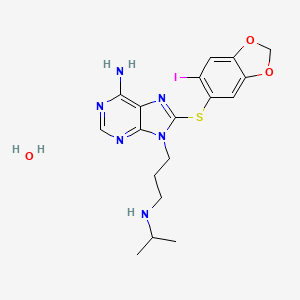
PU-H71 hydrate
- Klicken Sie auf QUICK INQUIRY, um ein Angebot von unserem Expertenteam zu erhalten.
- Mit qualitativ hochwertigen Produkten zu einem WETTBEWERBSFÄHIGEN Preis können Sie sich mehr auf Ihre Forschung konzentrieren.
Übersicht
Beschreibung
PU-H71 (Hydrat) ist ein potenter Inhibitor des Hitzeschockproteins 90 (Hsp90). Hitzeschockprotein 90 ist ein molekularer Chaperon, der bei der korrekten Faltung von Proteinen hilft, insbesondere bei solchen, die an der Transformation maligner Zellen beteiligt sind. PU-H71 hat in verschiedenen präklinischen Modellen eine signifikante therapeutische Wirksamkeit gezeigt und wird derzeit in klinischen Studien auf seine potenzielle Verwendung in der Krebstherapie untersucht .
Wirkmechanismus
Target of Action
PU-H71 hydrate is a potent inhibitor of heat shock protein 90 (Hsp90) . Hsp90 is a molecular chaperone that plays a crucial role in regulating the stability and function of many client proteins, many of which are involved in essential cellular processes .
Mode of Action
This compound interacts with its target, Hsp90, by binding to it and disrupting the epichaperome, a complex network of proteins that forms under cellular stress . This disruption leads to the degradation of client proteins, including JAK2 . JAK2 is a protein that plays a critical role in signaling pathways associated with cell growth and survival .
Biochemical Pathways
The interaction of this compound with Hsp90 affects several biochemical pathways. It has been shown to induce efficient and sustained downregulation and inactivation of proteins involved in the Ras/Raf/MAPK pathway and G2-M phase, contributing to its anti-proliferative effect . It also induces the degradation of activated Akt and Bcl-xL, leading to apoptosis . Furthermore, it inhibits activated NF- B, Akt, ERK2, Tyk2, and PKC, reducing the invasive potential of certain cancer cells .
Pharmacokinetics
In a first-in-human trial of PU-H71, the compound was administered intravenously over 1 hour on days 1 and 8 of 21-day cycles in patients with refractory solid tumors . The mean terminal half-life (T 1/2) was 8.4 ± 3.6 hours, with no dependency on the dose level . This suggests that the compound has a relatively long half-life, which could potentially allow for less frequent dosing .
Result of Action
The action of this compound results in significant molecular and cellular effects. It potently kills cancer cells via disruption of the epichaperome and degradation of JAK2 as well as other client proteins . In preclinical models, the activity of mutated JAK2 is tightly regulated by epichaperomes, and PU-H71 has been shown to disrupt this regulation . This disruption leads to the death of cancer cells .
Vorbereitungsmethoden
Synthesewege und Reaktionsbedingungen
Die Synthese von PU-H71 umfasst mehrere Schritte, beginnend mit handelsüblichen Ausgangsmaterialien. Die wichtigsten Schritte umfassen die Bildung der Kernstruktur durch eine Reihe von Kondensations- und Cyclisierungsreaktionen. Das Endprodukt wird dann gereinigt und in seine Hydratform umgewandelt. Detaillierte Synthesewege und Reaktionsbedingungen sind urheberrechtlich geschützt und in der Regel in spezialisierter chemischer Syntheseliteratur verfügbar .
Industrielle Produktionsverfahren
Die industrielle Produktion von PU-H71 (Hydrat) umfasst die Hochskalierung des Labor-Syntheseprozesses. Dazu gehört die Optimierung der Reaktionsbedingungen, um eine hohe Ausbeute und Reinheit zu gewährleisten. Der Prozess beinhaltet auch strenge Qualitätskontrollmaßnahmen, um die Konsistenz und Sicherheit des Endprodukts zu gewährleisten. Spezifische Details zu industriellen Produktionsverfahren sind oft urheberrechtlich geschützt und werden nicht öffentlich zugänglich gemacht .
