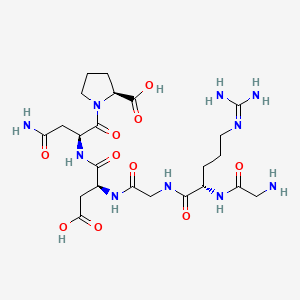
RGD-Peptid (GRGDNP)
Übersicht
Beschreibung
Die Verbindung Arginin-Glycin-Asparaginsäure-Peptid (GRGDNP) ist eine kurze Peptidsequenz, die eine entscheidende Rolle bei der Zellanhaftung, -migration und -signalisierung spielt. Sie wurde erstmals 1984 von Pierschbacher und Ruoslahti als minimale Erkennungssequenz in Fibronectin identifiziert . Diese Sequenz findet sich in den adhäsiven extrazellulären Matrizen verschiedener Zellen und wird als häufiges Zell-Erkennungsmotiv erkannt . Das Peptid hat eine hohe Affinität für den transmembranen Heterodimer-αvβ3-Integrin-Rezeptor, der auf aktiviertem neoplastischem Endothel überexprimiert wird .
Herstellungsmethoden
Synthesewege und Reaktionsbedingungen
Die Synthese von Arginin-Glycin-Asparaginsäure-Peptid (GRGDNP) erfolgt üblicherweise mittels Festphasenpeptidsynthese (SPPS). Diese Methode beinhaltet die schrittweise Addition von geschützten Aminosäuren zu einer wachsenden Peptidkette, die an einem festen Harz verankert ist . Der Prozess umfasst die folgenden Schritte:
Anlagerung der ersten Aminosäure: Die erste Aminosäure, die an der Aminogruppe geschützt ist, wird am Harz angebracht.
Entschützung: Die Schutzgruppe an der Aminogruppe wird entfernt, um die Kopplung der nächsten Aminosäure zu ermöglichen.
Kopplung: Die nächste Aminosäure, ebenfalls an der Aminogruppe geschützt, wird an die wachsende Peptidkette gekoppelt.
Wiederholung: Die Schritte 2 und 3 werden wiederholt, bis die gewünschte Peptidsequenz erhalten wird.
Spaltung: Das Peptid wird vom Harz abgespalten und entschützt, um das Endprodukt zu erhalten.
Industrielle Produktionsmethoden
In industriellen Umgebungen kann die Produktion von Arginin-Glycin-Asparaginsäure-Peptid (GRGDNP) unter Verwendung automatisierter Peptidsynthesizer, die den gleichen Prinzipien wie SPPS folgen, hochskaliert werden. Diese Maschinen können mehrere Synthesezyklen gleichzeitig verarbeiten, was die Effizienz und Ausbeute erhöht .
Chemische Reaktionsanalyse
Arten von Reaktionen
Arginin-Glycin-Asparaginsäure-Peptid (GRGDNP) unterliegt in erster Linie Reaktionen, die typisch für Peptide sind, darunter:
Hydrolyse: Abbau der Peptidbindungen in Gegenwart von Wasser.
Oxidation: Oxidation von Aminosäure-Seitenketten, insbesondere Methionin- und Cystein-Reste.
Reduktion: Reduktion von Disulfidbrücken in Cystein-Resten.
Substitution: Substitutionsreaktionen, die die Aminosäure-Seitenketten betreffen.
Häufige Reagenzien und Bedingungen
Hydrolyse: Saure oder basische Bedingungen, oft mit Salzsäure oder Natriumhydroxid.
Oxidation: Wasserstoffperoxid oder andere Oxidationsmittel.
Reduktion: Dithiothreitol (DTT) oder andere Reduktionsmittel.
Hauptprodukte, die gebildet werden
Die Hauptprodukte, die aus diesen Reaktionen gebildet werden, umfassen kleinere Peptidfragmente (aus Hydrolyse), oxidierte Aminosäuren (aus Oxidation) und reduzierte Peptide (aus Reduktion).
