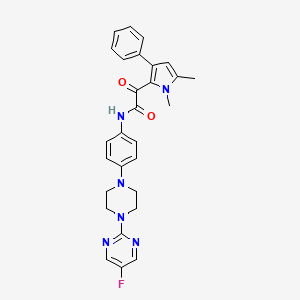
Olorofim
Übersicht
Beschreibung
Olorofim ist eine experimentelle Antimykotikum-Verbindung, die zur Klasse der Orotomide gehört. Es wird zur Behandlung invasiver Schimmelpilzinfektionen entwickelt, insbesondere solcher, die durch Arten verursacht werden, die gegenüber bestehenden Antimykotika resistent sind. This compound hemmt Dihydroorotat-Dehydrogenase, ein Enzym, das für die Biosynthese von Pyrimidinen entscheidend ist, die für die DNA- und RNA-Synthese von Pilzen essentiell sind .
Herstellungsmethoden
Synthesewege und Reaktionsbedingungen: Die Synthese von this compound umfasst mehrere Schritte, beginnend mit kommerziell erhältlichen Ausgangsmaterialien. Zu den wichtigsten Schritten gehören die Bildung der Orotomid-Kernstruktur, gefolgt von Modifikationen der funktionellen Gruppen, um die Antimykotika-Aktivität zu verstärken. Spezifische Reaktionsbedingungen wie Temperatur, Lösungsmittel und Katalysatoren werden optimiert, um hohe Ausbeuten und Reinheit zu erzielen .
Industrielle Produktionsmethoden: Die industrielle Produktion von this compound umfasst die großtechnische Synthese unter Verwendung optimierter Synthesewege. Der Prozess umfasst strenge Qualitätskontrollmaßnahmen, um die Konsistenz und Wirksamkeit des Endprodukts zu gewährleisten. Die Produktionsmethoden sind so konzipiert, dass sie kostengünstig und skalierbar sind, um den Anforderungen der klinischen und kommerziellen Anwendung gerecht zu werden .
Chemische Reaktionsanalyse
Arten von Reaktionen: this compound unterliegt verschiedenen chemischen Reaktionen, darunter Oxidation, Reduktion und Substitution. Diese Reaktionen sind wichtig, um die Struktur der Verbindung zu modifizieren, um ihre antimykotischen Eigenschaften zu verbessern .
Häufige Reagenzien und Bedingungen: Häufige Reagenzien, die bei der Synthese und Modifikation von this compound verwendet werden, umfassen Oxidationsmittel, Reduktionsmittel und Nucleophile. Reaktionsbedingungen wie Temperatur, pH-Wert und die Wahl des Lösungsmittels werden sorgfältig gesteuert, um die gewünschten chemischen Transformationen zu erreichen .
Hauptprodukte, die gebildet werden: Die Hauptprodukte, die aus den chemischen Reaktionen von this compound gebildet werden, umfassen verschiedene Derivate mit verbesserter antimykotischer Aktivität. Diese Derivate werden auf ihre Wirksamkeit gegen eine Reihe von Pilzpathogenen getestet, um die potentesten Verbindungen zu identifizieren .
Wissenschaftliche Forschungsanwendungen
This compound hat in der wissenschaftlichen Forschung vielversprechende Ergebnisse gezeigt, insbesondere im Bereich der Antimykotika-Therapie. Es ist wirksam gegen eine breite Palette von Schimmelpilzarten, einschließlich solcher, die gegen Azole und Amphotericin B resistent sind. This compound wird sowohl zur oralen als auch zur intravenösen Verabreichung entwickelt, was es für verschiedene klinische Szenarien vielseitig einsetzbar macht .
Sein einzigartiger Wirkmechanismus macht es zu einem wertvollen Werkzeug für Forscher, die die Biologie von Pilzen und Resistenzmechanismen untersuchen .
Wirkmechanismus
This compound entfaltet seine antimykotische Wirkung durch Hemmung der Dihydroorotat-Dehydrogenase, einem Enzym, das an der De-novo-Biosynthese von Pyrimidinen beteiligt ist. Diese Hemmung stört die Produktion von DNA und RNA in Pilzzellen, was zum Zelltod führt. Zu den molekularen Zielstrukturen von this compound gehören verschiedene Arten von Aspergillus, Scedosporium und Fusarium, unter anderem .
Vorbereitungsmethoden
Synthetic Routes and Reaction Conditions: The synthesis of Olorofim involves multiple steps, starting from commercially available starting materials. The key steps include the formation of the orotomide core structure, followed by functional group modifications to enhance antifungal activity. Specific reaction conditions, such as temperature, solvents, and catalysts, are optimized to achieve high yields and purity .