Analyse Chemischer Reaktionen
Arten von Reaktionen
PU-H71 durchläuft verschiedene chemische Reaktionen, darunter:
Oxidation: PU-H71 kann unter bestimmten Bedingungen oxidiert werden, was zur Bildung oxidierter Derivate führt.
Reduktion: Reduktionsreaktionen können PU-H71 in seine reduzierte Form umwandeln.
Substitution: PU-H71 kann Substitutionsreaktionen eingehen, bei denen bestimmte funktionelle Gruppen durch andere ersetzt werden
Häufige Reagenzien und Bedingungen
Oxidation: Häufige Oxidationsmittel sind Wasserstoffperoxid und Kaliumpermanganat.
Reduktion: Reduktionsmittel wie Natriumborhydrid und Lithiumaluminiumhydrid werden verwendet.
Substitution: Verschiedene Nucleophile und Elektrophile können für Substitutionsreaktionen verwendet werden
Hauptprodukte, die gebildet werden
Die Hauptprodukte, die aus diesen Reaktionen entstehen, hängen von den spezifischen Bedingungen und Reagenzien ab, die verwendet werden. Beispielsweise kann die Oxidation zur Bildung oxidierter Derivate führen, während die Reduktion reduzierte Formen von PU-H71 liefern kann .
Wissenschaftliche Forschungsanwendungen
PU-H71 hat eine breite Palette wissenschaftlicher Forschungsanwendungen, darunter:
Chemie: Als Werkzeug zur Untersuchung der Funktion des Hitzeschockproteins 90 und seiner Rolle bei der Proteinfaltung.
Biologie: Einsatz in der Forschung, um die molekularen Mechanismen des Hitzeschockproteins 90 und seiner Client-Proteine zu verstehen.
Medizin: Untersucht auf seine potenzielle Verwendung in der Krebstherapie, insbesondere bei der Sensibilisierung von Tumorzellen für Strahlentherapie und Chemotherapie.
Industrie: Einsatz bei der Entwicklung neuer Therapeutika, die auf Hitzeschockprotein 90 abzielen .
Wirkmechanismus
PU-H71 übt seine Wirkung aus, indem es die Aktivität des Hitzeschockproteins 90 hemmt. Hitzeschockprotein 90 ist an der Faltung und Stabilisierung verschiedener Client-Proteine beteiligt, darunter solche, die das Überleben und die Proliferation von Krebszellen fördern. Durch die Hemmung des Hitzeschockproteins 90 stört PU-H71 die Funktion dieser Client-Proteine, was zur Degradation onkogener Proteine und zur Induktion von Apoptose in Krebszellen führt. Die beteiligten molekularen Ziele und Signalwege umfassen den Ras/Raf/MAPK-Signalweg, Akt und Bcl-xL .
Wissenschaftliche Forschungsanwendungen
PU-H71 has a wide range of scientific research applications, including:
Chemistry: Used as a tool to study the function of heat shock protein 90 and its role in protein folding.
Biology: Employed in research to understand the molecular mechanisms of heat shock protein 90 and its client proteins.
Medicine: Investigated for its potential use in cancer therapy, particularly in sensitizing tumor cells to radiation and chemotherapy.
Industry: Utilized in the development of new therapeutic agents targeting heat shock protein 90 .
Vergleich Mit ähnlichen Verbindungen
PU-H71 ist einzigartig unter den Inhibitoren des Hitzeschockproteins 90 aufgrund seiner hohen Potenz und Selektivität. Ähnliche Verbindungen sind:
Geldanamycin: Ein weiterer Inhibitor des Hitzeschockproteins 90, jedoch mit höherer Toxizität.
17-AAG (17-Allylamino-17-Demethoxygeldanamycin): Ein Derivat von Geldanamycin mit verbessertem Sicherheitsprofil.
NVP-AUY922: Ein synthetischer Inhibitor des Hitzeschockproteins 90 mit potenter Antikrebsaktivität .
PU-H71 zeichnet sich durch seine Fähigkeit aus, selektiv Tumorzellen anzugreifen und gleichzeitig normale Zellen zu schonen, was es zu einem vielversprechenden Kandidaten für die Krebstherapie macht .
