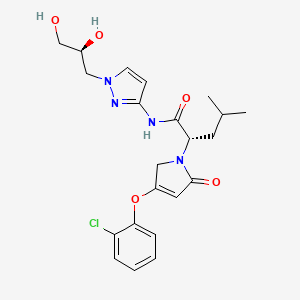
Sinogliatin
Übersicht
Beschreibung
Sinogliatin, also known as HMS-5552 and RO-5305552, is a glucokinase activator potentially for the treatment of type 2 diabetes.
Wissenschaftliche Forschungsanwendungen
Aktivierung der Glucokinase
Sinogliatin ist ein neuartiger Glucokinase-Aktivator {svg_1}. Glucokinase ist ein Enzym, das die Umwandlung von Glucose in Glucose-6-phosphat katalysiert, ein essentieller Schritt bei der Verwertung von Glucose durch den Körper. Durch die Aktivierung dieses Enzyms kann this compound zur Regulierung des Blutzuckerspiegels beitragen, was es zu einer potenziellen Behandlung für Diabetes mellitus macht {svg_2}.
Pharmakokinetische/Pharmakodynamische Modellierung
This compound wurde in Studien verwendet, um Methoden zur Vorhersage von pharmakokinetischen/pharmakodynamischen Profilen neuer Medikamente in First-in-Patient (FIP)-Studien zu entwickeln {svg_3}. Dies beinhaltet die dynamische Integration von wichtigen system- und medikamentenspezifischen Informationen in prädiktive Profile {svg_4}.
Behandlung von Typ-2-Diabetes mellitus (T2DM)
Die pharmakokinetischen/pharmakodynamischen Profile von this compound wurden bei Patienten mit T2DM untersucht {svg_5}. Die Ergebnisse dieser Studien können helfen, die Dosierung und Verabreichung von this compound bei der Behandlung von T2DM zu bestimmen {svg_6}.
Physiologisch basierte Pharmakokinetik (PBPK)-Modellierung
Ein PBPK-Modell für this compound wurde entwickelt, das allometrische Skalierung, In-vitro-zu-In-vivo-Exploration und Steady-State-Konzentration-mittlere Verweilzeit-Methoden integriert {svg_7}. Dieses Modell liefert mechanistische Einblicke in die pharmakokinetischen Eigenschaften von this compound beim Menschen {svg_8}.
Eigenschaften des Medikamentenstoffwechsels und der Pharmakokinetik (DMPK)
Es wurden Studien durchgeführt, um die DMPK-Eigenschaften von this compound zu verstehen {svg_9}. Diese Studien haben bestätigt, dass this compound überwiegend durch Cytochrom P450 (CYP) 3A4 metabolisiert wird {svg_10}.
Klinisches Design der Medikamenten-Wechselwirkungsstudie (DDI)
Das validierte PBPK-Modell von this compound wurde zur Unterstützung des Designs einer klinischen DDI-Studie verwendet {svg_11}. Dabei werden die Auswirkungen anderer Medikamente auf die Pharmakokinetik von this compound bewertet {svg_12}.
Bewertung von intrinsischen Faktoren auf die Medikamentenexposition
Das PBPK-Modell von this compound wurde auch verwendet, um die Auswirkungen von intrinsischen Faktoren wie Leberzirrhose und genetischen Faktoren auf die Medikamentenexposition zu bewerten {svg_13} {svg_14}.
Simulation der Pharmakokinetik unter Nahrungsaufnahme
Das PBPK-Modell von this compound wurde durch die Simulation seiner Pharmakokinetik unter Nahrungsaufnahme validiert {svg_15}. Dies kann helfen zu verstehen, wie die Nahrungsaufnahme die Absorption und den Stoffwechsel von this compound beeinflusst {svg_16}.
Wirkmechanismus
Target of Action
Sinogliatin primarily targets glucokinase , a rate-limiting enzyme expressed primarily in hepatocytes, and pancreatic β-cells and α-cells . Glucokinase plays a critical role in glucose homeostasis and is the primary glucose sensor that regulates insulin release by pancreatic β-cells .
Mode of Action
This compound is an oral glucokinase activator. It improves glycaemic control by activating pancreatic and hepatic glucokinase in a glucose-dependent manner . It is specifically formulated to increase the enzymatic activity of glucokinase while maintaining its glucose sensing function .
Biochemical Pathways
This compound affects the glucose homeostasis pathway. By allosterically stimulating glucokinase, it helps maintain glucose homeostasis . This action is particularly beneficial for patients with type 2 diabetes mellitus (T2DM), where the expression of glucokinase is reduced .
Pharmacokinetics
This compound’s pharmacokinetic properties have been studied using allometric scaling (AS), in vitro to in vivo exploration (IVIVE), and steady-state concentration–mean residence time (Css-MRT) methods . These studies confirmed that this compound is predominantly metabolized by cytochrome P450 (CYP) 3A4 . The Css-MRT method suggested that dog pharmacokinetic profiles were more similar to human pharmacokinetic profiles .
Result of Action
This compound has demonstrated excellent 24-hour glucose control of both fasting plasma glucose (FPG) and post-meal glucose (PMG) levels in diabetic patients . It also showed robust glucose-stimulated insulin release (GSIR), and sustained dose-proportional glucose lowering with very low risk of hypoglycemia throughout the trial .
Action Environment
The action of this compound can be influenced by various environmental factors. For instance, it was found that the pharmacokinetics of this compound could be simulated under a fed condition . The validated model was applied to support a clinical drug–drug interaction (DDI) study design and to evaluate the effects of intrinsic (hepatic cirrhosis, genetic) factors on drug exposure .
