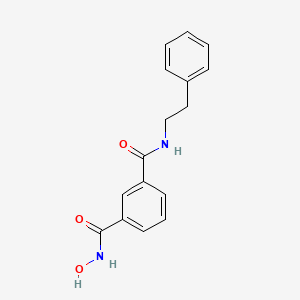
BRD73954
Übersicht
Beschreibung
BRD73954 ist ein potenter Inhibitor von Histon-Deacetylase-Enzymen, der speziell auf Histon-Deacetylase 6 und Histon-Deacetylase 8 abzielt. Histon-Deacetylase-Enzyme spielen eine entscheidende Rolle bei der Regulation der Genexpression, indem sie Acetylgruppen von Histonproteinen entfernen, was zu Chromatinverdichtung und transkriptioneller Repression führt. This compound hat aufgrund seiner Fähigkeit, diese Enzyme selektiv zu hemmen, ein großes Potenzial in der wissenschaftlichen Forschung gezeigt .
Herstellungsmethoden
This compound kann durch eine Reihe chemischer Reaktionen synthetisiert werden, die die Kondensation spezifischer Vorläufermoleküle beinhalten. Die Syntheseroute umfasst typischerweise die folgenden Schritte:
Bildung der Kernstruktur: Die Kernstruktur von this compound wird durch die Reaktion eines Benzolrings mit einem Carbonsäurederivat gebildet.
Einführung von funktionellen Gruppen: Verschiedene funktionelle Gruppen, wie Hydroxyl- und Phenethylgruppen, werden durch Substitutionsreaktionen in die Kernstruktur eingeführt.
Industrielle Produktionsmethoden für this compound sind nicht gut dokumentiert, da es hauptsächlich für Forschungszwecke verwendet wird. Die oben beschriebene Syntheseroute kann bei Bedarf für die Produktion in größerem Maßstab hochskaliert werden.
Chemische Reaktionsanalyse
This compound durchläuft verschiedene Arten chemischer Reaktionen, darunter:
Oxidation: this compound kann oxidiert werden, um verschiedene oxidierte Derivate zu bilden. Häufig verwendete Reagenzien in diesen Reaktionen sind Wasserstoffperoxid und Kaliumpermanganat.
Reduktion: Reduktionsreaktionen können this compound in reduzierte Derivate umwandeln. Natriumborhydrid und Lithiumaluminiumhydrid sind häufig verwendete Reduktionsmittel.
Substitution: Substitutionsreaktionen beinhalten die Substitution von funktionellen Gruppen in this compound durch andere Gruppen.
Die Hauptprodukte, die aus diesen Reaktionen gebildet werden, hängen von den spezifischen verwendeten Reagenzien und Bedingungen ab. So kann beispielsweise die Oxidation von this compound mit Wasserstoffperoxid zu hydroxylierten Derivaten führen, während die Reduktion mit Natriumborhydrid zu reduzierten Derivaten mit veränderten funktionellen Gruppen führen kann.
Wissenschaftliche Forschungsanwendungen
This compound hat eine breite Palette von wissenschaftlichen Forschungsanwendungen, darunter:
Chemie: this compound wird als Werkzeugverbindung verwendet, um die Rolle von Histon-Deacetylase-Enzymen in chemischen Reaktionen und der Genregulation zu untersuchen.
Biologie: In der biologischen Forschung wird this compound verwendet, um die Auswirkungen der Hemmung von Histon-Deacetylasen auf zelluläre Prozesse wie die Zellzyklusregulation, Apoptose und Differenzierung zu untersuchen.
Medizin: this compound hat potenzielle therapeutische Anwendungen bei der Behandlung von Krankheiten wie Krebs, neurodegenerativen Erkrankungen und entzündlichen Erkrankungen. Seine Fähigkeit, Histon-Deacetylase-Enzyme selektiv zu hemmen, macht es zu einem vielversprechenden Kandidaten für die Medikamentenentwicklung.
Industrie: Obwohl es hauptsächlich in der Forschung verwendet wird, kann this compound auch bei der Entwicklung neuer Materialien und chemischer Prozesse verwendet werden, die eine präzise Kontrolle der Genexpression erfordern
Wirkmechanismus
This compound übt seine Wirkung aus, indem es selektiv Histon-Deacetylase 6 und Histon-Deacetylase 8 hemmt. Die Hemmung dieser Enzyme führt zur Anhäufung von acetylierten Histonen, was zu einer Chromatinrelaxation und erhöhter Genexpression führt. Die molekularen Ziele von this compound umfassen die katalytischen Domänen von Histon-Deacetylase 6 und Histon-Deacetylase 8, die für die Deacetylierung von Histonproteinen verantwortlich sind. Durch die Bindung an diese Domänen verhindert this compound die Entfernung von Acetylgruppen von Histonen, wodurch die Genexpression und zelluläre Prozesse moduliert werden .