Wissenschaftliche Forschungsanwendungen
Arginin-Glycin-Asparaginsäure-Peptid (GRGDNP) hat eine breite Palette von Anwendungen in der wissenschaftlichen Forschung, insbesondere in den Bereichen Chemie, Biologie, Medizin und Industrie :
Chemie: Wird als Modellpeptid in Studien zur Peptidsynthese und -modifikation verwendet.
Biologie: Spielt eine Rolle in Studien zur Zellanhaftung, -migration und -signalisierung.
Medizin: Wird in gezielten Medikamententransportsystemen eingesetzt, insbesondere in der Krebstherapie, wo es die Zielfähigkeit von Nanocarriern für Integrin-Rezeptoren auf Krebszellen verbessert
Industrie: Wird bei der Entwicklung von Biomaterialien für die Gewebezüchtung und regenerative Medizin eingesetzt.
Wirkmechanismus
Der Wirkmechanismus von Arginin-Glycin-Asparaginsäure-Peptid (GRGDNP) beinhaltet seine Interaktion mit Integrin-Rezeptoren, insbesondere dem αvβ3-Integrin . Das Peptid bindet an diese Rezeptoren und hemmt deren Interaktion mit der extrazellulären Matrix (ECM). Diese Bindung fördert die Apoptose durch Aktivierung von Konformationsänderungen, die die Aktivierung und Autoverarbeitung von Pro-Caspase-3 verbessern . Das Peptid spielt auch eine entscheidende Rolle bei der Zellanhaftung, -migration, -wachstum und -differenzierung .
Wissenschaftliche Forschungsanwendungen
Arginine glycine aspartic acid peptide (GRGDNP) has a wide range of applications in scientific research, particularly in the fields of chemistry, biology, medicine, and industry :
Chemistry: Used as a model peptide in studies of peptide synthesis and modification.
Biology: Plays a role in cell adhesion, migration, and signaling studies.
Medicine: Utilized in targeted drug delivery systems, particularly in cancer therapy, where it enhances the targeting ability of nanocarriers to integrin receptors on cancer cells
Industry: Employed in the development of biomaterials for tissue engineering and regenerative medicine.
Wirkmechanismus
Target of Action
The primary target of the RGD peptide (GRGDNP) is the integrin receptor , specifically the α5β1 integrin receptor . Integrins are transmembrane receptors that facilitate cell-extracellular matrix (ECM) adhesion. The α5β1 integrin receptor is overexpressed on activated neoplastic endothelium .
Mode of Action
RGD peptide (GRGDNP) functions as an inhibitor of integrin-ligand interactions . It competitively inhibits the binding of α5β1 integrin with the extracellular matrix (ECM) . This interaction triggers conformational changes that enhance the activation and autoprocessing of pro-caspase-3 , a key enzyme in the apoptosis pathway .
Biochemical Pathways
The RGD peptide (GRGDNP) affects the apoptosis pathway . By enhancing the activation of pro-caspase-3, it promotes apoptosis, a process of programmed cell death . This action is particularly relevant in the context of cancer therapy, where promoting apoptosis in cancer cells can help control the growth and spread of tumors .
Result of Action
The primary result of the action of RGD peptide (GRGDNP) is the induction of apoptosis . By blocking the interaction between α5β1 integrin and the ECM, it triggers changes that lead to the activation of pro-caspase-3 . The activation of this enzyme then initiates the process of apoptosis, leading to cell death .
Action Environment
The action, efficacy, and stability of RGD peptide (GRGDNP) can be influenced by various environmental factors. For instance, the overexpression of α5β1 integrin on activated neoplastic endothelium can enhance the peptide’s targeting ability Additionally, the peptide’s efficacy may be influenced by the tumor microenvironment, including factors such as pH, temperature, and the presence of various enzymes
Biochemische Analyse
Biochemical Properties
The RGD peptide (GRGDNP) is involved in several biochemical reactions, primarily through its interaction with integrin receptors. Integrins are transmembrane receptors that facilitate cell-extracellular matrix adhesion. The RGD peptide binds specifically to integrins such as αvβ3, αvβ5, and α5β1, which are overexpressed in various cancer cells and activated endothelial cells. This binding is crucial for cell adhesion, migration, and signaling. The interaction between the RGD peptide and integrins involves the recognition of the RGD sequence by the integrin’s ligand-binding domain, leading to conformational changes that activate intracellular signaling pathways .
