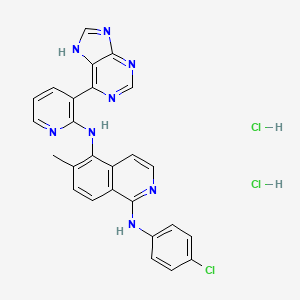
B-Raf inhibitor 1 dihydrochloride
Übersicht
Beschreibung
B-Raf-Inhibitor 1-Dihydrochlorid ist ein potenter und selektiver Inhibitor der B-Raf-Kinase, die eine entscheidende Rolle im MAPK/ERK-Signalweg spielt. Diese Verbindung ist besonders effektiv gegen die B-Raf-V600E-Mutation, eine häufige Mutation, die in verschiedenen Krebsarten vorkommt, darunter Melanom, Darmkrebs und Schilddrüsenkrebs . B-Raf-Inhibitor 1-Dihydrochlorid bindet an die DFG-out-Konformation von B-Raf, hemmt seine Aktivität und blockiert dadurch die nachgeschaltete Signalgebung, die zu Zellproliferation und -überleben führt .
Vorbereitungsmethoden
Die Synthese von B-Raf-Inhibitor 1-Dihydrochlorid umfasst mehrere Schritte, beginnend mit der Herstellung der Isochinolin-Grundstruktur. Der Syntheseweg umfasst typischerweise die folgenden Schritte:
Bildung des Isochinolin-Kerns: Die Grundstruktur wird durch eine Reihe von Reaktionen synthetisiert, darunter Cyclisierung und Modifikationen der funktionellen Gruppen.
Einführung von Substituenten: Verschiedene Substituenten, wie die 4-Chlorphenyl- und 7H-Purin-6-yl-Gruppen, werden durch nucleophile Substitution und Kupplungsreaktionen eingeführt.
Endmontage und Reinigung: Die endgültige Verbindung wird zusammengefügt, und das Dihydrochloridsalz wird durch Säure-Base-Reaktionen gebildet.
Industrielle Produktionsmethoden für B-Raf-Inhibitor 1-Dihydrochlorid sind ähnlich, aber für die großtechnische Synthese optimiert. Diese Methoden konzentrieren sich auf die Verbesserung der Ausbeute, die Reduzierung der Reaktionszeiten und die Sicherstellung der Reinheit des Endprodukts.
Analyse Chemischer Reaktionen
Key Chemical Modifications and SAR
-
Hinge-binding optimization : The 1,5-isoquinolinediamine core forms two hydrogen bonds with the hinge region (C532 backbone), while the chlorophenyl group enhances hydrophobic interactions .
-
Linker flexibility : A C₆ alkyl linker balances BRAF inhibition (IC₅₀ = 0.05 μM) and selectivity over B-Raf WT .
-
Dihydrochloride salt formation : Improves solubility (tested in vitro) and bioavailability compared to the free base .
Compound | B-Raf V600E IC₅₀ (μM) | B-Raf WT IC₅₀ (μM) |
---|---|---|
1m | 0.05 | >1.0 |
Vemurafenib | 0.13 | 0.48 |
Stability and Degradation Pathways
-
Metabolic stability : Shows resistance to CYP450-mediated oxidation in hepatic microsomes .
-
Hydrolytic susceptibility : The hydroxamic acid moiety may undergo hydrolysis under acidic conditions, requiring stabilization via salt formation .
-
Photodegradation : No data available, but analogous BRAF inhibitors show sensitivity to UV light due to aromatic heterocycles .
Mechanistic Interactions with BRAF
-
Binding mode : The purine-pyridine group occupies the ATP-binding pocket, while the isoquinoline core stabilizes the DFG-IN/αC-OUT conformation .
-
Dimerization effects : Unlike first-generation inhibitors (e.g., sorafenib), it minimizes paradoxical RAF dimerization via selective hinge binding .
Critical hydrogen bonds :
Wissenschaftliche Forschungsanwendungen
Targeted Therapy for Melanoma
B-Raf inhibitor 1 dihydrochloride has shown promise in treating melanoma patients with the V600E mutation. Studies indicate that compounds targeting this mutation can lead to significant tumor regression. For instance, clinical trials have demonstrated that patients treated with B-Raf inhibitors exhibit improved progression-free survival rates compared to those receiving conventional therapies .
Combination Therapies
Recent research emphasizes the efficacy of combining B-Raf inhibitors with MEK inhibitors. For example, the combination of encorafenib (a selective B-Raf inhibitor) and binimetinib (a MEK inhibitor) has shown enhanced antitumor activity in advanced melanoma . This synergistic approach not only improves treatment outcomes but also helps mitigate resistance associated with monotherapy.