Wissenschaftliche Forschungsanwendungen
BRD73954 has a wide range of scientific research applications, including:
Chemistry: this compound is used as a tool compound to study the role of histone deacetylase enzymes in chemical reactions and gene regulation.
Biology: In biological research, this compound is used to investigate the effects of histone deacetylase inhibition on cellular processes such as cell cycle regulation, apoptosis, and differentiation.
Medicine: this compound has potential therapeutic applications in the treatment of diseases such as cancer, neurodegenerative disorders, and inflammatory diseases. Its ability to selectively inhibit histone deacetylase enzymes makes it a promising candidate for drug development.
Industry: Although primarily used in research, this compound can also be used in the development of new materials and chemical processes that require precise control of gene expression
Biochemische Analyse
Biochemical Properties
BRD73954 selectively inhibits HDAC6 and HDAC8 with IC50 values of 0.0036 µM and 0.12 µM respectively . It interacts with these enzymes, leading to the inhibition of their activity. The interaction between this compound and these enzymes is characterized by the binding of the compound to the active site of the enzymes, thereby preventing them from exerting their deacetylase activity .
Cellular Effects
In cellular processes, this compound has been shown to decrease the levels of HDAC6, which is associated with the upregulation of Ac-Tubulin . This suggests that this compound influences cell function by modulating the acetylation state of proteins, which can impact cell signaling pathways, gene expression, and cellular metabolism .
Molecular Mechanism
The molecular mechanism of this compound involves its binding to HDAC6 and HDAC8, inhibiting their activity and leading to an increase in acetylation of specific substrates such as α-tubulin . This change in acetylation status can lead to alterations in gene expression and other cellular processes .
Vorbereitungsmethoden
BRD73954 can be synthesized through a series of chemical reactions involving the condensation of specific precursor molecules. The synthetic route typically involves the following steps:
Formation of the core structure: The core structure of this compound is formed by the reaction of a benzene ring with a carboxylic acid derivative.
Introduction of functional groups: Various functional groups, such as hydroxyl and phenethyl groups, are introduced to the core structure through substitution reactions.
Purification: The final product is purified using techniques such as recrystallization and chromatography to achieve high purity.
Industrial production methods for this compound are not well-documented, as it is primarily used for research purposes. the synthetic route described above can be scaled up for larger-scale production if needed.
Analyse Chemischer Reaktionen
BRD73954 undergoes several types of chemical reactions, including:
Oxidation: this compound can be oxidized to form various oxidized derivatives. Common reagents used in these reactions include hydrogen peroxide and potassium permanganate.
Reduction: Reduction reactions can convert this compound into reduced derivatives. Sodium borohydride and lithium aluminum hydride are commonly used reducing agents.
Substitution: Substitution reactions involve the replacement of functional groups in this compound with other groups.
The major products formed from these reactions depend on the specific reagents and conditions used. For example, oxidation of this compound with hydrogen peroxide can yield hydroxylated derivatives, while reduction with sodium borohydride can produce reduced derivatives with altered functional groups.
Wirkmechanismus
BRD73954 exerts its effects by selectively inhibiting histone deacetylase 6 and histone deacetylase 8. The inhibition of these enzymes leads to the accumulation of acetylated histones, resulting in chromatin relaxation and increased gene expression. The molecular targets of this compound include the catalytic domains of histone deacetylase 6 and histone deacetylase 8, which are responsible for the deacetylation of histone proteins. By binding to these domains, this compound prevents the removal of acetyl groups from histones, thereby modulating gene expression and cellular processes .
Vergleich Mit ähnlichen Verbindungen
BRD73954 ist einzigartig in seiner Fähigkeit, sowohl Histon-Deacetylase 6 als auch Histon-Deacetylase 8 selektiv zu hemmen. Zu anderen ähnlichen Verbindungen gehören:
Trichostatin A: Ein breitspektriger Histon-Deacetylase-Inhibitor, der mehrere Histon-Deacetylase-Enzyme, einschließlich Histon-Deacetylase 6 und Histon-Deacetylase 8, angreift.
Vorinostat: Ein weiterer breitspektriger Histon-Deacetylase-Inhibitor mit Aktivität gegen mehrere Histon-Deacetylase-Enzyme.
Panobinostat: Ein potenter Inhibitor mehrerer Histon-Deacetylase-Enzyme, einschließlich Histon-Deacetylase 6 und Histon-Deacetylase 8
Die Selektivität von this compound für Histon-Deacetylase 6 und Histon-Deacetylase 8 unterscheidet es von diesen anderen Inhibitoren, was es zu einem wertvollen Werkzeug für die Untersuchung der spezifischen Rollen dieser Enzyme in verschiedenen biologischen Prozessen macht.
