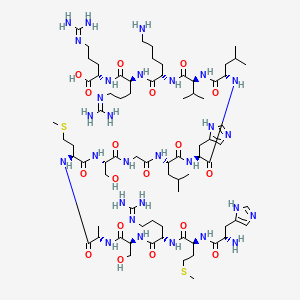
SAMS-Peptid
Übersicht
Beschreibung
The compound “SAMS Peptide” refers to peptide-based self-assembled monolayers. These are highly ordered molecular materials with versatile biochemical features and multidisciplinary applications. Self-assembled monolayers represent a significant advancement in the field of surface chemistry, providing a platform for various biological and chemical applications .
Wissenschaftliche Forschungsanwendungen
Peptide-based self-assembled monolayers have a wide range of scientific research applications, including:
Chemistry: Used as platforms for studying molecular interactions and surface chemistry.
Biology: Employed in cell adhesion, migration, and differentiation studies.
Medicine: Utilized in biosensing, drug delivery, and antimicrobial surfaces.
Industry: Applied in molecular electronics, advanced biosensing, and antifouling surfaces.
Wirkmechanismus
Target of Action
The primary target of the SAMS Peptide is the AMP-activated protein kinase (AMPK). The peptide is a substrate for this kinase and is rapidly phosphorylated by it . AMPK plays a crucial role in cellular energy homeostasis and is activated in response to stresses that deplete cellular ATP supplies such as low glucose, hypoxia, ischemia, and heat shock .
Mode of Action
The SAMS Peptide interacts with AMPK by serving as a substrate for phosphorylation . This interaction results in the activation of AMPK, which then goes on to phosphorylate a range of downstream targets, thereby regulating metabolic pathways to maintain energy homeostasis .
Biochemical Pathways
The activation of AMPK by the SAMS Peptide affects several biochemical pathways. AMPK acts as a metabolic master switch regulating several intracellular systems including the cellular uptake of glucose, the β-oxidation of fatty acids and the biogenesis of glucose transporter 4 (GLUT4) and mitochondria .
Pharmacokinetics
The pharmacokinetics of therapeutic peptides and proteins, including the SAMS Peptide, involves processes affecting their absorption, distribution, metabolism, and excretion . These processes determine the concentration of the peptide in body fluids, preferably plasma or blood, following administration of a certain dosage regimen . Understanding these properties is crucial for designing effective dosage regimens and for the rational clinical application of the peptide .
Result of Action
The molecular and cellular effects of the action of the SAMS Peptide are primarily related to its role in activating AMPK and thereby regulating energy homeostasis . This can have several downstream effects, including selective cell adhesion, migration and differentiation, biomolecular binding, advanced biosensing, molecular electronics, antimicrobial, osteointegrative and antifouling surfaces .
Action Environment
The action, efficacy, and stability of the SAMS Peptide can be influenced by various environmental factors. For instance, the peptide’s function can be affected by the presence of other molecules in its environment, the pH, temperature, and ionic strength of the solution it is in . Furthermore, the peptide can form self-assembled monolayers (SAMs) that exhibit highly ordered molecular materials with versatile biochemical features and multidisciplinary applications . These SAMs can interact with various entities, including extracellular matrix components, mammalian cells, metal ions, and biocatalytic targets .
Biochemische Analyse
Biochemical Properties
SAMS Peptide plays a crucial role in biochemical reactions, particularly in the formation of self-assembled monolayers. It interacts with various enzymes, proteins, and other biomolecules, facilitating selective cell adhesion, migration, and differentiation. The peptide’s interactions with biomolecules are highly specific, often involving binding to cell surface receptors or other proteins, which can trigger downstream signaling pathways .
Cellular Effects
SAMS Peptide significantly influences cellular processes, including cell signaling pathways, gene expression, and cellular metabolism. It has been shown to promote cell adhesion and migration, which are essential for tissue engineering and regenerative medicine applications. Additionally, SAMS Peptide can modulate gene expression by interacting with transcription factors or other regulatory proteins, thereby affecting cellular metabolism and function .
Molecular Mechanism
At the molecular level, SAMS Peptide exerts its effects through specific binding interactions with biomolecules. These interactions can lead to enzyme inhibition or activation, depending on the context. For example, SAMS Peptide can bind to cell surface receptors, triggering a cascade of intracellular signaling events that result in changes in gene expression and cellular behavior. Additionally, the peptide’s ability to form self-assembled monolayers enhances its stability and functionality in various biochemical applications .