Case Study 1: Advanced Melanoma
A patient diagnosed with advanced melanoma harboring the B-Raf V600E mutation was treated with a regimen including this compound alongside a MEK inhibitor. The treatment resulted in a partial response lasting over six months, highlighting the compound's potential in clinical settings .
Case Study 2: Colorectal Cancer
In another study focusing on colorectal cancer with B-Raf mutations, patients receiving a combination of B-Raf inhibitors and histone deacetylase inhibitors exhibited improved therapeutic responses compared to those receiving standard chemotherapy alone. The dual inhibition strategy effectively suppressed tumor growth and enhanced patient outcomes .
Data Tables
Compound | IC50 (μM) | Target | Cancer Type |
---|---|---|---|
This compound | 0.05 | B-Raf V600E | Melanoma |
Encorafenib | 0.001 | B-Raf V600E | Advanced Melanoma |
Vemurafenib | 0.13 | B-Raf V600E | Melanoma |
Dabrafenib | 0.002 | B-Raf V600E | Advanced Melanoma |
Challenges and Future Directions
Despite the promising applications of B-Raf inhibitors, challenges such as drug resistance and adverse effects remain prevalent. Ongoing research is focused on developing allosteric inhibitors that target different sites on the B-Raf protein to overcome these limitations . Additionally, exploring combination therapies with other targeted agents continues to be a significant area of investigation.
Wirkmechanismus
B-Raf inhibitor 1 dihydrochloride exerts its effects by binding to the DFG-out conformation of the B-Raf kinase, inhibiting its activity. This binding prevents the phosphorylation of downstream targets in the MAPK/ERK signaling pathway, thereby blocking cell proliferation and survival signals . The compound is particularly effective against the B-Raf V600E mutation, which leads to constitutive activation of the kinase and uncontrolled cell growth .
Vergleich Mit ähnlichen Verbindungen
B-Raf-Inhibitor 1-Dihydrochlorid ist einzigartig in seiner Selektivität und Potenz gegen die B-Raf-V600E-Mutation. Ähnliche Verbindungen umfassen:
Vemurafenib: Ein weiterer potenter B-Raf-Inhibitor, der selektiv auf die B-Raf-V600E-Mutation abzielt.
Sorafenib: Ein Multi-Kinase-Inhibitor, der auf B-Raf, C-Raf und andere Kinasen abzielt.
B-Raf-Inhibitor 1-Dihydrochlorid zeichnet sich durch seine hohe Selektivität für die B-Raf-V600E-Mutation und seine Fähigkeit aus, an die DFG-out-Konformation zu binden, was ihn zu einem wertvollen Werkzeug für die Forschung und potenzielle therapeutische Anwendungen macht .
Biologische Aktivität
B-Raf inhibitor 1 dihydrochloride is a compound that plays a significant role in cancer therapy, particularly for tumors with BRAF mutations. This article delves into its biological activity, mechanisms of action, and clinical implications, supported by data tables and relevant research findings.
Overview of B-Raf and Its Inhibition
B-Raf is a serine/threonine protein kinase that is part of the MAPK signaling pathway. Mutations in BRAF, particularly the V600E mutation, are implicated in various cancers, including melanoma. These mutations lead to constitutive activation of the kinase, resulting in uncontrolled cell proliferation and survival. B-Raf inhibitors aim to block this aberrant signaling.
Mechanism of Action
this compound functions primarily by binding to the DFG-out conformation of the ATP-binding site of B-Raf. This type IIA inhibition allows it to interfere with both mutant and wild-type B-Raf activity, although its selectivity is notably lower compared to other inhibitors. In cells expressing wild-type B-Raf, this inhibitor paradoxically activates the RAF-MEK-ERK signaling pathway due to RAF dimerization .
Inhibition Profiles
The biological activity of B-Raf inhibitor 1 can be summarized through its inhibition profiles against various forms of BRAF:
Preclinical Findings
In preclinical studies, B-Raf inhibitor 1 has shown potential for inducing hyperplasia in mouse models, indicating possible side effects that need careful consideration during therapeutic applications . Additionally, it has been noted that while it effectively inhibits mutant B-Raf, its effects on wild-type B-Raf can lead to unexpected activation of downstream signaling pathways.
Resistance Mechanisms
Despite the efficacy of B-Raf inhibitors, resistance remains a significant challenge. Studies have shown that resistance can arise from various mechanisms, including:
- Dimerization : Increased dimerization of RAF proteins can negate the effects of inhibitors.
- Upregulation of RTK signaling : This can lead to enhanced activation of downstream pathways despite the presence of inhibitors.
- Mutational changes : Secondary mutations in BRAF or other components of the MAPK pathway can confer resistance .