Eigenschaften
IUPAC Name |
3-N-hydroxy-1-N-(2-phenylethyl)benzene-1,3-dicarboxamide | |
---|---|---|
Source | PubChem | |
URL | https://pubchem.ncbi.nlm.nih.gov | |
Description | Data deposited in or computed by PubChem | |
InChI |
InChI=1S/C16H16N2O3/c19-15(17-10-9-12-5-2-1-3-6-12)13-7-4-8-14(11-13)16(20)18-21/h1-8,11,21H,9-10H2,(H,17,19)(H,18,20) | |
Source | PubChem | |
URL | https://pubchem.ncbi.nlm.nih.gov | |
Description | Data deposited in or computed by PubChem | |
InChI Key |
FIHKWEQJEDRIFS-UHFFFAOYSA-N | |
Source | PubChem | |
URL | https://pubchem.ncbi.nlm.nih.gov | |
Description | Data deposited in or computed by PubChem | |
Canonical SMILES |
C1=CC=C(C=C1)CCNC(=O)C2=CC(=CC=C2)C(=O)NO | |
Source | PubChem | |
URL | https://pubchem.ncbi.nlm.nih.gov | |
Description | Data deposited in or computed by PubChem | |
Molecular Formula |
C16H16N2O3 | |
Source | PubChem | |
URL | https://pubchem.ncbi.nlm.nih.gov | |
Description | Data deposited in or computed by PubChem | |
Molecular Weight |
284.31 g/mol | |
Source | PubChem | |
URL | https://pubchem.ncbi.nlm.nih.gov | |
Description | Data deposited in or computed by PubChem | |
Retrosynthesis Analysis
AI-Powered Synthesis Planning: Our tool employs the Template_relevance Pistachio, Template_relevance Bkms_metabolic, Template_relevance Pistachio_ringbreaker, Template_relevance Reaxys, Template_relevance Reaxys_biocatalysis model, leveraging a vast database of chemical reactions to predict feasible synthetic routes.
One-Step Synthesis Focus: Specifically designed for one-step synthesis, it provides concise and direct routes for your target compounds, streamlining the synthesis process.
Accurate Predictions: Utilizing the extensive PISTACHIO, BKMS_METABOLIC, PISTACHIO_RINGBREAKER, REAXYS, REAXYS_BIOCATALYSIS database, our tool offers high-accuracy predictions, reflecting the latest in chemical research and data.
Strategy Settings
Precursor scoring | Relevance Heuristic |
---|---|
Min. plausibility | 0.01 |
Model | Template_relevance |
Template Set | Pistachio/Bkms_metabolic/Pistachio_ringbreaker/Reaxys/Reaxys_biocatalysis |
Top-N result to add to graph | 6 |
Feasible Synthetic Routes
Q1: What makes BRD73954 unique compared to other HDAC inhibitors?
A1: this compound stands out as the first small molecule HDAC inhibitor capable of potently and selectively inhibiting both HDAC6 and HDAC8. [] This is significant because these two isoforms belong to different phylogenetic classes within the HDAC family. This dual-inhibitory activity makes this compound a valuable tool for studying the distinct and potentially synergistic roles of HDAC6 and HDAC8 in various biological processes.
Q2: How does the structure of this compound contribute to its dual HDAC6/8 inhibitory activity?
A2: The research highlights the importance of the meta substituent on the phenyl hydroxamic acid moiety of this compound. This meta substituent is deemed crucial for the potent inhibition of HDAC8, while also being well-accommodated within the binding site of HDAC6. [] This suggests that structural modifications in this region could be explored to fine-tune the selectivity and potency towards specific HDAC isoforms.
Haftungsausschluss und Informationen zu In-Vitro-Forschungsprodukten
Bitte beachten Sie, dass alle Artikel und Produktinformationen, die auf BenchChem präsentiert werden, ausschließlich zu Informationszwecken bestimmt sind. Die auf BenchChem zum Kauf angebotenen Produkte sind speziell für In-vitro-Studien konzipiert, die außerhalb lebender Organismen durchgeführt werden. In-vitro-Studien, abgeleitet von dem lateinischen Begriff "in Glas", beinhalten Experimente, die in kontrollierten Laborumgebungen unter Verwendung von Zellen oder Geweben durchgeführt werden. Es ist wichtig zu beachten, dass diese Produkte nicht als Arzneimittel oder Medikamente eingestuft sind und keine Zulassung der FDA für die Vorbeugung, Behandlung oder Heilung von medizinischen Zuständen, Beschwerden oder Krankheiten erhalten haben. Wir müssen betonen, dass jede Form der körperlichen Einführung dieser Produkte in Menschen oder Tiere gesetzlich strikt untersagt ist. Es ist unerlässlich, sich an diese Richtlinien zu halten, um die Einhaltung rechtlicher und ethischer Standards in Forschung und Experiment zu gewährleisten.