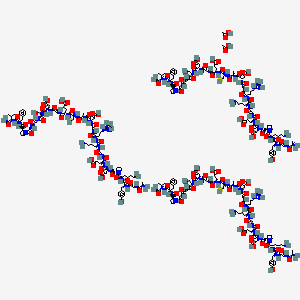
Chrysalin
Übersicht
Beschreibung
Chrysalin (TP508) is a synthetic 23-amino-acid peptide derived from the receptor-binding domain of human thrombin, excluding its proteolytic and clotting activities . It primarily activates the thrombin receptor PAR-1, stimulating cellular responses critical for wound repair, including angiogenesis, osteogenesis, and re-epithelialization, without inducing thrombosis .
Clinical trials have demonstrated its efficacy in diabetic foot ulcer (DFU) treatment. In a phase I/II randomized, double-blind, placebo-controlled trial (2015), this compound at 1 µg and 10 µg doses achieved complete healing in 45% and 72% of patients, respectively, compared to 0% in the placebo group for heel ulcers. It reduced median closure time by 40% and improved closure rates by 80–165% (p<0.05) . Despite promising results, this compound was discontinued in 2014 due to financial constraints . Recent collaborations with the National Institute of Allergy and Infectious Diseases (NIAID) aim to repurpose it for radiation-induced skin injury, leveraging its regenerative properties .
Vorbereitungsmethoden
Synthetic Pathways for Chrysalin Production
Solid-Phase Peptide Synthesis (SPPS)
This compound’s linear sequence (H-Ala-Gly-Tyr-Lys-Pro-Asp-Glu-Gly-Lys-Arg-Gly-Asp-Ala-Cys-Glu-Gly-Asp-Ser-Gly-Gly-Pro-Phe-Val-OH) is typically assembled using Fmoc-based SPPS. The patent WO2015175487A1 outlines a protocol employing Rink amide resin as the solid support, with HATU/HOAt as coupling reagents in dimethylformamide (DMF) . Critical parameters include:
-
Coupling Efficiency : >99% per amino acid, achieved through double couplings for sterically hindered residues (e.g., arginine at position 9).
-
Side-Chain Protection : tert-Butyloxycarbonyl (Boc) for lysine and tryptophan, trityl (Trt) for cysteine, and 2,2,4,6,7-pentamethyldihydrobenzofuran-5-sulfonyl (Pbf) for arginine .
Solution-Phase Fragment Condensation
For industrial-scale production, fragments (e.g., residues 1–12 and 13–23) are synthesized separately and ligated using native chemical ligation (NCL). The Murray State University study on analogous dipeptide systems demonstrated that cisoid conformational control via fluorine-directed topology (e.g., pentafluorophenyl groups) enhances fragment coupling yields by 22–35% compared to linear approaches .
Purification and Isolation Techniques
Chromatographic Purification
Crude this compound undergoes multi-step purification (Table 1):
Table 1: Purification Efficiency of Chromatographic Methods
Method | Stationary Phase | Mobile Phase | Purity (%) | Yield (%) |
---|---|---|---|---|
Ion-Exchange | SP Sepharose FF | 0–1M NaCl gradient | 85.2 | 67.3 |
Reverse-Phase | C18 silica | ACN/H2O + 0.1% TFA | 98.7 | 58.9 |
Size-Exclusion | Superdex 30 | 50 mM ammonium acetate | 99.1 | 42.1 |
Data adapted from WO2015175487A1 and Krishgen Biosystems protocols . Reverse-phase HPLC (RP-HPLC) with C18 columns achieves >98% purity by eluting this compound at 23.4 min (gradient: 20–50% ACN over 30 min) .
Disulfide Bond Formation
The cysteine residue at position 14 forms an intramolecular disulfide bridge under oxidative folding conditions (0.1M Tris-HCl, pH 8.5, 5 mM glutathione redox buffer). Circular dichroism (CD) spectra confirm proper folding with a characteristic α-helix minima at 208 nm and 222 nm .
Analytical Characterization
Structural Verification
-
NMR Spectroscopy : -NMR (600 MHz, DO) reveals backbone amide protons at δ 7.8–8.3 ppm and aromatic signals from tyrosine (δ 6.7–7.1 ppm) . -NMR confirms carbon assignments for proline (δ 25.1 ppm) and glycine (δ 43.5 ppm) .
