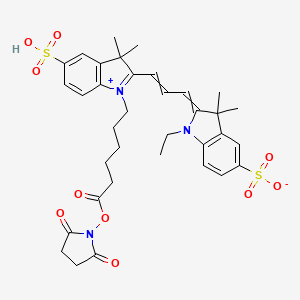
Cyanine 3, SE
Übersicht
Beschreibung
Cyanine 3, succinimidyl ester (Cyanine 3, SE) is a monofunctional NHS ester dye widely used for labeling peptides, proteins, and oligonucleotides. Its structure includes a reactive succinimidyl ester group that forms stable amide bonds with primary amines in biomolecules . The dye exhibits an excitation wavelength of 550 nm and emission at 570 nm, with a high extinction coefficient (~150,000 M⁻¹cm⁻¹) and quantum yield (~0.15), making it ideal for fluorescence microscopy, flow cytometry, and proteomic studies . When conjugated to proteins, this compound demonstrates enhanced fluorescence intensity due to reduced environmental quenching .
Vorbereitungsmethoden
Core Structure Synthesis: Monomethine Cyanine Dye Framework
The foundational step in preparing Cyanine 3, SE involves constructing its monomethine cyanine core, characterized by a conjugated polymethine chain linking two nitrogen-containing heterocycles. Contemporary synthesis routes leverage classical and novel strategies to optimize efficiency and purity.
Thioalkyl Method: Traditional One-Pot Synthesis
The thioalkyl method remains a cornerstone for symmetric monomethine cyanine dye synthesis. This one-pot approach involves quaternizing 2-methylbenzothiazole derivatives with methyl tosylate at 120°C, followed by condensation in pyridine to form the cyanine backbone . For example, dyes 2 –5 (Table 1) were synthesized with yields ranging from 68% to 94% by varying counterions (Cl⁻, Br⁻, I⁻, TsO⁻) . The reaction’s success hinges on the leaving group’s nucleophilicity, with tosylate facilitating higher yields due to its stability under reflux conditions.
Table 1: Thioalkyl Method Performance for Select Monomethine Cyanine Dyes
Dye | R Group | Counterion (W) | Yield (%) | λₐᵦₛ (nm) | Log ε |
---|---|---|---|---|---|
2 | CH₃ | Cl⁻ | 68 | 424 | 4.97 |
3 | CH₃ | Br⁻ | 69 | 424 | 4.83 |
4 | CH₃ | I⁻ | 94 | 424 | 4.96 |
5 | CH₃ | TsO⁻ | 77 | 424 | 4.98 |
This method’s limitation lies in its dependency on alkylthio leaving groups, which can complicate purification due to byproduct formation .
Nitrite Method: Alternative Pathway for Symmetric Dyes
To circumvent the thioalkyl method’s constraints, the nitrite method offers a streamlined route for symmetric monomethine cyanines. By reacting pre-quaternized heterocycles with sodium nitrite in acidic ethanol, dyes 8 –11 and 14 were synthesized in 25–75% yields . Although lower in efficiency, this approach eliminates the need for alkylthio intermediates, simplifying isolation. For instance, dye 8 (λₐᵦₛ = 424 nm) achieved a 75% yield using benzothiazole derivatives .
Functionalization with NHS Ester: Activating for Bioconjugation
The critical distinction of this compound lies in its N-hydroxysuccinimide (NHS) ester group, enabling covalent attachment to primary amines. This modification typically follows core structure synthesis through post-functionalization steps.
Carboxyl Group Activation
While explicit details of NHS esterification are sparse in the provided sources, industry protocols infer that the cyanine core’s terminal carboxyl group undergoes activation using NHS in the presence of carbodiimide crosslinkers (e.g., EDC) . For example, MedChemExpress’s protocol involves dissolving the cyanine dye in anhydrous DMSO and reacting it with a 10-fold molar excess of NHS under inert conditions . The reaction proceeds at room temperature for 2–4 hours, followed by precipitation in cold diethyl ether to isolate the NHS ester .
Purification and Characterization
Post-synthesis purification employs size-exclusion chromatography (e.g., Sephadex G-25) to separate the NHS ester from unreacted dye . Analytical techniques such as UV-Vis spectroscopy (λₐᵦₛ = 550 nm, ε = 150,000 M⁻¹cm⁻¹) and NMR confirm functional group integrity . For this compound, the NHS ester’s incorporation is evident via a characteristic shift in the ¹H NMR spectrum’s methylene protons adjacent to the succinimidyl group .
