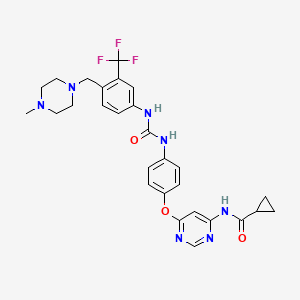
WS3
Übersicht
Beschreibung
Wolframtrisulfid ist eine anorganische Verbindung, die aus Wolfram und Schwefel besteht und die chemische Formel WS₃ hat. Es erscheint als schokoladenbraunes Pulver und ist bekannt für seine einzigartigen physikalischen und chemischen Eigenschaften .
Wirkmechanismus
Target of Action
WS3, also known as N-(6-(4-(3-(4-((4-methylpiperazin-1-yl)methyl)-3-(trifluoromethyl)phenyl)ureido)phenoxy)pyrimidin-4-yl)cyclopropanecarboxamide, primarily targets the Erb3 binding protein-1 (EBP1) and the IκB kinase pathway . EBP1 is a protein that plays a crucial role in cell proliferation and survival, while the IκB kinase pathway is involved in the regulation of immune response and inflammation .
Mode of Action
This compound interacts with its targets, EBP1 and the IκB kinase pathway, to induce cell proliferation .
Biochemical Pathways
The biochemical pathways affected by this compound are primarily related to cell proliferation and immune response, given its interaction with EBP1 and the IκB kinase pathway . The downstream effects of these interactions can lead to increased cell growth and modulation of immune responses.
Result of Action
The primary molecular and cellular effect of this compound’s action is the induction of cell proliferation . This is particularly observed in β cells, a type of cell found in the pancreas that produces insulin . This compound has been shown to induce proliferation in these cells, which could have potential implications for the treatment of conditions like diabetes .
Vorbereitungsmethoden
Synthesewege und Reaktionsbedingungen
Einleiten von Schwefelwasserstoffgas in eine heiße, angesäuerte Lösung von Wolframaten: Bei diesem Verfahren wird Schwefelwasserstoffgas durch eine heiße, angesäuerte Lösung von Wolframaten geleitet, was zur Bildung von Wolframtrisulfid führt.
Reaktion von Wolframdisulfid mit elementarem Schwefel beim Erhitzen: Wolframdisulfid reagiert beim Erhitzen mit elementarem Schwefel unter Bildung von Wolframtrisulfid.
Fällung beim Ansäuern von Thiowolframatlösungen: Das Ansäuern von Thiowolframatlösungen führt zur Fällung von Wolframtrisulfid.
Industrielle Produktionsverfahren
Industrielle Produktionsverfahren für Wolframtrisulfid ähneln den oben genannten Synthesewegen, wobei der Schwerpunkt auf der Optimierung der Reaktionsbedingungen liegt, um hohe Ausbeuten und Reinheit zu erzielen.
Analyse Chemischer Reaktionen
Arten von Reaktionen
Zersetzung: Wolframtrisulfid kann durch Erhitzen in Wolframdisulfid und elementaren Schwefel zersetzt werden.
Reaktionen mit Sulfidlösungen: Es reagiert mit Sulfidlösungen unter Bildung verschiedener Wolfram-Schwefel-Verbindungen.
Reduktion: Wolframtrisulfid kann mit Wasserstoff zu Wolframdisulfid reduziert werden.
Häufige Reagenzien und Bedingungen
Schwefelwasserstoff: Wird bei der Synthese und bei Reaktionen verwendet, die Wolframtrisulfid beinhalten.
Elementarer Schwefel: Reagiert mit Wolframdisulfid unter Bildung von Wolframtrisulfid.
Wasserstoff: Wird zur Reduktion von Wolframtrisulfid verwendet.
Hauptprodukte
Wolframdisulfid: Entsteht bei der Zersetzung und Reduktion von Wolframtrisulfid.
Elementarer Schwefel: Wird bei der Zersetzung von Wolframtrisulfid gebildet.
Wissenschaftliche Forschungsanwendungen
Elektrochemische Katalyse: Es zeigt vielversprechende Eigenschaften als Katalysator für die elektrochemische Wasserstoffentwicklungsreaktion aufgrund seiner Schichtstruktur und seiner einzigartigen Eigenschaften.
Materialwissenschaften: Seine Schichtstruktur macht es zu einem interessanten Thema für die Entwicklung neuer Materialien mit spezifischen physikalischen und chemischen Eigenschaften.