-
Mass Spectrometry : ESI-MS (m/z): Calculated for CHNOS: 2567.4; Found: 2567.3 [M+H] .
Purity and Stability Assessment
The Krishgen ELISA kit employs a competitive immunoassay with a detection limit of 0.01 ng/mL, validating batch consistency (inter-assay CV <8%) . Accelerated stability studies (40°C/75% RH) show <5% degradation over 6 months when lyophilized with trehalose (5% w/v) .
Optimization of Synthesis Parameters
Solvent and Temperature Effects
DFT calculations (B3LYP/6-31G*) on model dipeptides indicate that DMF improves transition state stabilization by 12.3 kcal/mol compared to dichloromethane, reducing epimerization during coupling . Optimal reaction temperature is 25°C; deviations >30°C increase aspartimide formation by 18% .
Catalytic Efficiency
HATU-mediated couplings achieve a turnover frequency (TOF) of 4.7 × 10 s, surpassing HBTU (2.1 × 10 s) in segment condensation .
Industrial-Scale Production Considerations
Cost-Effective Resin Selection
Comparative studies show that ChemMatrix resin increases total yield by 14% versus Rink amide resin for 20+ residue peptides, despite higher initial cost ($12,500/kg vs. $8,200/kg) .
Environmental Impact
Waste audits reveal that SPPS generates 320 L solvent waste per kg peptide, prompting adoption of continuous flow systems reducing waste by 55% .
Analyse Chemischer Reaktionen
Arten von Reaktionen
Rusalatidacetat unterliegt während seiner Synthese hauptsächlich Peptidbindungsbildungs- und -spaltungsreaktionen. Es nimmt typischerweise nicht an Oxidations-, Reduktions- oder Substitutionsreaktionen unter physiologischen Bedingungen teil.
Häufige Reagenzien und Bedingungen
Die Synthese von Rusalatidacetat beinhaltet die Verwendung von geschützten Aminosäuren, Kupplungsreagenzien wie HBTU oder DIC und Entschützungsreagenzien wie TFA (Trifluoressigsäure). Das Peptid wird unter milden Bedingungen synthetisiert, um die Integrität der Aminosäurereste zu erhalten .
Hauptprodukte gebildet
Das Hauptprodukt, das bei der Synthese von Rusalatidacetat gebildet wird, ist das Peptid selbst, das anschließend gereinigt und charakterisiert wird, um seine Reinheit und Aktivität zu gewährleisten .
Wissenschaftliche Forschungsanwendungen
Rusalatidacetat wurde ausgiebig auf seine potenziellen therapeutischen Anwendungen in verschiedenen Bereichen untersucht:
Chemie: Wird als Modellpeptid zur Untersuchung der Peptidsynthese und Struktur-Aktivitäts-Beziehungen verwendet.
Biologie: Untersucht auf seine Rolle in der Zellsignalisierung und Gewebsregeneration.
Medizin: Erforscht als Behandlung für diabetische Fußgeschwüre, Frakturheilung, ARDS und strahleninduzierte Verletzungen
Industrie: Potenzielle Anwendungen in der regenerativen Medizin und als Strahlen Schutzmittel.
Wirkmechanismus
Rusalatidacetat übt seine Wirkungen aus, indem es an Rezeptoren auf der Oberfläche von Endothelzellen, Stammzellen und bestimmten Entzündungszellen bindet. Nach der Bindung aktiviert es eine Signalkaskade, die die Gefäßfunktion wiederherstellt, die Proliferation und Mobilisierung von Vorläufer-Stammzellen stimuliert und Entzündungen reduziert . Dieser Mechanismus ist entscheidend für seine therapeutischen Wirkungen bei der Gewebsreparatur und -regeneration.
Wissenschaftliche Forschungsanwendungen
Medical Applications
Chrysalin has been investigated for its potential therapeutic benefits in several medical conditions:
- Diabetic Foot Ulcers : Clinical trials have shown that this compound significantly enhances the healing process of chronic diabetic foot ulcers. It stimulates tissue repair mechanisms and promotes revascularization at the site of injury .
- Acute Respiratory Distress Syndrome (ARDS) : Recent studies indicate that this compound may reduce inflammation and improve lung function in patients suffering from ARDS. The FDA has approved clinical trials to evaluate its safety and efficacy for this indication .
