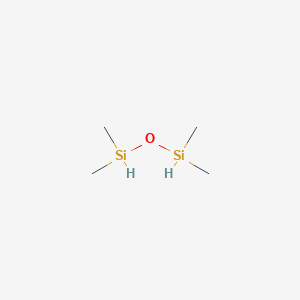
1,1,3,3-Tetramethyl-disiloxane
Übersicht
Beschreibung
1,1,3,3-Tetramethyl-disiloxane is an organosilicon compound with the molecular formula C4H14OSi2. It is a colorless, transparent liquid known for its mild reducing properties and its use as a hydride source in various chemical reactions. This compound is widely used in organic synthesis, polymer production, and as an intermediate in the preparation of other organosilicon compounds .
Vorbereitungsmethoden
1,1,3,3-Tetramethyl-disiloxane can be synthesized through several methods:
Reduction of 1,1,3,3-tetramethyl-1,3-dichloro-1,3-disiloxane: This method involves the reduction of 1,1,3,3-tetramethyl-1,3-dichloro-1,3-disiloxane using metal hydrides such as lithium aluminum hydride, sodium aluminum hydride, or lithium borohydride in tetrahydrofuran.
Hydrolysis of Dimethylchlorosilane: This conventional method uses the hydrolysis of dimethylchlorosilane to produce this compound.
Analyse Chemischer Reaktionen
1,1,3,3-Tetramethyl-disiloxane undergoes various chemical reactions, including:
Hydrosilylation: It acts as a hydride source in hydrosilylation reactions to synthesize mono-functionalized siloxane derivatives.
Reduction: It is used as a reducing agent to synthesize alkyl halides from aldehydes.
Dehydrogenative Silylation: This reaction involves the formation of polymers by reacting with alkadienes and alkynes.
Bromination: It enables direct bromination of carboxylic acids in the presence of indium bromide as a catalyst.
Common reagents and conditions used in these reactions include platinum catalysts, indium bromide, and various metal hydrides. The major products formed from these reactions are siloxane derivatives, alkyl halides, and polymers .
Wissenschaftliche Forschungsanwendungen
Wirkmechanismus
The mechanism by which 1,1,3,3-tetramethyl-disiloxane exerts its effects involves its electrophilic nature, making it an attractive hydride source. In hydrosilylation reactions, it donates hydride ions to unsaturated substrates, facilitating the formation of siloxane bonds. In reduction reactions, it transfers hydride ions to carbonyl compounds, reducing them to alcohols or amines .
Vergleich Mit ähnlichen Verbindungen
1,1,3,3-Tetramethyl-disiloxane can be compared with other similar organosilicon compounds:
1,1,3,3,5,5-Hexamethyltrisiloxane: This compound has a similar structure but with an additional siloxane unit, making it more suitable for certain polymer applications.
Octamethylcyclotetrasiloxane: This cyclic compound is used in the production of silicone elastomers and resins.
1,1,1,3,3,3-Hexafluoro-2-propanol: Although not a siloxane, this compound is used in similar reduction reactions due to its strong hydride-donating properties.
This compound stands out due to its mild reducing properties, making it a versatile reagent in organic synthesis and polymer production .
Eigenschaften
IUPAC Name |
dimethylsilyloxy(dimethyl)silane | |
---|---|---|
Details | Computed by LexiChem 2.6.6 (PubChem release 2019.06.18) | |
Source | PubChem | |
URL | https://pubchem.ncbi.nlm.nih.gov | |
Description | Data deposited in or computed by PubChem | |
InChI |
InChI=1S/C4H14OSi2/c1-6(2)5-7(3)4/h6-7H,1-4H3 | |
Details | Computed by InChI 1.0.5 (PubChem release 2019.06.18) | |
Source | PubChem | |
URL | https://pubchem.ncbi.nlm.nih.gov | |
Description | Data deposited in or computed by PubChem | |
InChI Key |
NVYQDQZEMGUESH-UHFFFAOYSA-N | |
Details | Computed by InChI 1.0.5 (PubChem release 2019.06.18) | |
Source | PubChem | |
URL | https://pubchem.ncbi.nlm.nih.gov | |
Description | Data deposited in or computed by PubChem | |
Canonical SMILES |
C[SiH](C)O[SiH](C)C | |
Details | Computed by OEChem 2.1.5 (PubChem release 2019.06.18) | |
Source | PubChem | |
URL | https://pubchem.ncbi.nlm.nih.gov | |
Description | Data deposited in or computed by PubChem | |
Molecular Formula |
C4H14OSi2 | |
Details | Computed by PubChem 2.1 (PubChem release 2019.06.18) | |
Source | PubChem | |
URL | https://pubchem.ncbi.nlm.nih.gov | |