Eigenschaften
IUPAC Name |
8-[(6-iodo-1,3-benzodioxol-5-yl)sulfanyl]-9-[3-(propan-2-ylamino)propyl]purin-6-amine;hydrate |
Source
|
---|---|---|
Source | PubChem | |
URL | https://pubchem.ncbi.nlm.nih.gov | |
Description | Data deposited in or computed by PubChem | |
InChI |
InChI=1S/C18H21IN6O2S.H2O/c1-10(2)21-4-3-5-25-17-15(16(20)22-8-23-17)24-18(25)28-14-7-13-12(6-11(14)19)26-9-27-13;/h6-8,10,21H,3-5,9H2,1-2H3,(H2,20,22,23);1H2 |
Source
|
Source | PubChem | |
URL | https://pubchem.ncbi.nlm.nih.gov | |
Description | Data deposited in or computed by PubChem | |
InChI Key |
ZUGBRIZOLBTCHT-UHFFFAOYSA-N |
Source
|
Source | PubChem | |
URL | https://pubchem.ncbi.nlm.nih.gov | |
Description | Data deposited in or computed by PubChem | |
Canonical SMILES |
CC(C)NCCCN1C2=NC=NC(=C2N=C1SC3=C(C=C4C(=C3)OCO4)I)N.O |
Source
|
Source | PubChem | |
URL | https://pubchem.ncbi.nlm.nih.gov | |
Description | Data deposited in or computed by PubChem | |
Molecular Formula |
C18H23IN6O3S |
Source
|
Source | PubChem | |
URL | https://pubchem.ncbi.nlm.nih.gov | |
Description | Data deposited in or computed by PubChem | |
Molecular Weight |
530.4 g/mol |
Source
|
Source | PubChem | |
URL | https://pubchem.ncbi.nlm.nih.gov | |
Description | Data deposited in or computed by PubChem | |
CAS No. |
1215828-29-7 |
Source
|
Record name | Zelavespib monohydrate | |
Source | ChemIDplus | |
URL | https://pubchem.ncbi.nlm.nih.gov/substance/?source=chemidplus&sourceid=1215828297 | |
Description | ChemIDplus is a free, web search system that provides access to the structure and nomenclature authority files used for the identification of chemical substances cited in National Library of Medicine (NLM) databases, including the TOXNET system. | |
Record name | ZELAVESPIB MONOHYDRATE | |
Source | FDA Global Substance Registration System (GSRS) | |
URL | https://gsrs.ncats.nih.gov/ginas/app/beta/substances/0NB0Z0L5G3 | |
Description | The FDA Global Substance Registration System (GSRS) enables the efficient and accurate exchange of information on what substances are in regulated products. Instead of relying on names, which vary across regulatory domains, countries, and regions, the GSRS knowledge base makes it possible for substances to be defined by standardized, scientific descriptions. | |
Explanation | Unless otherwise noted, the contents of the FDA website (www.fda.gov), both text and graphics, are not copyrighted. They are in the public domain and may be republished, reprinted and otherwise used freely by anyone without the need to obtain permission from FDA. Credit to the U.S. Food and Drug Administration as the source is appreciated but not required. | |
Haftungsausschluss und Informationen zu In-Vitro-Forschungsprodukten
Bitte beachten Sie, dass alle Artikel und Produktinformationen, die auf BenchChem präsentiert werden, ausschließlich zu Informationszwecken bestimmt sind. Die auf BenchChem zum Kauf angebotenen Produkte sind speziell für In-vitro-Studien konzipiert, die außerhalb lebender Organismen durchgeführt werden. In-vitro-Studien, abgeleitet von dem lateinischen Begriff "in Glas", beinhalten Experimente, die in kontrollierten Laborumgebungen unter Verwendung von Zellen oder Geweben durchgeführt werden. Es ist wichtig zu beachten, dass diese Produkte nicht als Arzneimittel oder Medikamente eingestuft sind und keine Zulassung der FDA für die Vorbeugung, Behandlung oder Heilung von medizinischen Zuständen, Beschwerden oder Krankheiten erhalten haben. Wir müssen betonen, dass jede Form der körperlichen Einführung dieser Produkte in Menschen oder Tiere gesetzlich strikt untersagt ist. Es ist unerlässlich, sich an diese Richtlinien zu halten, um die Einhaltung rechtlicher und ethischer Standards in Forschung und Experiment zu gewährleisten.