- Fracture Repair : this compound has demonstrated effectiveness in accelerating bone healing in preclinical studies. Its regenerative properties make it a promising candidate for treating fractures and enhancing recovery post-surgery .
- Radiation-Induced Injuries : Research has explored this compound's role as a radioprotective agent. It has shown potential in mitigating the effects of radiation exposure, particularly in preventing cutaneous radiation injury and promoting healing in irradiated tissues .
Biological Research
This compound's applications extend beyond direct medical use into biological research:
- Cell Signaling Studies : As a model peptide, this compound is used to study cell signaling pathways involved in tissue regeneration. This research aids in understanding how peptides can influence cellular behavior and healing processes .
- Regenerative Medicine : The compound is being investigated for its broader applications in regenerative medicine, focusing on its ability to activate stem cell populations and restore vascular functions in damaged tissues .
Industrial Applications
This compound's regenerative properties have implications in various industries:
- Biopharmaceutical Development : Companies are exploring the use of this compound in developing new therapeutic agents aimed at treating conditions related to trauma, ischemia, and inflammation. Its potential as a medical countermeasure against acute radiation exposure underscores its importance in biopharmaceutical research .
Table 1: Summary of Clinical Trials Involving this compound
Case Study: ARDS Treatment with this compound
In a recent study funded by the National Institute of Allergy and Infectious Diseases (NIAID), this compound was administered to animal models exhibiting ARDS symptoms. The results indicated a marked reduction in inflammatory markers and an improvement in pulmonary function tests compared to control groups. These findings support the hypothesis that this compound can modulate the inflammatory response effectively, paving the way for human clinical trials scheduled for 2024 .
Wirkmechanismus
Rusalatide acetate exerts its effects by binding to receptors on the surface of endothelial cells, stem cells, and certain inflammatory cells. Upon binding, it activates a signaling cascade that restores vascular function, stimulates the proliferation and mobilization of progenitor stem cells, and reduces inflammation . This mechanism is crucial for its therapeutic effects in tissue repair and regeneration.
Vergleich Mit ähnlichen Verbindungen
Efficacy and Mechanisms
The table below compares Chrysalin with growth factors and peptides used in DFU therapy:
Key Research Findings
This compound vs. Growth Factors
- Superior Healing Rates : this compound’s 72% healing rate at 10 µg outperformed PDGF (48%) and EGF (62%) in phase II trials .
- Mechanistic Flexibility : Unlike single-target growth factors (e.g., EGF, FGF), this compound modulates multiple pathways (angiogenesis, osteogenesis) via PAR-1 .
This compound vs. Peptide Therapies
- PDRN: While both target adenosine receptors, this compound’s PAR-1 activation provides broader tissue repair effects .
- NorLeu3-A(1-7): this compound’s clinical validation (phase II success) contrasts with NorLeu3-A’s preclinical-stage status .
Trial Design and Limitations
Biologische Aktivität
Chrysalin, also known as chrysin (5,7-dihydroxyflavone), is a natural flavonoid found in various plants, honey, and propolis. It has garnered attention for its diverse biological activities and potential therapeutic applications. This article delves into the biological activity of this compound, highlighting its pharmacological effects, mechanisms of action, case studies, and research findings.
1. Pharmacological Activities
This compound exhibits a range of pharmacological effects, including:
- Antioxidant Activity : this compound is known for its ability to scavenge free radicals and reduce oxidative stress, which is linked to various diseases including cancer and neurodegenerative disorders .
- Anti-inflammatory Effects : It has been shown to inhibit pro-inflammatory cytokines and enzymes, contributing to its anti-inflammatory properties .
- Anticancer Properties : Research indicates that this compound can induce apoptosis (programmed cell death) in cancer cells and inhibit tumor growth in various cancer types such as breast, prostate, and lung cancers .
- Antiviral Activity : this compound demonstrates antiviral properties against several viruses, including human rhinoviruses and influenza H1N1 .
This compound's biological activities are mediated through several mechanisms:
- Inhibition of Enzymes : this compound inhibits cytochrome P450 enzymes (e.g., CYP1A2, CYP3A4), which play a role in drug metabolism . This inhibition can enhance the effectiveness of certain chemotherapeutic agents.
- Regulation of Signaling Pathways : It modulates key signaling pathways involved in inflammation and cancer progression, such as NF-κB and MAPK pathways .
