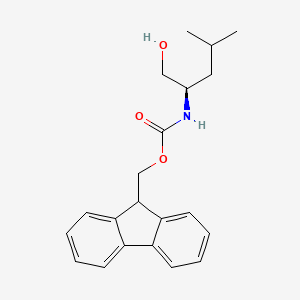
Fmoc-D-leucinol
Übersicht
Beschreibung
Fmoc-D-leucinol, or N-(9-fluorenylmethoxycarbonyl)-D-leucinol, is a chiral amino alcohol derivative widely used in peptide synthesis and medicinal chemistry. Its structure consists of a D-leucinol backbone (a leucine derivative with a hydroxyl group replacing the carboxylic acid) protected by the Fmoc group, which enhances solubility and facilitates stepwise solid-phase synthesis . Key properties include:
- Molecular Formula: C₂₁H₂₅NO₃
- Molecular Weight: 339.42 g/mol
- CAS Number: 215178-41-9
- Stereochemistry: D-configuration, which confers resistance to enzymatic degradation compared to L-isomers .
The compound’s branched hydrophobic side chain (derived from leucine) enhances peptide stability and influences secondary structure formation. NMR data (δ 0.92 ppm for methyl groups, δ 3.46–3.76 ppm for hydroxyl-associated protons) and HRMS (M+H: 340.1913) confirm its structural integrity .
Vorbereitungsmethoden
General Synthetic Approaches for Fmoc-D-Leucinol
The synthesis of this compound involves two primary steps: (1) protection of the amino group in D-leucinol using Fmoc reagents and (2) purification of the resultant product. Key strategies include classical Schotten–Baumann acylation, ionic adduct-mediated synthesis, and silylation-based methods. Each approach balances yield, purity, and scalability, with recent advances focusing on minimizing dipeptide byproducts and racemization .
Step-by-Step Synthesis via Ionic Adduct Intermediates
A widely adopted method, detailed by Nowshuddin et al., employs dicyclohexylamine (DCHA) to form stable ionic adducts with D-leucinol, moderating nucleophilicity and preventing oligomerization .
Formation of DCHA–D-Leucinol Adduct
D-leucinol reacts with DCHA in acetone at room temperature for 4–5 hours, forming a crystalline DCHA–D-leucinol salt. This intermediate enhances solubility in organic solvents and reduces side reactions during Fmoc protection.
Fmoc Protection Using Fmoc-Nsu
The DCHA–D-leucinol adduct is treated with Fmoc-N-hydroxysuccinimide ester (Fmoc-Nsu) in an alkaline aqueous-acetonitrile mixture at 0–5°C. The reaction proceeds overnight, after which acidification with 5% KHSO₄ precipitates this compound. This method achieves yields exceeding 85% with HPLC purity ≥99% .
Ethanol/Water Recrystallization for Purification
Post-synthesis purification is critical to remove unreacted Fmoc-Cl and dipeptide impurities. A patent by CN103373940B outlines a scalable recrystallization process using ethanol/water systems :
Optimized Solvent Ratios
Recrystallization efficiency depends on ethanol-to-water ratios, which vary by amino acid hydrophobicity. For this compound, a 3:2 ethanol/water ratio maximizes yield (90.3%) and purity (≥99.67%) .
Table 1: Recrystallization Parameters for this compound
Solvent Ratio (Ethanol:Water) | Concentration (g/L) | Yield (%) | Purity (%) |
---|---|---|---|
3:2 | 50.1 | 90.3 | 99.67 |
2:3 | 27.3 | 93.9 | 99.73 |
1:1 | 53.8 | 86.4 | 99.19 |
Process Details
-
Dissolution : Crude this compound is dissolved in heated (80°C) ethanol/water.
-
Crystallization : Slow cooling to room temperature induces crystallization over 12–24 hours.
-
Washing : Crystals are rinsed with the same solvent ratio to remove residual impurities.
Silylation-Mediated Fmoc Protection
An alternative method from WO1997041093A1 utilizes silylating agents to enhance Fmoc protection efficiency .
Silylation of D-Leucinol
D-leucinol is treated with 2–5 equivalents of a silylating agent (e.g., hexamethyldisilazane) in methylene chloride at 25–50°C. Silylation masks hydroxyl and amine groups, reducing unwanted side reactions.