Optimization Challenges and Solutions
Solubility and Reactivity Trade-offs
This compound’s hydrophobic nature necessitates polar aprotic solvents (e.g., DMSO) for NHS esterification, risking protein denaturation during bioconjugation . To mitigate this, sulfo-Cyanine 3 variants introduce sulfonate groups, enhancing water solubility but requiring additional synthetic steps .
Counterion Selection
The choice of counterion (W) profoundly impacts stability and reactivity. Iodide (I⁻) and tosylate (TsO⁻) counterions in dyes 4 and 5 improve crystallinity and shelf life compared to chloride or bromide . However, iodide’s nucleophilicity may interfere with NHS ester hydrolysis, necessitating stringent anhydrous conditions during storage .
Industrial-Scale Production Protocols
Large-scale synthesis of this compound adheres to Good Manufacturing Practice (GMP) guidelines, emphasizing reproducibility and purity. Lumiprobe’s production workflow highlights:
-
Step 1 : Batch synthesis of monomethine cyanine core via thioalkyl method (≥95% purity) .
-
Step 2 : NHS esterification in reactors under nitrogen atmosphere, monitored by HPLC .
-
Step 3 : Lyophilization to yield stable powder, stored at -20°C with desiccants .
Table 2: Industrial Synthesis Parameters for this compound
Parameter | Value |
---|---|
Reaction Scale | 1–100 kg batches |
Purity Post-Purification | ≥95% (HPLC) |
Storage Conditions | -20°C, desiccated |
Analyse Chemischer Reaktionen
Arten von Reaktionen
CY3-SE unterliegt hauptsächlich Substitutionsreaktionen, bei denen die NHS-Estergruppe mit primären Aminen an Biomolekülen reagiert, um stabile Amidbindungen zu bilden. Diese Reaktion ist hochspezifisch und effizient, was CY3-SE zu einem idealen Reagenz zum Markieren von Proteinen, Peptiden und Nukleinsäuren macht .
Häufige Reagenzien und Bedingungen
Die Markierungsreaktion mit CY3-SE wird typischerweise in einer Pufferlösung bei einem pH-Wert von 7-9 durchgeführt. Häufige verwendete Puffer umfassen Natriumphosphat, HEPES und Carbonat-/Bicarbonatpuffer. Die Reaktion wird üblicherweise bei Raumtemperatur oder leicht erhöhten Temperaturen durchgeführt, um eine vollständige Markierung zu gewährleisten .
Hauptprodukte
Die Hauptprodukte der Reaktion zwischen CY3-SE und Biomolekülen sind fluoreszenzmarkierte Proteine, Peptide oder Nukleinsäuren. Diese markierten Biomoleküle behalten ihre biologische Aktivität und können in verschiedenen analytischen und bildgebenden Anwendungen verwendet werden .
Wissenschaftliche Forschungsanwendungen
Fluorescence Imaging
Cyanine dyes, including Cyanine 3, SE, are integral to fluorescence imaging techniques. They are utilized in single-molecule and super-resolution imaging due to their high quantum yield and photostability. Recent studies have demonstrated the conversion of Cyanine 5 to Cyanine 3 upon photoexcitation, which can be leveraged for advanced imaging techniques without the need for harmful UV light .
Molecular Labeling
The NHS ester form of this compound allows it to react with primary amines on proteins and other biomolecules. This property is crucial for labeling applications in proteomics and cellular studies. The dye's ability to label amines makes it a valuable tool for tracking biomolecular interactions and dynamics within living cells .
Fluorescence Sensors
Cyanine dyes have been explored as potential fluorescence sensors in cellular environments. A comparative study examined several cyanine-based dyes, including this compound, for their ability to act as sensors responding to changes in macromolecular crowding within cells. The results indicated that while other dyes showed promise, the unique properties of Cyanine 3 make it suitable for specific sensing applications .
G-Quadruplex DNA Studies
Cyanine 3 has been employed to investigate structural changes in G-quadruplex DNA, which is significant in cancer research. By labeling these structures with Cyanine 3, researchers can monitor conformational changes that may influence gene expression and cellular behavior .