Description | Data deposited in or computed by PubChem | |
Related CAS |
30812-70-5 | |
Details | Compound: Disiloxane, 1,1,3,3-tetramethyl-, homopolymer | |
Record name | Disiloxane, 1,1,3,3-tetramethyl-, homopolymer | |
Source | CAS Common Chemistry | |
URL | https://commonchemistry.cas.org/detail?cas_rn=30812-70-5 | |
Description | CAS Common Chemistry is an open community resource for accessing chemical information. Nearly 500,000 chemical substances from CAS REGISTRY cover areas of community interest, including common and frequently regulated chemicals, and those relevant to high school and undergraduate chemistry classes. This chemical information, curated by our expert scientists, is provided in alignment with our mission as a division of the American Chemical Society. | |
Explanation | The data from CAS Common Chemistry is provided under a CC-BY-NC 4.0 license, unless otherwise stated. | |
Molecular Weight |
134.32 g/mol | |
Details | Computed by PubChem 2.1 (PubChem release 2021.05.07) | |
Source | PubChem | |
URL | https://pubchem.ncbi.nlm.nih.gov | |
Description | Data deposited in or computed by PubChem | |
CAS No. |
3277-26-7 | |
Record name | 1,1,3,3-Tetramethyldisiloxane | |
Source | CAS Common Chemistry | |
URL | https://commonchemistry.cas.org/detail?cas_rn=3277-26-7 | |
Description | CAS Common Chemistry is an open community resource for accessing chemical information. Nearly 500,000 chemical substances from CAS REGISTRY cover areas of community interest, including common and frequently regulated chemicals, and those relevant to high school and undergraduate chemistry classes. This chemical information, curated by our expert scientists, is provided in alignment with our mission as a division of the American Chemical Society. | |
Explanation | The data from CAS Common Chemistry is provided under a CC-BY-NC 4.0 license, unless otherwise stated. | |
Synthesis routes and methods
Procedure details
Retrosynthesis Analysis
AI-Powered Synthesis Planning: Our tool employs the Template_relevance Pistachio, Template_relevance Bkms_metabolic, Template_relevance Pistachio_ringbreaker, Template_relevance Reaxys, Template_relevance Reaxys_biocatalysis model, leveraging a vast database of chemical reactions to predict feasible synthetic routes.
One-Step Synthesis Focus: Specifically designed for one-step synthesis, it provides concise and direct routes for your target compounds, streamlining the synthesis process.
Accurate Predictions: Utilizing the extensive PISTACHIO, BKMS_METABOLIC, PISTACHIO_RINGBREAKER, REAXYS, REAXYS_BIOCATALYSIS database, our tool offers high-accuracy predictions, reflecting the latest in chemical research and data.
Strategy Settings
Precursor scoring | Relevance Heuristic |
---|---|
Min. plausibility | 0.01 |
Model | Template_relevance |
Template Set | Pistachio/Bkms_metabolic/Pistachio_ringbreaker/Reaxys/Reaxys_biocatalysis |
Top-N result to add to graph | 6 |
Feasible Synthetic Routes
Haftungsausschluss und Informationen zu In-Vitro-Forschungsprodukten
Bitte beachten Sie, dass alle Artikel und Produktinformationen, die auf BenchChem präsentiert werden, ausschließlich zu Informationszwecken bestimmt sind. Die auf BenchChem zum Kauf angebotenen Produkte sind speziell für In-vitro-Studien konzipiert, die außerhalb lebender Organismen durchgeführt werden. In-vitro-Studien, abgeleitet von dem lateinischen Begriff "in Glas", beinhalten Experimente, die in kontrollierten Laborumgebungen unter Verwendung von Zellen oder Geweben durchgeführt werden. Es ist wichtig zu beachten, dass diese Produkte nicht als Arzneimittel oder Medikamente eingestuft sind und keine Zulassung der FDA für die Vorbeugung, Behandlung oder Heilung von medizinischen Zuständen, Beschwerden oder Krankheiten erhalten haben. Wir müssen betonen, dass jede Form der körperlichen Einführung dieser Produkte in Menschen oder Tiere gesetzlich strikt untersagt ist. Es ist unerlässlich, sich an diese Richtlinien zu halten, um die Einhaltung rechtlicher und ethischer Standards in Forschung und Experiment zu gewährleisten.