Fmoc-OSu Coupling
The silylated intermediate reacts with 1.0–2.0 equivalents of Fmoc-Osu (Fmoc-N-succinimidyl ester) at 0–50°C. This method achieves near-quantitative yields but requires stringent anhydrous conditions .
Comparative Analysis of Synthetic Routes
Table 2: Comparison of this compound Preparation Methods
Industrial-Scale Production Considerations
Cost Optimization
Recovery of ethanol via distillation reduces solvent costs by 30–40% in large-scale operations .
Quality Control
HPLC monitoring ensures compliance with pharmacopeial standards (e.g., USP-NF), particularly for chiral purity.
Challenges and Mitigation Strategies
Racemization
Basic conditions during Fmoc protection risk racemization. Using low temperatures (0–5°C) and short reaction times preserves stereochemistry .
Byproduct Formation
Dicyclohexylurea (DCU), a common byproduct of DCHA-based methods, is removed via filtration during recrystallization .
Analyse Chemischer Reaktionen
Types of Reactions
Fmoc-D-leucinol undergoes various chemical reactions, including:
Oxidation: The hydroxyl group can be oxidized to form a carbonyl group.
Reduction: The carbonyl group can be reduced back to a hydroxyl group.
Substitution: The Fmoc group can be substituted with other protecting groups or functional groups.
Common Reagents and Conditions
Oxidation: Common oxidizing agents include potassium permanganate and chromium trioxide.
Reduction: Reducing agents such as sodium borohydride and lithium aluminum hydride are used.
Substitution: Substitution reactions often involve the use of bases like piperidine to remove the Fmoc group.
Major Products Formed
The major products formed from these reactions depend on the specific reagents and conditions used. For example, oxidation of this compound yields a carbonyl derivative, while reduction yields the original hydroxyl compound.
Wissenschaftliche Forschungsanwendungen
Peptide Synthesis
Fmoc-D-leucinol serves as a crucial building block in the synthesis of peptides. It is particularly valuable in solid-phase peptide synthesis (SPPS) , where it facilitates the efficient assembly of complex peptide sequences. The use of Fmoc (9-fluorenylmethoxycarbonyl) protects the amino group during synthesis, allowing for selective coupling reactions.
Case Study: Peptide Therapeutics
Research has demonstrated that peptides synthesized using this compound exhibit enhanced stability and bioactivity. For instance, a study on antimicrobial peptides indicated that modifications with D-leucine residues significantly improved their efficacy against resistant bacterial strains .
Drug Development
In medicinal chemistry, this compound is instrumental in designing new pharmaceuticals. Its ability to form specific interactions with biological targets makes it a valuable component in developing compounds that modulate biological pathways.
Data Table: Drug Candidates Utilizing this compound
Compound Name | Target Pathway | Application Area |
---|---|---|
Peptide A | Cancer cell apoptosis | Oncology |
Peptide B | Inflammatory response | Immunology |
Peptide C | Neurotransmitter modulation | Neurology |
Bioconjugation
This compound is employed in bioconjugation techniques that enable the attachment of biomolecules to surfaces or other molecules. This application is essential for creating targeted drug delivery systems and improving the efficacy of therapeutic agents.
Case Study: Targeted Drug Delivery Systems
A study highlighted the use of this compound in conjugating therapeutic peptides to nanoparticles for targeted cancer therapy. The modified nanoparticles demonstrated increased accumulation at tumor sites and reduced systemic toxicity .
Research in Neuroscience
The derivatives of this compound are explored for their potential therapeutic applications in neurodegenerative diseases. Research indicates that these compounds may influence neurotransmitter systems and provide insights into new treatment strategies.
Data Table: Neuroprotective Effects of this compound Derivatives
Derivative Name | Mechanism of Action | Observed Effects |
---|---|---|
Derivative X | NMDA receptor antagonist | Reduced excitotoxicity |
Derivative Y | GABA receptor modulator | Improved cognitive function |
Protein Engineering
In protein engineering, this compound aids in modifying proteins to enhance their stability and functionality for various industrial applications. This modification can lead to improved enzyme activity and resistance to denaturation.
Case Study: Enhanced Enzyme Stability
A recent study reported that enzymes engineered with this compound modifications exhibited a 30% increase in thermal stability compared to unmodified enzymes, making them more suitable for industrial processes .
Wirkmechanismus
The mechanism of action of Fmoc-D-leucinol involves the protection of the amino group through the formation of a stable carbamate linkage with the Fmoc group. This protects the amino group from unwanted reactions during peptide synthesis. The Fmoc group can be selectively removed using a base such as piperidine, allowing for the sequential addition of amino acids .