Eigenschaften
IUPAC Name |
(2S)-2-[3-(2-chlorophenoxy)-5-oxo-2H-pyrrol-1-yl]-N-[1-[(2S)-2,3-dihydroxypropyl]pyrazol-3-yl]-4-methylpentanamide | |
---|---|---|
Source | PubChem | |
URL | https://pubchem.ncbi.nlm.nih.gov | |
Description | Data deposited in or computed by PubChem | |
InChI |
InChI=1S/C22H27ClN4O5/c1-14(2)9-18(22(31)24-20-7-8-26(25-20)11-15(29)13-28)27-12-16(10-21(27)30)32-19-6-4-3-5-17(19)23/h3-8,10,14-15,18,28-29H,9,11-13H2,1-2H3,(H,24,25,31)/t15-,18-/m0/s1 | |
Source | PubChem | |
URL | https://pubchem.ncbi.nlm.nih.gov | |
Description | Data deposited in or computed by PubChem | |
InChI Key |
HMUMWSORCUWQJO-YJBOKZPZSA-N | |
Source | PubChem | |
URL | https://pubchem.ncbi.nlm.nih.gov | |
Description | Data deposited in or computed by PubChem | |
Canonical SMILES |
CC(C)CC(C(=O)NC1=NN(C=C1)CC(CO)O)N2CC(=CC2=O)OC3=CC=CC=C3Cl | |
Source | PubChem | |
URL | https://pubchem.ncbi.nlm.nih.gov | |
Description | Data deposited in or computed by PubChem | |
Isomeric SMILES |
CC(C)C[C@@H](C(=O)NC1=NN(C=C1)C[C@@H](CO)O)N2CC(=CC2=O)OC3=CC=CC=C3Cl | |
Source | PubChem | |
URL | https://pubchem.ncbi.nlm.nih.gov | |
Description | Data deposited in or computed by PubChem | |
Molecular Formula |
C22H27ClN4O5 | |
Source | PubChem | |
URL | https://pubchem.ncbi.nlm.nih.gov | |
Description | Data deposited in or computed by PubChem | |
Molecular Weight |
462.9 g/mol | |
Source | PubChem | |
URL | https://pubchem.ncbi.nlm.nih.gov | |
Description | Data deposited in or computed by PubChem | |
Retrosynthesis Analysis
AI-Powered Synthesis Planning: Our tool employs the Template_relevance Pistachio, Template_relevance Bkms_metabolic, Template_relevance Pistachio_ringbreaker, Template_relevance Reaxys, Template_relevance Reaxys_biocatalysis model, leveraging a vast database of chemical reactions to predict feasible synthetic routes.
One-Step Synthesis Focus: Specifically designed for one-step synthesis, it provides concise and direct routes for your target compounds, streamlining the synthesis process.
Accurate Predictions: Utilizing the extensive PISTACHIO, BKMS_METABOLIC, PISTACHIO_RINGBREAKER, REAXYS, REAXYS_BIOCATALYSIS database, our tool offers high-accuracy predictions, reflecting the latest in chemical research and data.
Strategy Settings
Precursor scoring | Relevance Heuristic |
---|---|
Min. plausibility | 0.01 |
Model | Template_relevance |
Template Set | Pistachio/Bkms_metabolic/Pistachio_ringbreaker/Reaxys/Reaxys_biocatalysis |
Top-N result to add to graph | 6 |
Feasible Synthetic Routes
Q1: What is the significance of developing a physiologically based pharmacokinetic (PBPK) model for Sinogliatin, especially in the early stages of drug development?
A1: Developing a PBPK model for this compound, a first-in-class glucokinase activator, is crucial in early clinical development for several reasons []. First, it allows researchers to predict the drug's behavior in the human body based on preclinical data. This can help optimize clinical trial design, including dose selection and study population. Secondly, PBPK models integrate data from various sources, such as in vitro experiments and allometric scaling, offering a mechanistic understanding of the drug's absorption, distribution, metabolism, and excretion (ADME). This approach can be particularly valuable for this compound, as it helps to understand its pharmacokinetic properties and potentially predict potential drug-drug interactions.
Q2: How does the "Learn–Research–Confirm" process described in the research contribute to translational modeling and simulation in early-phase clinical development?
A2: The "Learn–Research–Confirm" process plays a vital role in refining translational modeling and simulation, particularly for new drugs like this compound in early-phase clinical trials []. This iterative approach involves:
Haftungsausschluss und Informationen zu In-Vitro-Forschungsprodukten
Bitte beachten Sie, dass alle Artikel und Produktinformationen, die auf BenchChem präsentiert werden, ausschließlich zu Informationszwecken bestimmt sind. Die auf BenchChem zum Kauf angebotenen Produkte sind speziell für In-vitro-Studien konzipiert, die außerhalb lebender Organismen durchgeführt werden. In-vitro-Studien, abgeleitet von dem lateinischen Begriff "in Glas", beinhalten Experimente, die in kontrollierten Laborumgebungen unter Verwendung von Zellen oder Geweben durchgeführt werden. Es ist wichtig zu beachten, dass diese Produkte nicht als Arzneimittel oder Medikamente eingestuft sind und keine Zulassung der FDA für die Vorbeugung, Behandlung oder Heilung von medizinischen Zuständen, Beschwerden oder Krankheiten erhalten haben. Wir müssen betonen, dass jede Form der körperlichen Einführung dieser Produkte in Menschen oder Tiere gesetzlich strikt untersagt ist. Es ist unerlässlich, sich an diese Richtlinien zu halten, um die Einhaltung rechtlicher und ethischer Standards in Forschung und Experiment zu gewährleisten.