Temporal Effects in Laboratory Settings
In laboratory settings, the effects of SAMS Peptide can change over time. The peptide’s stability and degradation are critical factors that influence its long-term effects on cellular function. Studies have shown that SAMS Peptide maintains its structural integrity and functionality over extended periods, making it suitable for long-term in vitro and in vivo studies. The peptide’s effects may diminish over time due to gradual degradation or changes in its biochemical environment .
Dosage Effects in Animal Models
The effects of SAMS Peptide vary with different dosages in animal models. At lower doses, the peptide can promote beneficial cellular responses, such as enhanced cell adhesion and migration. At higher doses, SAMS Peptide may exhibit toxic or adverse effects, including cytotoxicity or disruption of normal cellular functions. It is essential to determine the optimal dosage to achieve the desired therapeutic effects while minimizing potential side effects .
Metabolic Pathways
SAMS Peptide is involved in various metabolic pathways, interacting with enzymes and cofactors that regulate cellular metabolism. The peptide can influence metabolic flux and metabolite levels by modulating enzyme activity or gene expression. For example, SAMS Peptide may enhance the activity of enzymes involved in energy production or biosynthetic pathways, thereby supporting cellular growth and function .
Transport and Distribution
Within cells and tissues, SAMS Peptide is transported and distributed through specific transporters or binding proteins. These interactions facilitate the peptide’s localization and accumulation in target cells or tissues, enhancing its therapeutic potential. The peptide’s distribution can also be influenced by its physicochemical properties, such as size, charge, and hydrophobicity .
Subcellular Localization
SAMS Peptide’s subcellular localization is critical for its activity and function. The peptide can be directed to specific compartments or organelles through targeting signals or post-translational modifications. For example, SAMS Peptide may localize to the cell membrane, cytoplasm, or nucleus, depending on its functional role. This localization is essential for the peptide’s ability to interact with specific biomolecules and exert its biochemical effects .
Vorbereitungsmethoden
Synthetic Routes and Reaction Conditions
Peptide-based self-assembled monolayers can be synthesized using well-established synthetic chemical methods such as solid-phase peptide synthesis. This method involves the sequential addition of amino acids to a growing peptide chain, which is anchored to a solid support. The process is automated and can be performed on a large scale. The synthesized peptides are then purified using reverse-phase high-performance liquid chromatography to achieve the required purity .
Industrial Production Methods
In industrial settings, the production of peptide-based self-assembled monolayers involves the use of automated peptide synthesizers and large-scale purification systems. The peptides are synthesized in bulk and then assembled onto substrates to form the monolayers. This process ensures consistency and high throughput, making it suitable for commercial applications .
Analyse Chemischer Reaktionen
Types of Reactions
Peptide-based self-assembled monolayers undergo various chemical reactions, including:
Oxidation: Involves the addition of oxygen or the removal of hydrogen.
Reduction: Involves the addition of hydrogen or the removal of oxygen.
Substitution: Involves the replacement of one functional group with another.
Common Reagents and Conditions
Common reagents used in these reactions include oxidizing agents like hydrogen peroxide, reducing agents like sodium borohydride, and various nucleophiles and electrophiles for substitution reactions. The reactions are typically carried out under controlled conditions to ensure the stability and functionality of the monolayers .
Major Products Formed
The major products formed from these reactions depend on the specific reagents and conditions used. For example, oxidation reactions may produce oxidized peptide derivatives, while substitution reactions can introduce new functional groups into the peptide structure .
Vergleich Mit ähnlichen Verbindungen
Similar Compounds
Similar compounds to peptide-based self-assembled monolayers include:
Alkanethiol-based self-assembled monolayers: These are simpler in structure and primarily used for basic surface modifications.
Polymer-based self-assembled monolayers: These offer greater flexibility and can be tailored for specific applications.
Uniqueness
Peptide-based self-assembled monolayers are unique due to their structural complexity, stimuli-responsiveness, and biological relevance. They provide a versatile platform for a wide range of applications, from biosensing to molecular electronics, making them superior to simpler self-assembled monolayers .