Cellular Effects
The RGD peptide (GRGDNP) has significant effects on various cell types and cellular processes. It influences cell adhesion, migration, proliferation, and apoptosis. By binding to integrin receptors, the RGD peptide can activate signaling pathways such as the focal adhesion kinase (FAK) pathway, which is involved in cell survival and migration. Additionally, the RGD peptide can induce apoptosis in certain cell types by promoting the activation of pro-caspase-3 and its subsequent autoprocessing . This peptide also affects gene expression and cellular metabolism by modulating integrin-mediated signaling pathways.
Molecular Mechanism
The molecular mechanism of action of the RGD peptide (GRGDNP) involves its binding to integrin receptors on the cell surface. This binding triggers conformational changes in the integrin, leading to the activation of intracellular signaling cascades. The RGD peptide competitively inhibits the binding of natural ligands to integrins, thereby modulating cell adhesion and migration. Additionally, the RGD peptide can induce apoptosis by enhancing the activation of pro-caspase-3 through conformational changes . These molecular interactions highlight the peptide’s role in regulating cellular functions and its potential therapeutic applications.
Temporal Effects in Laboratory Settings
In laboratory settings, the effects of the RGD peptide (GRGDNP) can vary over time. The stability and degradation of the peptide are critical factors that influence its long-term effects on cellular function. Studies have shown that the RGD peptide can maintain its activity for extended periods under controlled conditions. Its stability may be affected by factors such as temperature, pH, and enzymatic degradation . Long-term exposure to the RGD peptide has been observed to influence cell adhesion, migration, and apoptosis, with potential implications for tissue engineering and cancer therapy.
Dosage Effects in Animal Models
The effects of the RGD peptide (GRGDNP) in animal models are dose-dependent. At lower doses, the peptide can enhance cell adhesion and migration, promoting tissue repair and regeneration. At higher doses, the RGD peptide may induce apoptosis and inhibit cell proliferation, leading to potential toxic or adverse effects . These dosage effects highlight the importance of optimizing the concentration of the RGD peptide for therapeutic applications to achieve the desired outcomes without causing harm.
Metabolic Pathways
The RGD peptide (GRGDNP) is involved in various metabolic pathways, primarily through its interaction with integrin receptors. Upon binding to integrins, the peptide can modulate signaling pathways that regulate cellular metabolism, including the focal adhesion kinase (FAK) and mitogen-activated protein kinase (MAPK) pathways . These interactions can influence metabolic flux and the levels of metabolites within the cell, affecting processes such as cell growth, differentiation, and apoptosis.
Transport and Distribution
The transport and distribution of the RGD peptide (GRGDNP) within cells and tissues are mediated by its interaction with integrin receptors. Once bound to integrins, the peptide can be internalized and transported to various cellular compartments. This process is facilitated by endocytosis, where the peptide-receptor complex is engulfed by the cell membrane and transported into the cell . The distribution of the RGD peptide within tissues is influenced by factors such as receptor expression levels and the presence of extracellular matrix components.
Subcellular Localization
The subcellular localization of the RGD peptide (GRGDNP) is primarily determined by its binding to integrin receptors on the cell surface. Upon binding, the peptide can be internalized and localized to specific cellular compartments, such as endosomes and lysosomes . This localization is crucial for the peptide’s activity and function, as it allows the peptide to interact with intracellular signaling molecules and modulate cellular processes. Post-translational modifications and targeting signals may also play a role in directing the peptide to specific subcellular locations.
Vorbereitungsmethoden
Synthetic Routes and Reaction Conditions
The synthesis of arginine glycine aspartic acid peptide (GRGDNP) is typically carried out using solid-phase peptide synthesis (SPPS). This method involves the sequential addition of protected amino acids to a growing peptide chain anchored to a solid resin . The process includes the following steps:
Attachment of the first amino acid: The first amino acid, protected at the amino group, is attached to the resin.
Deprotection: The protecting group on the amino group is removed to allow the next amino acid to couple.
Coupling: The next amino acid, also protected at the amino group, is coupled to the growing peptide chain.