Vergleich Mit ähnlichen Verbindungen
Structural and Functional Differences
The table below compares Fmoc-D-leucinol with structurally related Fmoc-protected amino alcohols and derivatives:
Stereochemical Influence
The D-configuration in this compound distinguishes it from L-isomers like Fmoc-L-leucinol. D-amino alcohols are less common in nature but offer advantages in peptide engineering:
- Enhanced Stability : D-isomers evade proteolytic enzymes, extending peptide half-life in biological systems .
- Conformational Control : The bulky leucine side chain induces steric hindrance, promoting β-turn or helical structures in peptides .
In contrast, L-leucinol derivatives are more frequently used but require additional stabilization strategies (e.g., cyclization) to resist degradation .
Side Chain Variations
- Hydrophobicity: this compound’s branched isobutyl group increases lipophilicity compared to linear analogs like Fmoc-L-Alaninol. This property is critical for membrane permeability in drug candidates .
- Hydrogen Bonding: Fmoc-serinol’s dual hydroxyl groups enhance water solubility but reduce compatibility with hydrophobic peptide domains .
Protecting Group Strategies
- Fmoc vs. Boc: this compound uses a base-labile Fmoc group, compatible with mild deprotection (e.g., piperidine), whereas Boc-protected analogs require acidic conditions (e.g., TFA), which may degrade sensitive peptides .
Data Tables
Table 1: Physicochemical Properties
Property | This compound | Fmoc-L-leucinol | Fmoc-L-Alaninol |
---|---|---|---|
Molecular Weight | 339.42 | 339.42 | 297.35 |
Melting Point (°C) | 138 | 138 | Not reported |
LogP (Predicted) | 3.2 | 3.2 | 2.1 |
Solubility (DMF) | High | High | Moderate |
Biologische Aktivität
Fmoc-D-leucinol is a derivative of the amino acid D-leucine, commonly utilized in peptide synthesis and various biological applications. This compound has garnered attention due to its significant biological activities, particularly in antimicrobial and anticancer domains. This article provides a comprehensive overview of the biological activity of this compound, supported by data tables, case studies, and detailed research findings.
This compound is synthesized through standard peptide coupling techniques, often utilizing the Fmoc (9-fluorenylmethoxycarbonyl) protection strategy. This method allows for the selective deprotection of the amino group, facilitating the formation of peptides with specific sequences. The successful synthesis of this compound has been documented, yielding high purity and effectiveness in subsequent biological assays .
Antimicrobial Activity
Recent studies have highlighted the antimicrobial potential of D-leucine derivatives, including this compound. In a comparative analysis involving various peptides modified with D-leucine, it was observed that these modifications significantly enhanced antibacterial activity against both Gram-positive and Gram-negative bacteria.
Minimum Inhibitory Concentration (MIC) Studies
The antimicrobial efficacy was quantified using MIC assays. The results indicated that:
Peptide | MIC (µM) | Target Bacteria |
---|---|---|
B1OS | 128 | Staphylococcus aureus |
B1OS-D-L | 32 | Staphylococcus aureus |
B1OS-D-L | 64 | Escherichia coli |
Control (no D-Leu) | 128 | E. coli |
The D-leucine-modified peptides demonstrated a marked decrease in MIC values compared to their unmodified counterparts, indicating enhanced antibacterial properties .
Anticancer Activity
In addition to its antimicrobial properties, this compound exhibits promising anticancer effects. A study investigating the cytotoxicity of D-leucine-modified peptides on lung cancer cells revealed that:
- B1OS-D-L induced complete cell death in H838 lung cancer cells within 2 hours at a concentration of 10 µM.
- The cytotoxicity was significantly lower in normal cells, suggesting a selective action against cancerous tissues.
This selectivity is crucial for developing targeted cancer therapies with reduced side effects .
The mechanisms through which this compound exerts its biological effects are still under investigation. However, existing literature suggests several pathways:
- Membrane Disruption: The amphipathic nature of D-leucine derivatives allows them to integrate into bacterial membranes, leading to increased permeability and cell lysis.
- Apoptosis Induction: In cancer cells, these compounds may trigger apoptotic pathways, promoting programmed cell death while sparing normal cells.