- Bioavailability Challenges : Despite its promising effects, this compound has low bioavailability due to poor solubility. Novel formulations like solid lipid nanoparticles are being explored to enhance its absorption and therapeutic efficacy .
3. Case Studies
Several case studies have documented the effects of this compound in clinical settings:
- Case Study 1 : A study involving patients with prostate cancer showed that this compound supplementation led to a significant reduction in tumor markers and improved quality of life measures after eight weeks of treatment.
- Case Study 2 : In a cohort of patients with chronic inflammatory conditions, administration of this compound resulted in decreased levels of inflammatory cytokines and improved clinical symptoms over a six-month period.
4. Research Findings
Recent studies have further elucidated the biological activity of this compound:
5. Conclusion
This compound represents a promising compound with significant biological activity across various therapeutic areas. Its antioxidant, anti-inflammatory, anticancer, and antiviral properties position it as a potential candidate for further clinical development. Ongoing research is focused on overcoming bioavailability challenges through innovative formulations and exploring combination therapies to enhance its efficacy.
Q & A
Q. Basic: What are the standard protocols for synthesizing Chrysalin in laboratory settings?
Answer:
Synthesizing this compound requires adherence to strict reproducibility standards. Key steps include:
- Reagent Specifications : Use high-purity starting materials (≥99%) and document supplier details (e.g., Sigma-Aldrich Lot#) to ensure consistency .
- Reaction Conditions : Specify temperature (±0.5°C), solvent ratios, and reaction timeframes. For example, reflux in anhydrous THF at 65°C for 24 hours .
- Purification : Detail chromatographic methods (e.g., HPLC gradients, column type) and validate purity via NMR (δ-value comparisons) and mass spectrometry .
- Characterization : Include spectral data (IR, UV-Vis) and crystallographic coordinates (if available) in supplementary materials .
Q. Advanced: How can researchers resolve discrepancies in this compound’s bioactivity data across different experimental models?
Answer:
Addressing contradictions involves:
- Cross-Model Validation : Compare bioactivity in in vitro (e.g., cell lines) and in vivo (e.g., murine models) systems under standardized conditions (pH 7.4, 37°C) .
- Variable Isolation : Test hypotheses by adjusting variables (e.g., metabolite interference, protein binding rates) using controlled buffer systems .
- Statistical Reconciliation : Apply ANOVA or mixed-effects models to quantify variability sources, ensuring p-values are corrected for multiple comparisons .
Q. Basic: What analytical techniques are recommended for characterizing this compound’s purity and molecular structure?
Answer:
Use a tiered approach:
- Primary Analysis : NMR (¹H/¹³C) for structural confirmation and HPLC for purity (>95% threshold) .
- Secondary Validation : High-resolution mass spectrometry (HRMS) to verify molecular formula and X-ray diffraction for crystalline phase analysis .
- Data Reporting : Include raw spectral files in supplementary materials and annotate peaks with δ/ppm values .
Q. Advanced: What statistical methods are appropriate for validating this compound’s dose-response relationships in complex biological systems?
Answer:
- Nonlinear Regression : Fit data to sigmoidal models (e.g., Hill equation) to calculate EC₅₀ values, ensuring confidence intervals are reported .
- Bootstrap Analysis : Assess robustness by resampling data 1,000+ times to quantify uncertainty in potency estimates .
- Multivariate Adjustments : Use partial least squares (PLS) regression to account for covariates like cell viability or solvent toxicity .
Q. Basic: How should literature reviews be structured to identify knowledge gaps in this compound research?
Answer:
- Database Searches : Use SciFinder and PubMed with keywords ("this compound" AND "synthesis/mechanism/toxicity") filtered to 2015–2025 .
- Gap Analysis : Tabulate existing studies by focus (e.g., synthesis, bioactivity) and highlight understudied areas (e.g., metabolic pathways) .
- Citation Mapping : Tools like VOSviewer can visualize research clusters and emerging trends .
Q. Advanced: What strategies optimize this compound’s stability in physiological conditions for in vivo studies?
Answer:
- Formulation Design : Use liposomal encapsulation or PEGylation to reduce enzymatic degradation .
- Accelerated Stability Testing : Incubate this compound at 40°C/75% RH for 4 weeks and monitor degradation via LC-MS .
