cover areas of community interest, including common and frequently regulated chemicals, and those relevant to high school and undergraduate chemistry classes. This chemical information, curated by our expert scientists, is provided in alignment with our mission as a division of the American Chemical Society. | |
Explanation | The data from CAS Common Chemistry is provided under a CC-BY-NC 4.0 license, unless otherwise stated. | |
DSSTOX Substance ID |
DTXSID7022910, DTXSID4048729 | |
Record name | D-Glucose | |
Source | EPA DSSTox | |
URL | https://comptox.epa.gov/dashboard/DTXSID7022910 | |
Description | DSSTox provides a high quality public chemistry resource for supporting improved predictive toxicology. | |
Record name | DL-Glucose | |
Source | EPA DSSTox | |
URL | https://comptox.epa.gov/dashboard/DTXSID4048729 | |
Description | DSSTox provides a high quality public chemistry resource for supporting improved predictive toxicology. | |
Molecular Weight |
180.16 g/mol | |
Source | PubChem | |
URL | https://pubchem.ncbi.nlm.nih.gov | |
Description | Data deposited in or computed by PubChem | |
Solubility |
Soluble | |
Record name | D-glucose | |
Source | DrugBank | |
URL | https://www.drugbank.ca/drugs/DB01914 | |
Description | The DrugBank database is a unique bioinformatics and cheminformatics resource that combines detailed drug (i.e. chemical, pharmacological and pharmaceutical) data with comprehensive drug target (i.e. sequence, structure, and pathway) information. | |
Explanation | Creative Common's Attribution-NonCommercial 4.0 International License (http://creativecommons.org/licenses/by-nc/4.0/legalcode) | |
Mechanism of Action |
Glucose supplies most of the energy to all tissues by generating energy molecules ATP and NADH during a series of metabolism reactions called glycolysis. Glycolysis can be divided into two main phases where the preparatory phase is initiated by the phosphorylation of glucose by hexokinase to form glucose 6-phosphate. The addition of the high-energy phosphate group activates glucose for the subsequent breakdown in later steps of glycolysis and is the rate-limiting step. Products end up as substrates for following reactions, to ultimately convert C6 glucose molecule into two C3 sugar molecules. These products enter the energy-releasing phase where the total of 4ATP and 2NADH molecules are generated per one glucose molecule. The total aerobic metabolism of glucose can produce up to 36 ATP molecules. These energy-producing reactions of glucose are limited to D-glucose as L-glucose cannot be phosphorylated by hexokinase. Glucose can act as precursors to generate other biomolecules such as vitamin C. It plays a role as a signaling molecule to control glucose and energy homeostasis. Glucose can regulate gene transcription, enzyme activity, hormone secretion, and the activity of glucoregulatory neurons. The types, number, and kinetics of glucose transporters expressed depends on the tissues and fine-tunes glucose uptake, metabolism, and signal generation to preserve cellular and whole body metabolic integrity. | |
Record name | D-glucose | |
Source | DrugBank | |
URL | https://www.drugbank.ca/drugs/DB01914 | |
Description | The DrugBank database is a unique bioinformatics and cheminformatics resource that combines detailed drug (i.e. chemical, pharmacological and pharmaceutical) data with comprehensive drug target (i.e. sequence, structure, and pathway) information. | |
Explanation | Creative Common's Attribution-NonCommercial 4.0 International License (http://creativecommons.org/licenses/by-nc/4.0/legalcode) | |
CAS No. |
50-99-7, 815-92-9, 19030-38-7, 28823-03-2, 58367-01-4 | |
Record name | Glucose | |
Source | CAS Common Chemistry | |
URL | https://commonchemistry.cas.org/detail?cas_rn=50-99-7 | |
Description | CAS Common Chemistry is an open community resource for accessing chemical information. Nearly 500,000 chemical substances from CAS REGISTRY cover areas of community interest, including common and frequently regulated chemicals, and those relevant to high school and undergraduate chemistry classes. This chemical information, curated by our expert scientists, is provided in alignment with our mission as a division of the American Chemical Society. | |
Explanation | The data from CAS Common Chemistry is provided under a CC-BY-NC 4.0 license, unless otherwise stated. | |
Record name | Glucose [JAN] | |
Source | ChemIDplus | |
URL | https://pubchem.ncbi.nlm.nih.gov/substance/?source=chemidplus&sourceid=0000050997 | |
Description | ChemIDplus is a free, web search system that provides access to the structure and nomenclature authority files used for the identification of chemical substances cited in National Library of Medicine (NLM) databases, including the TOXNET system. | |
Record name | D-Glucose, labeled with carbon-14 | |
Source | ChemIDplus | |
URL | https://pubchem.ncbi.nlm.nih.gov/substance/?source=chemidplus&sourceid=0000815929 | |