Biologische Aktivität
SAMS Peptide, known scientifically as a substrate for AMP-activated protein kinase (AMPK), has garnered significant attention in recent biological research due to its unique properties and applications. This article delves into the biological activity of SAMS Peptide, highlighting its mechanisms of action, experimental findings, and implications in various fields of study.
Overview of SAMS Peptide
- Chemical Structure : SAMS Peptide (CAS 125911-68-4) is characterized by its specific amino acid sequence that allows it to interact effectively with AMPK.
- Function : It serves as a substrate for AMPK, which plays a critical role in cellular energy homeostasis. Upon phosphorylation by AMPK, SAMS Peptide becomes a valuable tool for studying AMPK activity and related metabolic processes .
AMPK is a key regulator of cellular energy metabolism. When activated, it phosphorylates various substrates, including SAMS Peptide, leading to downstream effects such as:
- Increased Fatty Acid Oxidation : AMPK activation promotes the breakdown of fatty acids, which is crucial for energy production in cells.
- Inhibition of Lipogenesis : By phosphorylating acetyl-CoA carboxylase and other enzymes involved in lipid synthesis, AMPK reduces fat storage .
- Regulation of Glucose Uptake : AMPK enhances glucose uptake by promoting the translocation of glucose transporters to the cell membrane.
Experimental Findings
Recent studies have provided insights into the biological activity and applications of SAMS Peptide:
Table 1: Summary of Experimental Studies Involving SAMS Peptide
Case Studies
-
AMPK Activation and Metabolic Regulation :
A study measured the phosphorylation levels of SAMS Peptide as an indicator of AMPK activity under different metabolic states. The results indicated a direct correlation between increased AMPK activity and enhanced phosphorylation of SAMS Peptide, suggesting its potential as a biomarker for metabolic disorders . -
Stem Cell Research :
Research involving SAMS Peptide demonstrated its utility in creating controlled environments for stem cell growth. By varying the peptide density on surfaces, researchers could manipulate stem cell behavior, promoting adhesion and differentiation into specific lineages . This has implications for tissue engineering and regenerative medicine. -
Biocompatibility Studies :
The anti-biofouling properties of SAMS-based surfaces were evaluated using various protein adsorption assays. The findings revealed that surfaces modified with SAMS Peptide exhibited significantly lower protein adsorption compared to unmodified controls, highlighting their potential use in medical devices .
Eigenschaften
IUPAC Name |
(2S)-2-[[(2S)-2-[[(2S)-6-amino-2-[[(2S)-2-[[(2S)-2-[[(2S)-2-[[(2S)-2-[[2-[[(2S)-2-[[(2S)-2-[[(2S)-2-[[(2S)-2-[[(2S)-2-[[(2S)-2-[[(2S)-2-amino-3-(1H-imidazol-5-yl)propanoyl]amino]-4-methylsulfanylbutanoyl]amino]-5-(diaminomethylideneamino)pentanoyl]amino]-3-hydroxypropanoyl]amino]propanoyl]amino]-4-methylsulfanylbutanoyl]amino]-3-hydroxypropanoyl]amino]acetyl]amino]-4-methylpentanoyl]amino]-3-(1H-imidazol-5-yl)propanoyl]amino]-4-methylpentanoyl]amino]-3-methylbutanoyl]amino]hexanoyl]amino]-5-(diaminomethylideneamino)pentanoyl]amino]-5-(diaminomethylideneamino)pentanoic acid | |
---|---|---|
Source | PubChem | |
URL | https://pubchem.ncbi.nlm.nih.gov | |
