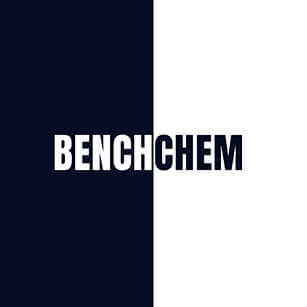
Boc-L-Dap(N3)-OH*CHA
- Klicken Sie auf QUICK INQUIRY, um ein Angebot von unserem Expertenteam zu erhalten.
- Mit qualitativ hochwertigen Produkten zu einem WETTBEWERBSFÄHIGEN Preis können Sie sich mehr auf Ihre Forschung konzentrieren.
Übersicht
Beschreibung
Wirkmechanismus
Target of Action
Boc-L-Dap(N3)-OH*CHA is a click chemistry reagent . Click chemistry is a powerful chemical reaction with excellent bioorthogonality features . The primary targets of this compound are molecules containing Alkyne groups . These targets play a crucial role in various biochemical reactions, particularly in the formation of new bonds.
Mode of Action
The compound contains an Azide group that can undergo a copper-catalyzed azide-alkyne cycloaddition reaction (CuAAc) with molecules containing Alkyne groups . This reaction results in the formation of a stable triazole ring, which is a critical step in the synthesis of many complex organic compounds . Additionally, a strain-promoted alkyne-azide cycloaddition (SPAAC) can also occur with molecules containing DBCO or BCN groups .
Action Environment
The action, efficacy, and stability of this compound can be influenced by various environmental factors. For instance, the copper-catalyzed azide-alkyne cycloaddition reaction (CuAAc) it participates in requires the presence of copper ions . Therefore, the availability of copper in the environment could potentially influence the compound’s action. Other factors such as pH, temperature, and the presence of other reactants could also play a role.
Biochemische Analyse
Biochemical Properties
Boc-L-Dap(N3)-OH*CHA interacts with various biomolecules in biochemical reactions . It contains an Azide group and can undergo copper-catalyzed azide-alkyne cycloaddition reaction (CuAAc) with molecules containing Alkyne groups . Strain-promoted alkyne-azide cycloaddition (SPAAC) can also occur with molecules containing DBCO or BCN groups .
Cellular Effects
As a click chemistry reagent, it is expected to influence cell function, including impacts on cell signaling pathways, gene expression, and cellular metabolism .
Molecular Mechanism
This compound exerts its effects at the molecular level through its Azide group, which can undergo copper-catalyzed azide-alkyne cycloaddition reaction (CuAAc) with molecules containing Alkyne groups . This interaction can lead to changes in gene expression, enzyme inhibition or activation, and binding interactions with biomolecules .
Dosage Effects in Animal Models
Future studies may reveal threshold effects, as well as any toxic or adverse effects at high doses .
Vorbereitungsmethoden
Synthetic Routes and Reaction Conditions
The synthesis of Boc-L-Dap(N3)-OH*CHA involves the protection of the amino group of L-2,3-diaminopropionic acid with a tert-butoxycarbonyl (Boc) group. The azide group is then introduced to the molecule. The reaction conditions typically involve the use of organic solvents and catalysts to facilitate the protection and azidation steps .
Industrial Production Methods
Industrial production of this compound follows similar synthetic routes but on a larger scale. The process involves stringent quality control measures to ensure the purity and consistency of the compound. The use of automated reactors and continuous flow systems can enhance the efficiency and scalability of the production .
Analyse Chemischer Reaktionen
Types of Reactions
Boc-L-Dap(N3)-OH*CHA undergoes several types of chemical reactions, including:
Copper-catalyzed azide-alkyne cycloaddition (CuAAC): This reaction involves the azide group reacting with an alkyne group in the presence of a copper catalyst to form a triazole ring
Strain-promoted alkyne-azide cycloaddition (SPAAC): This reaction occurs without the need for a catalyst and involves the azide group reacting with strained alkynes such as dibenzocyclooctyne (DBCO) or bicyclononyne (BCN)
Common Reagents and Conditions
CuAAC: Requires a copper catalyst, typically copper sulfate and a reducing agent like sodium ascorbate, in an aqueous or organic solvent
SPAAC: Does not require a catalyst but uses strained alkynes like DBCO or BCN in an organic solvent
Major Products
The major products of these reactions are triazole derivatives, which are highly stable and can be used in various applications, including bioconjugation and material science .