Case Study: Fluorescence Imaging Techniques
In a study focusing on the photoconversion of Cyanine dyes during imaging, researchers elucidated the mechanism by which Cyanine 5 converts to Cyanine 3 under specific conditions. This finding has implications for multicolor fluorescence imaging, allowing researchers to utilize this conversion strategically to enhance imaging resolution and accuracy without introducing toxic agents .
Case Study: Cellular Sensing Applications
A recent investigation into the use of Cyanine dyes as sensors revealed that while some cyanines performed well under controlled conditions, Cyanine 3 displayed unique stability and photophysical properties that allowed for effective monitoring of cellular environments. The study highlighted the dye's ability to respond dynamically to variations in macromolecular crowding within HeLa cells .
Wirkmechanismus
The mechanism of action of CY3-SE involves the formation of a covalent bond between the NHS ester group of the dye and the primary amine group of the target biomolecule. This reaction forms a stable amide bond, resulting in the fluorescent labeling of the biomolecule. The fluorescence of CY3-SE is due to the excitation of the cyanine dye moiety, which emits light at a specific wavelength when excited by a suitable light source .
Vergleich Mit ähnlichen Verbindungen
Comparison with Similar Cyanine Dyes
Structural and Spectral Properties
Table 1: Spectral and Physicochemical Properties
- Key Observations :
- This compound and IC-OSu ethyl-Cy3 share identical spectral profiles but differ in applications; the latter is optimized for pre-electrophoretic labeling in proteomics .
- Heptamethine dyes like IR-783 exhibit near-infrared (NIR) emission (>800 nm), enabling deeper tissue penetration compared to this compound’s visible-range emission .
- Cationic cyanines (e.g., DiOC2(3)) accumulate in mitochondria due to lipophilicity, whereas this compound relies on covalent conjugation for localization .
Research Limitations and Contradictions
- This contrasts with this compound, which is non-toxic regardless of charge due to covalent conjugation .
- Albumin Dependence : Tumor targeting of this compound and IR-783 is contingent on albumin complexation, limiting utility in hypoalbuminemic conditions .
Biologische Aktivität
Cyanine 3, SE (CAS 146368-16-3) is a fluorescent dye widely used in biological research for labeling amines in biomolecules, particularly proteins, peptides, and oligonucleotides. This article delves into the biological activity of this compound, highlighting its properties, applications, and relevant research findings.
This compound is characterized by its orange fluorescence with an emission maximum around 570 nm. It exhibits the following key optical properties:
Property | Value |
---|---|
Emission Color | Orange |
Absorption Maximum (λ abs) | 550 nm |
Emission Maximum (λ em) | 570 nm |
Extinction Coefficient (ε) | 150,000 M⁻¹cm⁻¹ |
Quantum Yield (φ) | 0.31 |
Reactive Group | NHS ester |
Cell Permeable | No |
These properties make this compound suitable for various applications in fluorescence microscopy and flow cytometry .
Cyanine dyes, including this compound, interact with biomolecules through covalent bonding with primary amines. This reactivity allows for specific labeling of target molecules within complex biological systems. The dye's fluorescence is influenced by its environment within the biomolecule, affecting its quantum yield and stability .
Antiproliferative Effects
Research indicates that cyanine dyes exhibit significant antiproliferative effects on various human cancer cell lines. For instance, studies have shown that certain cyanine derivatives can induce cell cycle arrest in the G0/G1 phase, leading to decreased proliferation rates in treated cells. This effect has been attributed to the dyes' ability to interact with cellular components such as DNA and RNA .
Cellular Localization
Confocal microscopy studies reveal that this compound tends to accumulate preferentially in specific cellular compartments. The dye has been observed to localize within mitochondria and nuclei of cells, suggesting potential applications in studying mitochondrial dynamics and nuclear processes .
Case Studies
- Cancer Cell Line Study : In a study involving various human cancer cell lines, this compound demonstrated submicromolar activity against all tested lines. The dye's localization patterns were analyzed using confocal microscopy, confirming its potential as a theranostic agent due to its dual role in imaging and therapeutic applications .
- DNA Interaction : Another study focused on the interaction of cyanine dyes with DNA demonstrated that the binding affinity and fluorescence properties varied significantly based on the sequence and structural context of the DNA. This variability highlights the importance of considering molecular interactions when utilizing cyanine dyes for labeling purposes .