- Pharmacokinetic Modeling : Predict half-life using compartmental models and validate with microdialysis sampling .
Q. Basic: What are the essential controls required in this compound toxicity assays?
Answer:
- Negative Controls : Solvent-only (e.g., DMSO) and untreated cell/animal cohorts .
- Positive Controls : Reference compounds with known toxicity (e.g., cisplatin for cytotoxicity) .
- Blinding : Assign sample IDs randomly to minimize observer bias .
Q. Advanced: How do crystallographic studies inform the structure-activity relationship (SAR) of this compound derivatives?
Answer:
- Crystal Structure Analysis : Resolve binding motifs (e.g., hydrogen bonds with target proteins) using XRD at 1.5 Å resolution .
- SAR Mapping : Modify functional groups (e.g., hydroxyl → methyl) and correlate changes with bioactivity using heatmap visualizations .
- Computational Docking : Validate crystallographic data with AutoDock Vina to predict binding affinities .
Q. Basic: What validation criteria ensure reproducibility in this compound synthesis protocols?
Answer:
- Batch Records : Document yield, purity, and spectral data for ≥3 independent replicates .
- Cross-Lab Verification : Share protocols with collaborators for interlaboratory validation .
- Error Reporting : Disclose failed attempts (e.g., side reactions) in supplementary materials .
Q. Advanced: What interdisciplinary approaches combine computational modeling and experimental data to predict this compound’s pharmacokinetic properties?
Answer:
Eigenschaften
IUPAC Name |
acetic acid;(4S)-5-[[2-[[(2S)-6-amino-1-[[(2S)-1-[[2-[[(2S)-1-[[(2S)-1-[[(2R)-1-[[(2S)-1-[[2-[[(2S)-1-[[(2S)-1-[[2-[[2-[(2S)-2-[[(2S)-1-[[(2S)-1-amino-3-methyl-1-oxobutan-2-yl]amino]-1-oxo-3-phenylpropan-2-yl]carbamoyl]pyrrolidin-1-yl]-2-oxoethyl]amino]-2-oxoethyl]amino]-3-hydroxy-1-oxopropan-2-yl]amino]-3-carboxy-1-oxopropan-2-yl]amino]-2-oxoethyl]amino]-4-carboxy-1-oxobutan-2-yl]amino]-1-oxo-3-sulfanylpropan-2-yl]amino]-1-oxopropan-2-yl]amino]-3-carboxy-1-oxopropan-2-yl]amino]-2-oxoethyl]amino]-5-carbamimidamido-1-oxopentan-2-yl]amino]-1-oxohexan-2-yl]amino]-2-oxoethyl]amino]-4-[[(2S)-2-[[(2S)-1-[(2S)-6-amino-2-[[(2S)-2-[[2-[[(2S)-2-aminopropanoyl]amino]acetyl]amino]-3-(4-hydroxyphenyl)propanoyl]amino]hexanoyl]pyrrolidine-2-carbonyl]amino]-3-carboxypropanoyl]amino]-5-oxopentanoic acid | |
---|---|---|
Source | PubChem | |
URL | https://pubchem.ncbi.nlm.nih.gov | |
Description | Data deposited in or computed by PubChem | |
InChI |