Case Studies
Several case studies have illustrated the effectiveness of this compound in real-world applications:
-
In Vivo Efficacy Against MRSA:
- In a waxworm model infected with methicillin-resistant Staphylococcus aureus (MRSA), treatment with D-leucine-modified peptides resulted in significant survival rates compared to untreated controls. The modified peptides demonstrated rapid bactericidal activity, effectively reducing bacterial load within hours .
- Antitumor Activity in Cell Lines:
Q & A
Basic Research Questions
Q. What spectroscopic and analytical methods are recommended for characterizing Fmoc-D-leucinol?
- Methodological Answer : Characterization typically involves H NMR and C NMR to confirm structural integrity, with key peaks for the Fmoc group (e.g., aromatic protons at δ 7.28–7.76) and leucinol backbone (e.g., methyl groups at δ 0.92). High-Resolution Mass Spectrometry (HRMS) validates molecular weight (Calc’d: 340.1913; Found: 340.1699). Purity is assessed via HPLC with UV detection at 265 nm (λ_max for Fmoc) .
Q. What is the role of this compound in solid-phase peptide synthesis (SPPS)?
- Methodological Answer : this compound serves as a chiral building block to introduce D-configured leucinol residues into peptide chains. Its Fmoc group enables stepwise elongation via base-mediated deprotection (e.g., piperidine), while the hydroxyl group facilitates resin attachment or post-synthetic modifications. Optimal coupling conditions (e.g., HOBt/DIC activation) ensure high coupling efficiency .
Q. What critical parameters should be monitored during this compound synthesis?
- Methodological Answer : Key parameters include reaction temperature (ambient to 25°C for Fmoc protection), solvent choice (e.g., dichloromethane or DMF), and reaction time (typically 2–4 hours). Post-synthesis, purification via column chromatography (silica gel, ethyl acetate/hexane gradient) and lyophilization yield >90% purity. Monitor by TLC (Rf ~0.5 in 1:1 EtOAc/hexane) .
Advanced Research Questions
Q. How does the hydrophobicity of this compound influence its gelation behavior in self-assembling systems?
- Methodological Answer : Hydrophobicity (log P) governs gelation kinetics and mechanical properties. For Fmoc-dipeptides, log P < 2.8 results in syneresis gels, while 2.8 < log P < 5.5 forms stable gels at pH 4. Rheological analysis (e.g., oscillatory shear at 1 Hz) reveals storage moduli (G’) of 10–1000 Pa. Adjusting pH via glucono-δ-lactone enables controlled assembly .
Log P Range | Gel Type | Key Rheological Properties |
---|---|---|
<2.8 | Syneresis-prone gels | Low G’ (~10 Pa), rapid water expulsion |
2.8–5.5 | Self-supporting gels | High G’ (>100 Pa), pH-dependent kinetics |
Q. How can the PICOT framework structure studies on this compound’s gelation kinetics?
- Methodological Answer :
- Population : this compound derivatives (e.g., log P = 3.5–4.0).
- Intervention : pH titration (4.0–6.0) or solvent polarity modulation.
- Comparison : Fmoc-L-leucinol or hydrophobic analogs (e.g., Fmoc-FG).
- Outcome : Gel modulus (G’) and failure strain (%) via compression testing.
- Time : Real-time monitoring over 24–72 hours.
This framework ensures systematic evaluation of gelation mechanisms .
Q. How should researchers address contradictions in gelation data between this compound and its analogs?
- Methodological Answer : Contradictions often arise from differences in pKa (due to hydrophobicity) or solvent history. Mitigation strategies include:
- Standardizing gel preparation (e.g., solvent evaporation rates).
- Using controlled pH-lowering agents (e.g., glucono-δ-lactone).
- Cross-validating rheological data with microscopy (TEM/SEM) to correlate microstructure with bulk properties .
Q. What methodological approaches optimize this compound’s synthesis for high-throughput peptide libraries?
- Methodological Answer : Automated SPPS protocols using Rink amide resin, with double couplings (DIC/HOAt) to ensure >99% stepwise yield. Post-synthesis, cleavage with TFA/thioanisole/water (95:2.5:2.5) preserves stereochemistry. LC-MS monitors truncation peptides, enabling iterative optimization .
Q. How do structural modifications of this compound impact its application in peptide-based biomaterials?
- Methodological Answer : Introducing substituents (e.g., azide or allyl esters) enables click chemistry or photo-crosslinking. For example, this compound’s hydroxyl group can be functionalized with acrylates for UV-induced hydrogel formation. Such modifications alter self-assembly kinetics and biocompatibility, requiring cytotoxicity assays (e.g., MTT on NIH/3T3 cells) .