Clinical Applications
Clinical applications of B-Raf inhibitors have predominantly focused on melanoma patients harboring the V600E mutation. The FDA has approved several first-generation inhibitors like vemurafenib and dabrafenib; however, their effectiveness is often compromised by rapid development of resistance . The need for second-generation inhibitors like B-Raf inhibitor 1 arises from the necessity to overcome these limitations.
Eigenschaften
IUPAC Name |
1-N-(4-chlorophenyl)-6-methyl-5-N-[3-(7H-purin-6-yl)pyridin-2-yl]isoquinoline-1,5-diamine;dihydrochloride | |
---|---|---|
Source | PubChem | |
URL | https://pubchem.ncbi.nlm.nih.gov | |
Description | Data deposited in or computed by PubChem | |
InChI |
InChI=1S/C26H19ClN8.2ClH/c1-15-4-9-19-18(10-12-29-24(19)34-17-7-5-16(27)6-8-17)21(15)35-25-20(3-2-11-28-25)22-23-26(32-13-30-22)33-14-31-23;;/h2-14H,1H3,(H,28,35)(H,29,34)(H,30,31,32,33);2*1H | |
Source | PubChem | |
URL | https://pubchem.ncbi.nlm.nih.gov | |
Description | Data deposited in or computed by PubChem | |
InChI Key |
HRLQRNBAJCQMMG-UHFFFAOYSA-N | |
Source | PubChem | |
URL | https://pubchem.ncbi.nlm.nih.gov | |
Description | Data deposited in or computed by PubChem | |
Canonical SMILES |
CC1=C(C2=C(C=C1)C(=NC=C2)NC3=CC=C(C=C3)Cl)NC4=C(C=CC=N4)C5=C6C(=NC=N5)N=CN6.Cl.Cl | |
Source | PubChem | |
URL | https://pubchem.ncbi.nlm.nih.gov | |
Description | Data deposited in or computed by PubChem | |
Molecular Formula |
C26H21Cl3N8 | |
Source | PubChem | |
URL | https://pubchem.ncbi.nlm.nih.gov | |
Description | Data deposited in or computed by PubChem | |
Molecular Weight |
551.9 g/mol | |
Source | PubChem | |
URL | https://pubchem.ncbi.nlm.nih.gov | |
Description | Data deposited in or computed by PubChem | |
Retrosynthesis Analysis
AI-Powered Synthesis Planning: Our tool employs the Template_relevance Pistachio, Template_relevance Bkms_metabolic, Template_relevance Pistachio_ringbreaker, Template_relevance Reaxys, Template_relevance Reaxys_biocatalysis model, leveraging a vast database of chemical reactions to predict feasible synthetic routes.
One-Step Synthesis Focus: Specifically designed for one-step synthesis, it provides concise and direct routes for your target compounds, streamlining the synthesis process.
Accurate Predictions: Utilizing the extensive PISTACHIO, BKMS_METABOLIC, PISTACHIO_RINGBREAKER, REAXYS, REAXYS_BIOCATALYSIS database, our tool offers high-accuracy predictions, reflecting the latest in chemical research and data.
Strategy Settings
Precursor scoring | Relevance Heuristic |
---|---|
Min. plausibility | 0.01 |
Model | Template_relevance |
Template Set | Pistachio/Bkms_metabolic/Pistachio_ringbreaker/Reaxys/Reaxys_biocatalysis |
Top-N result to add to graph | 6 |
Feasible Synthetic Routes
Haftungsausschluss und Informationen zu In-Vitro-Forschungsprodukten
Bitte beachten Sie, dass alle Artikel und Produktinformationen, die auf BenchChem präsentiert werden, ausschließlich zu Informationszwecken bestimmt sind. Die auf BenchChem zum Kauf angebotenen Produkte sind speziell für In-vitro-Studien konzipiert, die außerhalb lebender Organismen durchgeführt werden. In-vitro-Studien, abgeleitet von dem lateinischen Begriff "in Glas", beinhalten Experimente, die in kontrollierten Laborumgebungen unter Verwendung von Zellen oder Geweben durchgeführt werden. Es ist wichtig zu beachten, dass diese Produkte nicht als Arzneimittel oder Medikamente eingestuft sind und keine Zulassung der FDA für die Vorbeugung, Behandlung oder Heilung von medizinischen Zuständen, Beschwerden oder Krankheiten erhalten haben. Wir müssen betonen, dass jede Form der körperlichen Einführung dieser Produkte in Menschen oder Tiere gesetzlich strikt untersagt ist. Es ist unerlässlich, sich an diese Richtlinien zu halten, um die Einhaltung rechtlicher und ethischer Standards in Forschung und Experiment zu gewährleisten.