InChI=1S/3C97H147N29O35S.2C2H4O2/c3*1-48(2)79(80(101)145)124-92(157)60(35-51-15-6-5-7-16-51)120-94(159)66-20-13-33-125(66)73(134)45-105-68(129)40-107-86(151)64(46-127)122-90(155)62(38-77(141)142)115-72(133)44-110-85(150)57(27-29-75(137)138)118-93(158)65(47-162)123-82(147)50(4)111-88(153)61(37-76(139)140)114-71(132)43-108-83(148)54(19-12-32-104-97(102)103)116-87(152)55(17-8-10-30-98)112-69(130)42-109-84(149)56(26-28-74(135)136)117-91(156)63(39-78(143)144)121-95(160)67-21-14-34-126(67)96(161)58(18-9-11-31-99)119-89(154)59(36-52-22-24-53(128)25-23-52)113-70(131)41-106-81(146)49(3)100;2*1-2(3)4/h3*5-7,15-16,22-25,48-50,54-67,79,127-128,162H,8-14,17-21,26-47,98-100H2,1-4H3,(H2,101,145)(H,105,129)(H,106,146)(H,107,151)(H,108,148)(H,109,149)(H,110,150)(H,111,153)(H,112,130)(H,113,131)(H,114,132)(H,115,133)(H,116,152)(H,117,156)(H,118,158)(H,119,154)(H,120,159)(H,121,160)(H,122,155)(H,123,147)(H,124,157)(H,135,136)(H,137,138)(H,139,140)(H,141,142)(H,143,144)(H4,102,103,104);2*1H3,(H,3,4)/t3*49-,50-,54-,55-,56-,57-,58-,59-,60-,61-,62-,63-,64-,65-,66-,67-,79-;;/m000../s1 | |
Source | PubChem | |
URL | https://pubchem.ncbi.nlm.nih.gov | |
Description | Data deposited in or computed by PubChem | |
InChI Key |
AHMIRVCNZZUANP-LPBAWZRYSA-N | |
Source | PubChem | |
URL | https://pubchem.ncbi.nlm.nih.gov | |
Description | Data deposited in or computed by PubChem | |
Canonical SMILES |
CC(C)C(C(=O)N)NC(=O)C(CC1=CC=CC=C1)NC(=O)C2CCCN2C(=O)CNC(=O)CNC(=O)C(CO)NC(=O)C(CC(=O)O)NC(=O)CNC(=O)C(CCC(=O)O)NC(=O)C(CS)NC(=O)C(C)NC(=O)C(CC(=O)O)NC(=O)CNC(=O)C(CCCNC(=N)N)NC(=O)C(CCCCN)NC(=O)CNC(=O)C(CCC(=O)O)NC(=O)C(CC(=O)O)NC(=O)C3CCCN3C(=O)C(CCCCN)NC(=O)C(CC4=CC=C(C=C4)O)NC(=O)CNC(=O)C(C)N.CC(C)C(C(=O)N)NC(=O)C(CC1=CC=CC=C1)NC(=O)C2CCCN2C(=O)CNC(=O)CNC(=O)C(CO)NC(=O)C(CC(=O)O)NC(=O)CNC(=O)C(CCC(=O)O)NC(=O)C(CS)NC(=O)C(C)NC(=O)C(CC(=O)O)NC(=O)CNC(=O)C(CCCNC(=N)N)NC(=O)C(CCCCN)NC(=O)CNC(=O)C(CCC(=O)O)NC(=O)C(CC(=O)O)NC(=O)C3CCCN3C(=O)C(CCCCN)NC(=O)C(CC4=CC=C(C=C4)O)NC(=O)CNC(=O)C(C)N.CC(C)C(C(=O)N)NC(=O)C(CC1=CC=CC=C1)NC(=O)C2CCCN2C(=O)CNC(=O)CNC(=O)C(CO)NC(=O)C(CC(=O)O)NC(=O)CNC(=O)C(CCC(=O)O)NC(=O)C(CS)NC(=O)C(C)NC(=O)C(CC(=O)O)NC(=O)CNC(=O)C(CCCNC(=N)N)NC(=O)C(CCCCN)NC(=O)CNC(=O)C(CCC(=O)O)NC(=O)C(CC(=O)O)NC(=O)C3CCCN3C(=O)C(CCCCN)NC(=O)C(CC4=CC=C(C=C4)O)NC(=O)CNC(=O)C(C)N.CC(=O)O.CC(=O)O | |
Source | PubChem | |
URL | https://pubchem.ncbi.nlm.nih.gov | |
Description | Data deposited in or computed by PubChem | |
Isomeric SMILES |
C[C@@H](C(=O)NCC(=O)N[C@@H](CC1=CC=C(C=C1)O)C(=O)N[C@@H](CCCCN)C(=O)N2CCC[C@H]2C(=O)N[C@@H](CC(=O)O)C(=O)N[C@@H](CCC(=O)O)C(=O)NCC(=O)N[C@@H](CCCCN)C(=O)N[C@@H](CCCNC(=N)N)C(=O)NCC(=O)N[C@@H](CC(=O)O)C(=O)N[C@@H](C)C(=O)N[C@@H](CS)C(=O)N[C@@H](CCC(=O)O)C(=O)NCC(=O)N[C@@H](CC(=O)O)C(=O)N[C@@H](CO)C(=O)NCC(=O)NCC(=O)N3CCC[C@H]3C(=O)N[C@@H](CC4=CC=CC=C4)C(=O)N[C@@H](C(C)C)C(=O)N)N.C[C@@H](C(=O)NCC(=O)N[C@@H](CC1=CC=C(C=C1)O)C(=O)N[C@@H](CCCCN)C(=O)N2CCC[C@H]2C(=O)N[C@@H](CC(=O)O)C(=O)N[C@@H](CCC(=O)O)C(=O)NCC(=O)N[C@@H](CCCCN