Nanotechnologie: Die Morphologie und Struktur von Wolframtrisulfid werden für Anwendungen in der Nanotechnologie untersucht.
Wirkmechanismus
Der Mechanismus, durch den Wolframtrisulfid seine Wirkung entfaltet, hängt hauptsächlich mit seiner Schichtstruktur und seiner chemischen Zusammensetzung zusammen. Seine molekularen Zielstrukturen und -wege beinhalten Wechselwirkungen mit Wasserstoff- und Schwefelatomen, die Reaktionen wie Wasserstoffentwicklung und Reduktion ermöglichen .
Vergleich Mit ähnlichen Verbindungen
Ähnliche Verbindungen
Wolframdisulfid (WS₂): Bekannt für seine Schichtstruktur und ähnliche chemische Eigenschaften.
Molybdäntrisulfid (MoS₃): Ein weiteres Übergangsmetallsulfid mit vergleichbaren Eigenschaften und Anwendungen.
Wolframtriselenid (WSe₃): Teilt ähnliche strukturelle und chemische Merkmale mit Wolframtrisulfid.
Einzigartigkeit
Wolframtrisulfid ist aufgrund seiner spezifischen Schichtstruktur und seiner Fähigkeit, als effektiver Katalysator in elektrochemischen Reaktionen zu fungieren, einzigartig. Seine ausgeprägte Morphologie, wie die wüstenrosenartige Struktur, hebt es von anderen ähnlichen Verbindungen ab .
Eigenschaften
IUPAC Name |
N-[6-[4-[[4-[(4-methylpiperazin-1-yl)methyl]-3-(trifluoromethyl)phenyl]carbamoylamino]phenoxy]pyrimidin-4-yl]cyclopropanecarboxamide | |
---|---|---|
Source | PubChem | |
URL | https://pubchem.ncbi.nlm.nih.gov | |
Description | Data deposited in or computed by PubChem | |
InChI |
InChI=1S/C28H30F3N7O3/c1-37-10-12-38(13-11-37)16-19-4-5-21(14-23(19)28(29,30)31)35-27(40)34-20-6-8-22(9-7-20)41-25-15-24(32-17-33-25)36-26(39)18-2-3-18/h4-9,14-15,17-18H,2-3,10-13,16H2,1H3,(H2,34,35,40)(H,32,33,36,39) | |
Source | PubChem | |
URL | https://pubchem.ncbi.nlm.nih.gov | |
Description | Data deposited in or computed by PubChem | |
InChI Key |
KIKOYRNAERIVSJ-UHFFFAOYSA-N | |
Source | PubChem | |
URL | https://pubchem.ncbi.nlm.nih.gov | |
Description | Data deposited in or computed by PubChem | |
Canonical SMILES |
CN1CCN(CC1)CC2=C(C=C(C=C2)NC(=O)NC3=CC=C(C=C3)OC4=NC=NC(=C4)NC(=O)C5CC5)C(F)(F)F | |
Source | PubChem | |
URL | https://pubchem.ncbi.nlm.nih.gov | |
Description | Data deposited in or computed by PubChem | |
Molecular Formula |
C28H30F3N7O3 | |
Source | PubChem | |
URL | https://pubchem.ncbi.nlm.nih.gov | |
Description | Data deposited in or computed by PubChem | |
Molecular Weight |
569.6 g/mol | |
Source | PubChem | |
URL | https://pubchem.ncbi.nlm.nih.gov | |
Description | Data deposited in or computed by PubChem | |
Retrosynthesis Analysis
AI-Powered Synthesis Planning: Our tool employs the Template_relevance Pistachio, Template_relevance Bkms_metabolic, Template_relevance Pistachio_ringbreaker, Template_relevance Reaxys, Template_relevance Reaxys_biocatalysis model, leveraging a vast database of chemical reactions to predict feasible synthetic routes.
One-Step Synthesis Focus: Specifically designed for one-step synthesis, it provides concise and direct routes for your target compounds, streamlining the synthesis process.
Accurate Predictions: Utilizing the extensive PISTACHIO, BKMS_METABOLIC, PISTACHIO_RINGBREAKER, REAXYS, REAXYS_BIOCATALYSIS database, our tool offers high-accuracy predictions, reflecting the latest in chemical research and data.