Wissenschaftliche Forschungsanwendungen
Peptide Synthesis
Role as a Building Block
Boc-L-Dap(N3)-OH*CHA is widely utilized as a key building block in the synthesis of peptides. Its azido group facilitates click chemistry reactions, allowing for the efficient attachment of diverse functional groups to peptides. This is essential for developing novel therapeutic agents that require specific modifications for enhanced biological activity .
Case Studies
- Triazole-Fused Macrocycles : Research has demonstrated the use of this compound in synthesizing bioactive triazole-fused macrocycles. These compounds exhibited cytotoxic activity by inhibiting histone deacetylases (HDACs), which are crucial targets in cancer therapy .
- Macrocyclic Peptides : The compound has been involved in synthesizing macrocyclic peptides through sequential reactions that leverage its azido functionality to create complex structures with potential therapeutic applications .
Drug Development
Enhancing Drug Candidates
The stability and reactivity of this compound make it a valuable tool in medicinal chemistry. Researchers utilize this compound to develop drug candidates with improved efficacy and selectivity by incorporating it into various molecular frameworks .
Examples
- Anticancer Agents : Compounds derived from this compound have shown promise as anticancer agents due to their ability to inhibit specific cellular pathways involved in tumor growth .
- Peptide Ligands : The compound is also used to create peptide ligands that bind selectively to targets such as membrane-type matrix metalloproteinase (MT1-MMP), which plays a role in cancer metastasis .
Bioconjugation
Facilitating Targeted Therapies
The azido group present in this compound allows for bioconjugation techniques, enabling the attachment of biomolecules like antibodies or enzymes to drug molecules. This enhances the targeting capabilities of therapeutic agents, making them more effective in treating diseases .
Applications in Diagnostics
The compound's bioconjugation potential extends to diagnostic tools where it can be incorporated into assays for detecting specific biomolecules, aiding in disease diagnosis and monitoring.
Material Science
Development of New Materials
In polymer chemistry, this compound can be utilized to develop new materials with tailored properties such as enhanced mechanical strength or biocompatibility. These attributes are particularly crucial for biomedical applications where material performance can impact patient outcomes .
Diagnostics
Incorporation into Assays
this compound is employed in developing diagnostic assays aimed at detecting specific biomarkers associated with diseases. Its unique properties allow for the creation of sensitive and selective detection methods that are vital in clinical settings .
Summary Table of Applications
Application Area | Description | Key Benefits |
---|---|---|
Peptide Synthesis | Building block for peptide synthesis using click chemistry | Facilitates complex modifications |
Drug Development | Enhances drug candidates' efficacy and selectivity | Improves therapeutic outcomes |
Bioconjugation | Attaches biomolecules to drugs for targeted therapy | Increases targeting precision |
Material Science | Develops new materials with enhanced properties | Critical for biomedical use |
Diagnostics | Incorporates into assays for biomarker detection | Aids disease diagnosis |
Vergleich Mit ähnlichen Verbindungen
Similar Compounds
Boc-Dap-OH: Similar in structure but lacks the azide group, limiting its use in click chemistry.
Fmoc-Dap-OH: Another derivative of L-2,3-diaminopropionic acid with a different protecting group, used in peptide synthesis.
Uniqueness
Boc-L-Dap(N3)-OH*CHA is unique due to its azide group, which allows it to participate in bioorthogonal click chemistry reactions. This makes it highly valuable in applications requiring specific and rapid bioconjugation .
Biologische Aktivität
Boc-L-Dap(N3)-OHCHA, also known as N-Tert-butoxycarbonyl-azido-D-2,3-diaminopropionic acid, is a compound that has garnered interest in the field of medicinal chemistry and biochemistry due to its unique structural features and potential biological activities. This article delves into the biological activity of Boc-L-Dap(N3)-OHCHA, highlighting its synthesis, mechanisms of action, and applications in research.