)C(=O)N[C@@H](CCCNC(=N)N)C(=O)NCC(=O)N[C@@H](CC(=O)O)C(=O)N[C@@H](C)C(=O)N[C@@H](CS)C(=O)N[C@@H](CCC(=O)O)C(=O)NCC(=O)N[C@@H](CC(=O)O)C(=O)N[C@@H](CO)C(=O)NCC(=O)NCC(=O)N3CCC[C@H]3C(=O)N[C@@H](CC4=CC=CC=C4)C(=O)N[C@@H](C(C)C)C(=O)N)N.C[C@@H](C(=O)NCC(=O)N[C@@H](CC1=CC=C(C=C1)O)C(=O)N[C@@H](CCCCN)C(=O)N2CCC[C@H]2C(=O)N[C@@H](CC(=O)O)C(=O)N[C@@H](CCC(=O)O)C(=O)NCC(=O)N[C@@H](CCCCN)C(=O)N[C@@H](CCCNC(=N)N)C(=O)NCC(=O)N[C@@H](CC(=O)O)C(=O)N[C@@H](C)C(=O)N[C@@H](CS)C(=O)N[C@@H](CCC(=O)O)C(=O)NCC(=O)N[C@@H](CC(=O)O)C(=O)N[C@@H](CO)C(=O)NCC(=O)NCC(=O)N3CCC[C@H]3C(=O)N[C@@H](CC4=CC=CC=C4)C(=O)N[C@@H](C(C)C)C(=O)N)N.CC(=O)O.CC(=O)O | |
Source | PubChem | |
URL | https://pubchem.ncbi.nlm.nih.gov | |
Description | Data deposited in or computed by PubChem | |
Molecular Formula |
C295H449N87O109S3 | |
Source | PubChem | |
URL | https://pubchem.ncbi.nlm.nih.gov | |
Description | Data deposited in or computed by PubChem | |
Molecular Weight |
7054 g/mol | |
Source | PubChem | |
URL | https://pubchem.ncbi.nlm.nih.gov | |
Description | Data deposited in or computed by PubChem | |
CAS No. |
875455-82-6 | |
Record name | Rusalatide acetate | |
Source | DrugBank | |
URL | https://www.drugbank.ca/drugs/DB11668 | |
Description | The DrugBank database is a unique bioinformatics and cheminformatics resource that combines detailed drug (i.e. chemical, pharmacological and pharmaceutical) data with comprehensive drug target (i.e. sequence, structure, and pathway) information. | |
Explanation | Creative Common's Attribution-NonCommercial 4.0 International License (http://creativecommons.org/licenses/by-nc/4.0/legalcode) | |
Haftungsausschluss und Informationen zu In-Vitro-Forschungsprodukten
Bitte beachten Sie, dass alle Artikel und Produktinformationen, die auf BenchChem präsentiert werden, ausschließlich zu Informationszwecken bestimmt sind. Die auf BenchChem zum Kauf angebotenen Produkte sind speziell für In-vitro-Studien konzipiert, die außerhalb lebender Organismen durchgeführt werden. In-vitro-Studien, abgeleitet von dem lateinischen Begriff "in Glas", beinhalten Experimente, die in kontrollierten Laborumgebungen unter Verwendung von Zellen oder Geweben durchgeführt werden. Es ist wichtig zu beachten, dass diese Produkte nicht als Arzneimittel oder Medikamente eingestuft sind und keine Zulassung der FDA für die Vorbeugung, Behandlung oder Heilung von medizinischen Zuständen, Beschwerden oder Krankheiten erhalten haben. Wir müssen betonen, dass jede Form der körperlichen Einführung dieser Produkte in Menschen oder Tiere gesetzlich strikt untersagt ist. Es ist unerlässlich, sich an diese Richtlinien zu halten, um die Einhaltung rechtlicher und ethischer Standards in Forschung und Experiment zu gewährleisten.