Repetition: Steps 2 and 3 are repeated until the desired peptide sequence is obtained.
Cleavage: The peptide is cleaved from the resin and deprotected to yield the final product.
Industrial Production Methods
In industrial settings, the production of arginine glycine aspartic acid peptide (GRGDNP) can be scaled up using automated peptide synthesizers that follow the same principles as SPPS. These machines can handle multiple synthesis cycles simultaneously, increasing efficiency and yield .
Analyse Chemischer Reaktionen
Types of Reactions
Arginine glycine aspartic acid peptide (GRGDNP) primarily undergoes reactions typical of peptides, including:
Hydrolysis: Breaking down the peptide bonds in the presence of water.
Oxidation: Oxidation of amino acid side chains, particularly methionine and cysteine residues.
Reduction: Reduction of disulfide bonds in cysteine residues.
Substitution: Substitution reactions involving the amino acid side chains.
Common Reagents and Conditions
Hydrolysis: Acidic or basic conditions, often using hydrochloric acid or sodium hydroxide.
Oxidation: Hydrogen peroxide or other oxidizing agents.
Reduction: Dithiothreitol (DTT) or other reducing agents.
Major Products Formed
The major products formed from these reactions include smaller peptide fragments (from hydrolysis), oxidized amino acids (from oxidation), and reduced peptides (from reduction).
Vergleich Mit ähnlichen Verbindungen
Arginin-Glycin-Asparaginsäure-Peptid (GRGDNP) ist einzigartig in seiner hohen Affinität für Integrin-Rezeptoren und seiner Fähigkeit, Apoptose durch spezifische molekulare Pfade zu fördern . Ähnliche Verbindungen umfassen andere RGD-Peptide und Integrin-bindende Peptide, wie zum Beispiel:
Cyclo(RGDfK): Ein cyclisches Peptid mit erhöhter Stabilität und Bindungsaffinität.
Lineare RGD-Peptide: Varianten der RGD-Sequenz mit unterschiedlichen Aminosäurezusammensetzungen.
iRGD (internalisierendes RGD): Ein Peptid, das nicht nur an Integrin-Rezeptoren bindet, sondern auch in Tumorgewebe eindringt.
Diese ähnlichen Verbindungen teilen die Fähigkeit, an Integrin-Rezeptoren zu binden, unterscheiden sich jedoch in ihrer Stabilität, Bindungsaffinität und spezifischen Anwendungen.
Eigenschaften
IUPAC Name |
(2S)-1-[(2S)-4-amino-2-[[(2S)-2-[[2-[[(2S)-2-[(2-aminoacetyl)amino]-5-(diaminomethylideneamino)pentanoyl]amino]acetyl]amino]-3-carboxypropanoyl]amino]-4-oxobutanoyl]pyrrolidine-2-carboxylic acid | |
---|---|---|
Source | PubChem | |
URL | https://pubchem.ncbi.nlm.nih.gov | |
Description | Data deposited in or computed by PubChem | |
InChI |
InChI=1S/C23H38N10O10/c24-9-16(35)30-11(3-1-5-28-23(26)27)19(39)29-10-17(36)31-12(8-18(37)38)20(40)32-13(7-15(25)34)21(41)33-6-2-4-14(33)22(42)43/h11-14H,1-10,24H2,(H2,25,34)(H,29,39)(H,30,35)(H,31,36)(H,32,40)(H,37,38)(H,42,43)(H4,26,27,28)/t11-,12-,13-,14-/m0/s1 | |
Source | PubChem | |
URL | https://pubchem.ncbi.nlm.nih.gov | |
Description | Data deposited in or computed by PubChem | |
InChI Key |
CWAHAVYVGPRZJU-XUXIUFHCSA-N | |
Source | PubChem | |
URL | https://pubchem.ncbi.nlm.nih.gov | |
Description | Data deposited in or computed by PubChem | |
Canonical SMILES |
C1CC(N(C1)C(=O)C(CC(=O)N)NC(=O)C(CC(=O)O)NC(=O)CNC(=O)C(CCCN=C(N)N)NC(=O)CN)C(=O)O | |
Source | PubChem | |
URL | https://pubchem.ncbi.nlm.nih.gov | |
Description | Data deposited in or computed by PubChem | |
Isomeric SMILES |
C1C[C@H](N(C1)C(=O)[C@H](CC(=O)N)NC(=O)[C@H](CC(=O)O)NC(=O)CNC(=O)[C@H](CCCN=C(N)N)NC(=O)CN)C(=O)O | |