Q & A
Basic Research Questions
Q. What are the optimal reaction conditions for conjugating Cyanine 3, SE to amine-containing biomolecules?
Methodological Answer: Successful conjugation requires adjusting pH (8.0–8.5 using sodium bicarbonate buffer), a 10:1 molar ratio (dye:protein), and incubation at 4°C for 1–2 hours. Post-reaction, unbound dye should be removed via size-exclusion chromatography or dialysis. Validate efficiency using absorbance spectroscopy (e.g., measuring the 550 nm peak for Cy3) . Ensure biomolecule purity, as contaminants (e.g., BSA) may compete for amine binding .
Q. How should researchers handle and store this compound to maintain its reactivity and fluorescence efficiency?
Methodological Answer: Store lyophilized this compound in a desiccator at -20°C, protected from light. For working solutions, dissolve in anhydrous DMSO to prevent hydrolysis. Avoid repeated freeze-thaw cycles. Fluorescence stability in labeled probes can degrade under prolonged light exposure; include light-protected controls in imaging workflows .
Q. What methods are recommended for quantifying the conjugation efficiency of this compound to proteins?
Methodological Answer: Use absorbance spectroscopy to calculate the dye-to-protein ratio (DPR):
where = 150,000 M⁻¹cm⁻¹ and is protein-specific. SDS-PAGE with fluorescence imaging can confirm covalent binding .
Advanced Research Questions
Q. How can researchers mitigate variability in Cyanine 3 fluorescence signals caused by environmental factors during imaging?
Methodological Answer: Normalize signals using internal controls (e.g., housekeeping protein labels). Account for photobleaching by limiting exposure time and using antifade mounting media. For quantitative studies, include calibration curves with known dye concentrations. Statistical analysis (e.g., coefficient of variation (% CV)) should be applied to assess intra-experiment variability .
Q. What statistical approaches are appropriate for analyzing Cyanine 3-derived data in high-throughput screening assays?
Methodological Answer: Use robust regression models to correct for background noise (e.g., median blank + 3 SD threshold, as in ). For multiplexed assays, apply spectral unmixing algorithms to resolve overlapping emissions. Non-parametric tests (e.g., Kruskal-Wallis) are recommended for non-normal distributions .
Q. How can this compound be integrated into multiplexed assays with other fluorescent dyes without spectral overlap interference?
Methodological Answer: Select dyes with non-overlapping emission spectra (e.g., pair Cy3 with Cy5 or Alexa Fluor 488). Use narrow-bandpass filters and validate via single-dye control experiments. For spectral imaging, linear unmixing software (e.g., Zeiss Zen) can isolate signals. Cross-talk should be quantified and subtracted during analysis .
Q. What steps should be taken to troubleshoot low labeling efficiency of this compound in protein conjugation experiments?
Methodological Answer:
- Confirm protein integrity (SDS-PAGE) and amine accessibility (e.g., lysine residues).
- Test alternative buffers (e.g., PBS vs. carbonate) and adjust pH.
- Increase dye-to-protein ratio (up to 20:1) for low-affinity targets.
- Use centrifugal filtration to concentrate proteins before labeling .
Q. How should researchers assess the stability of Cyanine 3-labeled probes over extended experimental timelines?
Methodological Answer: Perform accelerated degradation studies: incubate probes at 37°C and measure fluorescence intensity weekly. Compare to -80°C-stocked controls. Use size-exclusion HPLC to detect aggregation or hydrolysis. Stability thresholds should align with assay duration (e.g., >90% signal retention over 4 weeks) .
Q. What computational tools or algorithms are recommended for deconvoluting Cyanine 3 signals in complex biological samples?
Methodological Answer: Use ImageJ plugins (e.g., FIJI) for background subtraction and thresholding. For 3D imaging, employ deconvolution software like Huygens or Imaris. Machine learning models (e.g., U-Net) can segment Cy3 signals in dense tissues when trained on ground-truth datasets .
Q. How can researchers validate the specificity of Cyanine 3-labeled probes in live-cell imaging studies?
Methodological Answer: Include negative controls (e.g., unlabeled cells or competition assays with excess unlabeled ligand). For receptor studies, use knockout cell lines or siRNA silencing. Co-localization analysis with organelle-specific markers (e.g., MitoTracker) confirms targeting accuracy .