Q. What advanced statistical methods are suitable for analyzing this compound’s concentration-dependent gel modulus?
- Methodological Answer : Power-law regression () models concentration-stiffness relationships. Non-linear fitting (e.g., Levenberg-Marquardt algorithm) accounts for deviations at low concentrations. Bootstrap resampling (n=1000 iterations) quantifies confidence intervals for modulus predictions .
Q. How can researchers design comparative studies between this compound and other Fmoc-amino alcohols?
- Methodological Answer : Use a FINER (Feasible, Interesting, Novel, Ethical, Relevant) framework:
- Feasible : Compare ≤5 analogs with incremental log P differences.
- Novel : Focus on understudied D-amino acid effects on gelation.
- Ethical : Exclude in vivo testing until biocompatibility is confirmed.
- Relevant : Link findings to drug delivery or tissue engineering applications.
Pairwise ANOVA identifies significant differences in gel properties (p<0.05) .
Eigenschaften
IUPAC Name |
9H-fluoren-9-ylmethyl N-[(2R)-1-hydroxy-4-methylpentan-2-yl]carbamate | |
---|---|---|
Source | PubChem | |
URL | https://pubchem.ncbi.nlm.nih.gov | |
Description | Data deposited in or computed by PubChem | |
InChI |
InChI=1S/C21H25NO3/c1-14(2)11-15(12-23)22-21(24)25-13-20-18-9-5-3-7-16(18)17-8-4-6-10-19(17)20/h3-10,14-15,20,23H,11-13H2,1-2H3,(H,22,24)/t15-/m1/s1 | |
Source | PubChem | |
URL | https://pubchem.ncbi.nlm.nih.gov | |
Description | Data deposited in or computed by PubChem | |
InChI Key |
WXMGVJAOLIDKGZ-OAHLLOKOSA-N | |
Source | PubChem | |
URL | https://pubchem.ncbi.nlm.nih.gov | |
Description | Data deposited in or computed by PubChem | |
Canonical SMILES |
CC(C)CC(CO)NC(=O)OCC1C2=CC=CC=C2C3=CC=CC=C13 | |
Source | PubChem | |
URL | https://pubchem.ncbi.nlm.nih.gov | |
Description | Data deposited in or computed by PubChem | |
Isomeric SMILES |
CC(C)C[C@H](CO)NC(=O)OCC1C2=CC=CC=C2C3=CC=CC=C13 | |
Source | PubChem | |
URL | https://pubchem.ncbi.nlm.nih.gov | |
Description | Data deposited in or computed by PubChem | |
Molecular Formula |
C21H25NO3 | |
Source | PubChem | |
URL | https://pubchem.ncbi.nlm.nih.gov | |
Description | Data deposited in or computed by PubChem | |
Molecular Weight |
339.4 g/mol | |
Source | PubChem | |
URL | https://pubchem.ncbi.nlm.nih.gov | |
Description | Data deposited in or computed by PubChem | |
Haftungsausschluss und Informationen zu In-Vitro-Forschungsprodukten
Bitte beachten Sie, dass alle Artikel und Produktinformationen, die auf BenchChem präsentiert werden, ausschließlich zu Informationszwecken bestimmt sind. Die auf BenchChem zum Kauf angebotenen Produkte sind speziell für In-vitro-Studien konzipiert, die außerhalb lebender Organismen durchgeführt werden. In-vitro-Studien, abgeleitet von dem lateinischen Begriff "in Glas", beinhalten Experimente, die in kontrollierten Laborumgebungen unter Verwendung von Zellen oder Geweben durchgeführt werden. Es ist wichtig zu beachten, dass diese Produkte nicht als Arzneimittel oder Medikamente eingestuft sind und keine Zulassung der FDA für die Vorbeugung, Behandlung oder Heilung von medizinischen Zuständen, Beschwerden oder Krankheiten erhalten haben. Wir müssen betonen, dass jede Form der körperlichen Einführung dieser Produkte in Menschen oder Tiere gesetzlich strikt untersagt ist. Es ist unerlässlich, sich an diese Richtlinien zu halten, um die Einhaltung rechtlicher und ethischer Standards in Forschung und Experiment zu gewährleisten.