Source | PubChem | |
URL | https://pubchem.ncbi.nlm.nih.gov | |
Description | Data deposited in or computed by PubChem | |
Molecular Formula |
C23H38N10O10 | |
Source | PubChem | |
URL | https://pubchem.ncbi.nlm.nih.gov | |
Description | Data deposited in or computed by PubChem | |
Molecular Weight |
614.6 g/mol | |
Source | PubChem | |
URL | https://pubchem.ncbi.nlm.nih.gov | |
Description | Data deposited in or computed by PubChem | |
Retrosynthesis Analysis
AI-Powered Synthesis Planning: Our tool employs the Template_relevance Pistachio, Template_relevance Bkms_metabolic, Template_relevance Pistachio_ringbreaker, Template_relevance Reaxys, Template_relevance Reaxys_biocatalysis model, leveraging a vast database of chemical reactions to predict feasible synthetic routes.
One-Step Synthesis Focus: Specifically designed for one-step synthesis, it provides concise and direct routes for your target compounds, streamlining the synthesis process.
Accurate Predictions: Utilizing the extensive PISTACHIO, BKMS_METABOLIC, PISTACHIO_RINGBREAKER, REAXYS, REAXYS_BIOCATALYSIS database, our tool offers high-accuracy predictions, reflecting the latest in chemical research and data.
Strategy Settings
Precursor scoring | Relevance Heuristic |
---|---|
Min. plausibility | 0.01 |
Model | Template_relevance |
Template Set | Pistachio/Bkms_metabolic/Pistachio_ringbreaker/Reaxys/Reaxys_biocatalysis |
Top-N result to add to graph | 6 |
Feasible Synthetic Routes
A: GRGDNP primarily targets integrins, specifically α5β1 integrin, which are transmembrane receptors involved in cell adhesion and signaling. [, ] By binding to these integrins, GRGDNP can interfere with their normal function. For instance, in cardiac muscle cells, GRGDNP binding to α5β1 integrin has been shown to decrease contractile force, potentially through modulation of intracellular calcium levels and myofilament activation, a process influenced by PKCε. [, ]
ANone:
ANone: Research suggests potential therapeutic applications of GRGDNP in various areas:
A: While the provided research doesn't explicitly address GRGDNP's stability, peptides in general can be susceptible to degradation. Research suggests exploring different formulations like lyophilisates, liquid buffers, or even incorporating GRGDNP into artificial mother milk for improved stability and delivery. [, , ]
Haftungsausschluss und Informationen zu In-Vitro-Forschungsprodukten
Bitte beachten Sie, dass alle Artikel und Produktinformationen, die auf BenchChem präsentiert werden, ausschließlich zu Informationszwecken bestimmt sind. Die auf BenchChem zum Kauf angebotenen Produkte sind speziell für In-vitro-Studien konzipiert, die außerhalb lebender Organismen durchgeführt werden. In-vitro-Studien, abgeleitet von dem lateinischen Begriff "in Glas", beinhalten Experimente, die in kontrollierten Laborumgebungen unter Verwendung von Zellen oder Geweben durchgeführt werden. Es ist wichtig zu beachten, dass diese Produkte nicht als Arzneimittel oder Medikamente eingestuft sind und keine Zulassung der FDA für die Vorbeugung, Behandlung oder Heilung von medizinischen Zuständen, Beschwerden oder Krankheiten erhalten haben. Wir müssen betonen, dass jede Form der körperlichen Einführung dieser Produkte in Menschen oder Tiere gesetzlich strikt untersagt ist. Es ist unerlässlich, sich an diese Richtlinien zu halten, um die Einhaltung rechtlicher und ethischer Standards in Forschung und Experiment zu gewährleisten.