Strategy Settings
Precursor scoring | Relevance Heuristic |
---|---|
Min. plausibility | 0.01 |
Model | Template_relevance |
Template Set | Pistachio/Bkms_metabolic/Pistachio_ringbreaker/Reaxys/Reaxys_biocatalysis |
Top-N result to add to graph | 6 |
Feasible Synthetic Routes
Q1: What is tungsten trisulfide (WS3)?
A1: Tungsten trisulfide (this compound) is a chemical compound with potential applications in various fields, including catalysis and electronics.
Q2: What is the difference between amorphous this compound and crystalline this compound?
A2: Amorphous this compound lacks a well-defined, long-range ordered structure. Crystalline this compound possesses a defined structure, recently reported to be a layered structure. [, ]
Q3: What are the potential advantages of using this compound as a catalyst for the hydrogen evolution reaction (HER)?
A3: this compound exhibits promising electrocatalytic activity for HER due to its unique electronic structure and the presence of active sites on its surface. [, , ]
Q4: How does the morphology of this compound affect its catalytic performance?
A4: Research suggests that the amorphous structure of this compound, compared to crystalline WS2, leads to a larger surface area and potentially exposes more active sites for improved catalytic activity. []
Q5: What is the role of this compound in enhancing the performance of silicon nanowires for solar-driven hydrogen production?
A5: Tungsten sulfides, both in crystalline (WS2) and amorphous (this compound) forms, have been shown to enhance photoelectrochemical hydrogen production in silicon nanowires. The amorphous this compound exhibits higher catalytic activity for the hydrogen evolution reaction compared to crystalline WS2. []
Q6: Can this compound be combined with other materials to improve its catalytic properties?
A6: Yes, combining this compound with reduced graphene oxide (rGO) has shown synergistic effects, improving both its HER and oxygen reduction reaction (ORR) activities due to enhanced conductivity and increased surface area. [, ]
Q7: What are the advantages of electrochemical synthesis for producing this compound-based materials?
A7: Electrochemical synthesis offers a more environmentally friendly approach compared to traditional methods, as it reduces the use of hazardous chemicals and high-energy processes. This approach also allows for controlled synthesis and direct deposition of this compound on conductive substrates. [, ]
Q8: How does the oxidation method used to produce graphene oxide affect the properties of this compound-x/rGO hybrids?
A8: The choice of oxidation method employed during graphene oxide synthesis significantly impacts the properties of the final this compound-x/rGO hybrid. Using different oxidation techniques such as Hummers, Staudenmaier, or Hofmann methods will result in variations in the amount of metallic species present in the rGO, which in turn influences the electrocatalytic performance of the hybrid. []
Q9: What is the significance of the presence of this compound in the trigonal crystal system?
A9: The successful synthesis of crystalline this compound with a layered structure belonging to the trigonal crystal system broadens the scope of layered tungsten sulfide materials. []
Q10: How does the presence of sulfur in different oxidation states affect the properties of this compound?
A10: Studies on amorphous tungsten sulfides suggest that the presence of sulfur in both -1 and -2 oxidation states in this compound contributes to a greater degree of disorder in the W—S bonding compared to WS5. []
Haftungsausschluss und Informationen zu In-Vitro-Forschungsprodukten
Bitte beachten Sie, dass alle Artikel und Produktinformationen, die auf BenchChem präsentiert werden, ausschließlich zu Informationszwecken bestimmt sind. Die auf BenchChem zum Kauf angebotenen Produkte sind speziell für In-vitro-Studien konzipiert, die außerhalb lebender Organismen durchgeführt werden. In-vitro-Studien, abgeleitet von dem lateinischen Begriff "in Glas", beinhalten Experimente, die in kontrollierten Laborumgebungen unter Verwendung von Zellen oder Geweben durchgeführt werden. Es ist wichtig zu beachten, dass diese Produkte nicht als Arzneimittel oder Medikamente eingestuft sind und keine Zulassung der FDA für die Vorbeugung, Behandlung oder Heilung von medizinischen Zuständen, Beschwerden oder Krankheiten erhalten haben. Wir müssen betonen, dass jede Form der körperlichen Einführung dieser Produkte in Menschen oder Tiere gesetzlich strikt untersagt ist. Es ist unerlässlich, sich an diese Richtlinien zu halten, um die Einhaltung rechtlicher und ethischer Standards in Forschung und Experiment zu gewährleisten.