Industrial Production Methods: Industrial production of this compound involves large-scale synthesis using optimized synthetic routes. The process includes stringent quality control measures to ensure the consistency and efficacy of the final product. The production methods are designed to be cost-effective and scalable to meet the demands of clinical and commercial use .
Analyse Chemischer Reaktionen
Types of Reactions: Olorofim undergoes various chemical reactions, including oxidation, reduction, and substitution. These reactions are essential for modifying the compound’s structure to enhance its antifungal properties .
Common Reagents and Conditions: Common reagents used in the synthesis and modification of this compound include oxidizing agents, reducing agents, and nucleophiles. Reaction conditions such as temperature, pH, and solvent choice are carefully controlled to achieve the desired chemical transformations .
Major Products Formed: The major products formed from the chemical reactions of this compound include various derivatives with enhanced antifungal activity. These derivatives are tested for their efficacy against a range of fungal pathogens to identify the most potent compounds .
Wissenschaftliche Forschungsanwendungen
Olorofim has shown promising results in scientific research, particularly in the field of antifungal therapy. It is effective against a wide range of mold species, including those resistant to azoles and amphotericin B. This compound is being developed for both oral and intravenous administration, making it versatile for different clinical scenarios .
Its unique mechanism of action makes it a valuable tool for researchers studying fungal biology and resistance mechanisms .
Wirkmechanismus
Olorofim exerts its antifungal effects by inhibiting dihydroorotate dehydrogenase, an enzyme involved in the de novo biosynthesis of pyrimidines. This inhibition disrupts the production of DNA and RNA in fungal cells, leading to cell death. The molecular targets of this compound include various species of Aspergillus, Scedosporium, and Fusarium, among others .
Vergleich Mit ähnlichen Verbindungen
Ähnliche Verbindungen:
- Voriconazol
- Itraconazol
- Posaconazol
- Amphotericin B
Einzigartigkeit von Olorofim: this compound ist unter den Antimykotika aufgrund seines neuartigen Wirkmechanismus einzigartig. Im Gegensatz zu Azolen und Polyenen, die jeweils die Ergosterolsynthese und die Membranintegrität angreifen, zielt this compound auf die Pyrimidinbiosynthese ab. Dadurch ist es wirksam gegen Pilzarten, die eine Resistenz gegen andere Klassen von Antimykotika entwickelt haben .
Biologische Aktivität
Olorofim is a novel antifungal agent belonging to the orotomide class, primarily developed to combat invasive fungal infections, particularly those caused by resistant strains of Aspergillus and other filamentous fungi. Its mechanism of action involves the inhibition of dihydroorotate dehydrogenase (DHODH), which is crucial for pyrimidine biosynthesis, thereby impeding DNA synthesis, cell growth, and division in fungi.
In Vitro Activity
This compound has demonstrated potent in vitro activity against a variety of filamentous fungi, including both azole-susceptible and azole-resistant strains of Aspergillus fumigatus. The minimum inhibitory concentrations (MICs) for various fungal species have been extensively studied. Below is a summary of the MIC values reported for this compound against key fungal pathogens:
In Vivo Studies
In vivo studies have further corroborated the efficacy of this compound. Research has shown that treatment with this compound leads to significant reductions in fungal burden and serum galactomannan levels, a biomarker for invasive aspergillosis. Key findings include:
- Survival Rates : In murine models infected with azole-resistant A. fumigatus, treatment with this compound resulted in improved survival rates compared to control groups. For instance, a study indicated that mice treated with this compound had significantly lower galactomannan levels and higher survival rates than those treated with posaconazole .
- Histopathological Outcomes : Histological analysis revealed limited inflammatory responses and reduced fungal elements in organs from this compound-treated mice compared to placebo groups, indicating effective clearance of the pathogen .
Pharmacokinetics
The pharmacokinetic profile of this compound demonstrates its potential for effective systemic treatment:
- Bioavailability : Following oral administration, bioavailability ranges from 45% to 82% across different animal models .
- Protein Binding : this compound exhibits over 99% protein binding, suggesting substantial distribution within the body.
- Time-Dependent Activity : The pharmacokinetic/pharmacodynamic (PK/PD) relationship indicates that the Cmin/MIC ratio is critical for its antifungal efficacy, emphasizing the importance of maintaining adequate drug levels over time for optimal outcomes .