Description | ChemIDplus is a free, web search system that provides access to the structure and nomenclature authority files used for the identification of chemical substances cited in National Library of Medicine (NLM) databases, including the TOXNET system. | |
Record name | D-Glucose, labeled with carbon-13 | |
Source | ChemIDplus | |
URL | https://pubchem.ncbi.nlm.nih.gov/substance/?source=chemidplus&sourceid=0019030387 | |
Description | ChemIDplus is a free, web search system that provides access to the structure and nomenclature authority files used for the identification of chemical substances cited in National Library of Medicine (NLM) databases, including the TOXNET system. | |
Record name | D-Glucose, labeled with tritium | |
Source | ChemIDplus | |
URL | https://pubchem.ncbi.nlm.nih.gov/substance/?source=chemidplus&sourceid=0028823032 | |
Description | ChemIDplus is a free, web search system that provides access to the structure and nomenclature authority files used for the identification of chemical substances cited in National Library of Medicine (NLM) databases, including the TOXNET system. | |
Record name | D-glucose | |
Source | DrugBank | |
URL | https://www.drugbank.ca/drugs/DB01914 | |
Description | The DrugBank database is a unique bioinformatics and cheminformatics resource that combines detailed drug (i.e. chemical, pharmacological and pharmaceutical) data with comprehensive drug target (i.e. sequence, structure, and pathway) information. | |
Explanation | Creative Common's Attribution-NonCommercial 4.0 International License (http://creativecommons.org/licenses/by-nc/4.0/legalcode) | |
Record name | D-Glucose | |
Source | EPA DSSTox | |
URL | https://comptox.epa.gov/dashboard/DTXSID7022910 | |
Description | DSSTox provides a high quality public chemistry resource for supporting improved predictive toxicology. | |
Record name | DL-Glucose | |
Source | EPA DSSTox | |
URL | https://comptox.epa.gov/dashboard/DTXSID4048729 | |
Description | DSSTox provides a high quality public chemistry resource for supporting improved predictive toxicology. | |
Record name | ANHYDROUS DEXTROSE | |
Source | FDA Global Substance Registration System (GSRS) | |
URL | https://gsrs.ncats.nih.gov/ginas/app/beta/substances/5SL0G7R0OK | |
Description | The FDA Global Substance Registration System (GSRS) enables the efficient and accurate exchange of information on what substances are in regulated products. Instead of relying on names, which vary across regulatory domains, countries, and regions, the GSRS knowledge base makes it possible for substances to be defined by standardized, scientific descriptions. | |
Explanation | Unless otherwise noted, the contents of the FDA website (www.fda.gov), both text and graphics, are not copyrighted. They are in the public domain and may be republished, reprinted and otherwise used freely by anyone without the need to obtain permission from FDA. Credit to the U.S. Food and Drug Administration as the source is appreciated but not required. | |
Retrosynthesis Analysis
AI-Powered Synthesis Planning: Our tool employs the Template_relevance Pistachio, Template_relevance Bkms_metabolic, Template_relevance Pistachio_ringbreaker, Template_relevance Reaxys, Template_relevance Reaxys_biocatalysis model, leveraging a vast database of chemical reactions to predict feasible synthetic routes.
One-Step Synthesis Focus: Specifically designed for one-step synthesis, it provides concise and direct routes for your target compounds, streamlining the synthesis process.
Accurate Predictions: Utilizing the extensive PISTACHIO, BKMS_METABOLIC, PISTACHIO_RINGBREAKER, REAXYS, REAXYS_BIOCATALYSIS database, our tool offers high-accuracy predictions, reflecting the latest in chemical research and data.
Strategy Settings
Precursor scoring | Relevance Heuristic |
---|---|
Min. plausibility | 0.01 |
Model | Template_relevance |
Template Set | Pistachio/Bkms_metabolic/Pistachio_ringbreaker/Reaxys/Reaxys_biocatalysis |
Top-N result to add to graph | 6 |
Feasible Synthetic Routes
Haftungsausschluss und Informationen zu In-Vitro-Forschungsprodukten
Bitte beachten Sie, dass alle Artikel und Produktinformationen, die auf BenchChem präsentiert werden, ausschließlich zu Informationszwecken bestimmt sind. Die auf BenchChem zum Kauf angebotenen Produkte sind speziell für In-vitro-Studien konzipiert, die außerhalb lebender Organismen durchgeführt werden. In-vitro-Studien, abgeleitet von dem lateinischen Begriff "in Glas", beinhalten Experimente, die in kontrollierten Laborumgebungen unter Verwendung von Zellen oder Geweben durchgeführt werden. Es ist wichtig zu beachten, dass diese Produkte nicht als Arzneimittel oder Medikamente eingestuft sind und keine Zulassung der FDA für die Vorbeugung, Behandlung oder Heilung von medizinischen Zuständen, Beschwerden oder Krankheiten erhalten haben. Wir müssen betonen, dass jede Form der körperlichen Einführung dieser Produkte in Menschen oder Tiere gesetzlich strikt untersagt ist. Es ist unerlässlich, sich an diese Richtlinien zu halten, um die Einhaltung rechtlicher und ethischer Standards in Forschung und Experiment zu gewährleisten.