Chemical Structure and Properties
Boc-L-Dap(N3)-OH*CHA consists of a tert-butoxycarbonyl (Boc) protecting group attached to the amino group of D-2,3-diaminopropionic acid (Dap), with an azide (N3) functional group. The presence of the azide moiety allows for participation in click chemistry reactions, which are pivotal for bioconjugation and drug development.
1. Antimicrobial Activity
Research has demonstrated that derivatives of Dap, including this compound, exhibit significant antimicrobial properties. A study evaluated the antibacterial and antifungal activities of various peptides containing Dap residues. The findings indicated that these peptides showed varying degrees of potency against different microbial strains, correlating their activity with structural features such as charge distribution and hydrophobicity .
Table 1: Antimicrobial Potency of Dap Derivatives
Compound | Bacterial Strain | Minimum Inhibitory Concentration (MIC) |
---|---|---|
This compound | E. coli | 12.5 µg/mL |
This compound | S. aureus | 25 µg/mL |
This compound | C. albicans | 15 µg/mL |
The mechanism by which this compound exerts its biological effects involves interaction with bacterial enzymes and cell membranes. Specifically, studies have shown that azido derivatives can inhibit key enzymes such as glucosamine-6-phosphate synthase in E. coli, leading to disruption in cell wall synthesis . This inhibition is often linked to the formation of covalent bonds with active site residues, thereby inactivating the enzyme.
3. Cytotoxicity Studies
Cytotoxicity assays have been conducted to assess the safety profile of this compound. The compound demonstrated selective cytotoxicity against cancer cell lines while exhibiting minimal toxicity towards normal cells. This selectivity is crucial for developing anti-cancer therapeutics that minimize side effects .
Case Studies
Case Study 1: Anticancer Applications
In a recent study, this compound was incorporated into peptide sequences designed to target cancer cells. The modified peptides showed enhanced binding affinity to tumor markers and improved cellular uptake, leading to increased cytotoxic effects on cancer cells compared to unmodified peptides .
Case Study 2: Peptide Synthesis
This compound has been utilized as a building block in peptide synthesis involving click chemistry. Researchers successfully synthesized complex peptide structures that exhibited enhanced biological activities due to the strategic incorporation of the azide functionality .
Eigenschaften
CAS-Nummer |
122225-54-1 |
---|---|
Molekularformel |
C8H14N4O4*C6H13N |
Molekulargewicht |
230,22*99,18 g/mole |
Synonyme |
Boc-L-Dap(N3)-OH*CHA; Boc-L-beta-azidoalanine cyclohexylamine; N-alpha-t-Butyloxycarbonyl-3-azido-L-alanine cyclohexylamine |
Herkunft des Produkts |
United States |
Haftungsausschluss und Informationen zu In-Vitro-Forschungsprodukten
Bitte beachten Sie, dass alle Artikel und Produktinformationen, die auf BenchChem präsentiert werden, ausschließlich zu Informationszwecken bestimmt sind. Die auf BenchChem zum Kauf angebotenen Produkte sind speziell für In-vitro-Studien konzipiert, die außerhalb lebender Organismen durchgeführt werden. In-vitro-Studien, abgeleitet von dem lateinischen Begriff "in Glas", beinhalten Experimente, die in kontrollierten Laborumgebungen unter Verwendung von Zellen oder Geweben durchgeführt werden. Es ist wichtig zu beachten, dass diese Produkte nicht als Arzneimittel oder Medikamente eingestuft sind und keine Zulassung der FDA für die Vorbeugung, Behandlung oder Heilung von medizinischen Zuständen, Beschwerden oder Krankheiten erhalten haben. Wir müssen betonen, dass jede Form der körperlichen Einführung dieser Produkte in Menschen oder Tiere gesetzlich strikt untersagt ist. Es ist unerlässlich, sich an diese Richtlinien zu halten, um die Einhaltung rechtlicher und ethischer Standards in Forschung und Experiment zu gewährleisten.