Clinical Case Studies
Recent clinical trials have highlighted the effectiveness of this compound in treating challenging fungal infections:
- In an open-label phase 2 study involving patients with extrapulmonary coccidioidomycosis, 75.6% demonstrated clinical benefit by day 42 post-treatment with this compound, underscoring its potential in real-world applications .
- A comprehensive review concluded that this compound is well-tolerated with manageable side effects, primarily infusion-related reactions and mild gastrointestinal disturbances .
Eigenschaften
CAS-Nummer |
1928707-56-5 |
---|---|
Molekularformel |
C28H27FN6O2 |
Molekulargewicht |
498.6 g/mol |
IUPAC-Name |
2-(1,5-dimethyl-3-phenylpyrrol-2-yl)-N-[4-[4-(5-fluoropyrimidin-2-yl)piperazin-1-yl]phenyl]-2-oxoacetamide |
InChI |
InChI=1S/C28H27FN6O2/c1-19-16-24(20-6-4-3-5-7-20)25(33(19)2)26(36)27(37)32-22-8-10-23(11-9-22)34-12-14-35(15-13-34)28-30-17-21(29)18-31-28/h3-11,16-18H,12-15H2,1-2H3,(H,32,37) |
InChI-Schlüssel |
SUFPWYYDCOKDLL-UHFFFAOYSA-N |
SMILES |
CC1=CC(=C(N1C)C(=O)C(=O)NC2=CC=C(C=C2)N3CCN(CC3)C4=NC=C(C=N4)F)C5=CC=CC=C5 |
Kanonische SMILES |
CC1=CC(=C(N1C)C(=O)C(=O)NC2=CC=C(C=C2)N3CCN(CC3)C4=NC=C(C=N4)F)C5=CC=CC=C5 |
Aussehen |
Solid powder |
Reinheit |
>98% (or refer to the Certificate of Analysis) |
Haltbarkeit |
>2 years if stored properly |
Löslichkeit |
Soluble in DMSO |
Lagerung |
Dry, dark and at 0 - 4 C for short term (days to weeks) or -20 C for long term (months to years). |
Synonyme |
F-901318; F901318; F 901318; Olorofim |
Herkunft des Produkts |
United States |
Retrosynthesis Analysis
AI-Powered Synthesis Planning: Our tool employs the Template_relevance Pistachio, Template_relevance Bkms_metabolic, Template_relevance Pistachio_ringbreaker, Template_relevance Reaxys, Template_relevance Reaxys_biocatalysis model, leveraging a vast database of chemical reactions to predict feasible synthetic routes.
One-Step Synthesis Focus: Specifically designed for one-step synthesis, it provides concise and direct routes for your target compounds, streamlining the synthesis process.
Accurate Predictions: Utilizing the extensive PISTACHIO, BKMS_METABOLIC, PISTACHIO_RINGBREAKER, REAXYS, REAXYS_BIOCATALYSIS database, our tool offers high-accuracy predictions, reflecting the latest in chemical research and data.
Strategy Settings
Precursor scoring | Relevance Heuristic |
---|---|
Min. plausibility | 0.01 |
Model | Template_relevance |
Template Set | Pistachio/Bkms_metabolic/Pistachio_ringbreaker/Reaxys/Reaxys_biocatalysis |
Top-N result to add to graph | 6 |
Feasible Synthetic Routes
Haftungsausschluss und Informationen zu In-Vitro-Forschungsprodukten
Bitte beachten Sie, dass alle Artikel und Produktinformationen, die auf BenchChem präsentiert werden, ausschließlich zu Informationszwecken bestimmt sind. Die auf BenchChem zum Kauf angebotenen Produkte sind speziell für In-vitro-Studien konzipiert, die außerhalb lebender Organismen durchgeführt werden. In-vitro-Studien, abgeleitet von dem lateinischen Begriff "in Glas", beinhalten Experimente, die in kontrollierten Laborumgebungen unter Verwendung von Zellen oder Geweben durchgeführt werden. Es ist wichtig zu beachten, dass diese Produkte nicht als Arzneimittel oder Medikamente eingestuft sind und keine Zulassung der FDA für die Vorbeugung, Behandlung oder Heilung von medizinischen Zuständen, Beschwerden oder Krankheiten erhalten haben. Wir müssen betonen, dass jede Form der körperlichen Einführung dieser Produkte in Menschen oder Tiere gesetzlich strikt untersagt ist. Es ist unerlässlich, sich an diese Richtlinien zu halten, um die Einhaltung rechtlicher und ethischer Standards in Forschung und Experiment zu gewährleisten.