Description | Data deposited in or computed by PubChem | |
InChI |
InChI=1S/C74H131N29O18S2/c1-38(2)27-51(66(115)100-53(30-43-32-84-37-90-43)67(116)99-52(28-39(3)4)68(117)103-57(40(5)6)70(119)97-45(15-10-11-21-75)61(110)95-46(16-12-22-85-72(77)78)62(111)98-50(71(120)121)18-14-24-87-74(81)82)92-56(106)33-88-60(109)54(34-104)101-65(114)48(19-25-122-8)93-58(107)41(7)91-69(118)55(35-105)102-63(112)47(17-13-23-86-73(79)80)96-64(113)49(20-26-123-9)94-59(108)44(76)29-42-31-83-36-89-42/h31-32,36-41,44-55,57,104-105H,10-30,33-35,75-76H2,1-9H3,(H,83,89)(H,84,90)(H,88,109)(H,91,118)(H,92,106)(H,93,107)(H,94,108)(H,95,110)(H,96,113)(H,97,119)(H,98,111)(H,99,116)(H,100,115)(H,101,114)(H,102,112)(H,103,117)(H,120,121)(H4,77,78,85)(H4,79,80,86)(H4,81,82,87)/t41-,44-,45-,46-,47-,48-,49-,50-,51-,52-,53-,54-,55-,57-/m0/s1 | |
Source | PubChem | |
URL | https://pubchem.ncbi.nlm.nih.gov | |
Description | Data deposited in or computed by PubChem | |
InChI Key |
YJAHUEAICBOBTC-CSVPVIMBSA-N | |
Source | PubChem | |
URL | https://pubchem.ncbi.nlm.nih.gov | |
Description | Data deposited in or computed by PubChem | |
Canonical SMILES |
CC(C)CC(C(=O)NC(CC1=CN=CN1)C(=O)NC(CC(C)C)C(=O)NC(C(C)C)C(=O)NC(CCCCN)C(=O)NC(CCCN=C(N)N)C(=O)NC(CCCN=C(N)N)C(=O)O)NC(=O)CNC(=O)C(CO)NC(=O)C(CCSC)NC(=O)C(C)NC(=O)C(CO)NC(=O)C(CCCN=C(N)N)NC(=O)C(CCSC)NC(=O)C(CC2=CN=CN2)N | |
Source | PubChem | |
URL | https://pubchem.ncbi.nlm.nih.gov | |
Description | Data deposited in or computed by PubChem | |
Isomeric SMILES |
C[C@@H](C(=O)N[C@@H](CCSC)C(=O)N[C@@H](CO)C(=O)NCC(=O)N[C@@H](CC(C)C)C(=O)N[C@@H](CC1=CN=CN1)C(=O)N[C@@H](CC(C)C)C(=O)N[C@@H](C(C)C)C(=O)N[C@@H](CCCCN)C(=O)N[C@@H](CCCN=C(N)N)C(=O)N[C@@H](CCCN=C(N)N)C(=O)O)NC(=O)[C@H](CO)NC(=O)[C@H](CCCN=C(N)N)NC(=O)[C@H](CCSC)NC(=O)[C@H](CC2=CN=CN2)N | |
Source | PubChem | |
URL | https://pubchem.ncbi.nlm.nih.gov | |
Description | Data deposited in or computed by PubChem | |
Molecular Formula |
C74H131N29O18S2 | |
Source | PubChem | |
URL | https://pubchem.ncbi.nlm.nih.gov | |
Description | Data deposited in or computed by PubChem | |
Molecular Weight |
1779.2 g/mol | |
Source | PubChem | |
URL | https://pubchem.ncbi.nlm.nih.gov | |
Description | Data deposited in or computed by PubChem | |
Haftungsausschluss und Informationen zu In-Vitro-Forschungsprodukten
Bitte beachten Sie, dass alle Artikel und Produktinformationen, die auf BenchChem präsentiert werden, ausschließlich zu Informationszwecken bestimmt sind. Die auf BenchChem zum Kauf angebotenen Produkte sind speziell für In-vitro-Studien konzipiert, die außerhalb lebender Organismen durchgeführt werden. In-vitro-Studien, abgeleitet von dem lateinischen Begriff "in Glas", beinhalten Experimente, die in kontrollierten Laborumgebungen unter Verwendung von Zellen oder Geweben durchgeführt werden. Es ist wichtig zu beachten, dass diese Produkte nicht als Arzneimittel oder Medikamente eingestuft sind und keine Zulassung der FDA für die Vorbeugung, Behandlung oder Heilung von medizinischen Zuständen, Beschwerden oder Krankheiten erhalten haben. Wir müssen betonen, dass jede Form der körperlichen Einführung dieser Produkte in Menschen oder Tiere gesetzlich strikt untersagt ist. Es ist unerlässlich, sich an diese Richtlinien zu halten, um die Einhaltung rechtlicher und ethischer Standards in Forschung und Experiment zu gewährleisten.