Eigenschaften
CAS-Nummer |
146368-16-3 |
---|---|
Molekularformel |
C35H41N3O10S2 |
Molekulargewicht |
727.8 g/mol |
IUPAC-Name |
(2Z)-2-[(E)-3-[1-[6-(2,5-dioxopyrrolidin-1-yl)oxy-6-oxohexyl]-3,3-dimethyl-5-sulfoindol-1-ium-2-yl]prop-2-enylidene]-1-ethyl-3,3-dimethylindole-5-sulfonate |
InChI |
InChI=1S/C35H41N3O10S2/c1-6-36-27-16-14-23(49(42,43)44)21-25(27)34(2,3)29(36)11-10-12-30-35(4,5)26-22-24(50(45,46)47)15-17-28(26)37(30)20-9-7-8-13-33(41)48-38-31(39)18-19-32(38)40/h10-12,14-17,21-22H,6-9,13,18-20H2,1-5H3,(H-,42,43,44,45,46,47) |
InChI-Schlüssel |
SOVMUUCAZOFIQP-UHFFFAOYSA-N |
SMILES |
CCN1C2=C(C=C(C=C2)S(=O)(=O)[O-])C(C1=CC=CC3=[N+](C4=C(C3(C)C)C=C(C=C4)S(=O)(=O)O)CCCCCC(=O)ON5C(=O)CCC5=O)(C)C |
Isomerische SMILES |
CCN\1C2=C(C=C(C=C2)S(=O)(=O)[O-])C(/C1=C/C=C/C3=[N+](C4=C(C3(C)C)C=C(C=C4)S(=O)(=O)O)CCCCCC(=O)ON5C(=O)CCC5=O)(C)C |
Kanonische SMILES |
CCN1C2=C(C=C(C=C2)S(=O)(=O)[O-])C(C1=CC=CC3=[N+](C4=C(C3(C)C)C=C(C=C4)S(=O)(=O)O)CCCCCC(=O)ON5C(=O)CCC5=O)(C)C |
Reinheit |
97%min |
Herkunft des Produkts |
United States |
Retrosynthesis Analysis
AI-Powered Synthesis Planning: Our tool employs the Template_relevance Pistachio, Template_relevance Bkms_metabolic, Template_relevance Pistachio_ringbreaker, Template_relevance Reaxys, Template_relevance Reaxys_biocatalysis model, leveraging a vast database of chemical reactions to predict feasible synthetic routes.
One-Step Synthesis Focus: Specifically designed for one-step synthesis, it provides concise and direct routes for your target compounds, streamlining the synthesis process.
Accurate Predictions: Utilizing the extensive PISTACHIO, BKMS_METABOLIC, PISTACHIO_RINGBREAKER, REAXYS, REAXYS_BIOCATALYSIS database, our tool offers high-accuracy predictions, reflecting the latest in chemical research and data.
Strategy Settings
Precursor scoring | Relevance Heuristic |
---|---|
Min. plausibility | 0.01 |
Model | Template_relevance |
Template Set | Pistachio/Bkms_metabolic/Pistachio_ringbreaker/Reaxys/Reaxys_biocatalysis |
Top-N result to add to graph | 6 |
Feasible Synthetic Routes
Haftungsausschluss und Informationen zu In-Vitro-Forschungsprodukten
Bitte beachten Sie, dass alle Artikel und Produktinformationen, die auf BenchChem präsentiert werden, ausschließlich zu Informationszwecken bestimmt sind. Die auf BenchChem zum Kauf angebotenen Produkte sind speziell für In-vitro-Studien konzipiert, die außerhalb lebender Organismen durchgeführt werden. In-vitro-Studien, abgeleitet von dem lateinischen Begriff "in Glas", beinhalten Experimente, die in kontrollierten Laborumgebungen unter Verwendung von Zellen oder Geweben durchgeführt werden. Es ist wichtig zu beachten, dass diese Produkte nicht als Arzneimittel oder Medikamente eingestuft sind und keine Zulassung der FDA für die Vorbeugung, Behandlung oder Heilung von medizinischen Zuständen, Beschwerden oder Krankheiten erhalten haben. Wir müssen betonen, dass jede Form der körperlichen Einführung dieser Produkte in Menschen oder Tiere gesetzlich strikt untersagt ist. Es ist unerlässlich, sich an diese Richtlinien zu halten, um die Einhaltung rechtlicher und ethischer Standards in Forschung und Experiment zu gewährleisten.