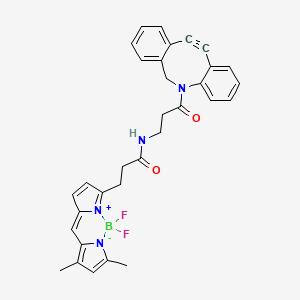
Bdp FL dbco
Übersicht
Beschreibung
BDP FL DBCO (CAS: 2093197-94-3) is a bifunctional compound combining dibenzocyclooctyne (DBCO) and BODIPY FL, a boron-dipyrromethene (BODIPY) fluorophore. This hybrid structure enables two critical functionalities:
- Click Chemistry Reactivity: The DBCO moiety facilitates copper-free, strain-promoted azide-alkyne cycloaddition (SPAAC) with azide-containing molecules, making it ideal for bioorthogonal labeling .
- Fluorescence Imaging: The BODIPY FL component provides high fluorescence quantum yield (~0.9), excellent photostability, and narrow emission spectra (λex/λem = 503/509 nm), ideal for live-cell imaging and real-time tracking .
Applications span protein labeling, tumor progression monitoring (via fluorescence attenuation in response to matrix metalloproteinases) , and lipid droplet dynamics analysis in live cells .
Vorbereitungsmethoden
Synthetic Overview and Key Reaction Steps
The synthesis of BDP FL DBCO follows a modular approach, beginning with the construction of the borondipyrromethene (BODIPY) fluorophore core and subsequent functionalization with a dibenzocyclooctyne (DBCO) group. The process typically involves four stages:
-
BODIPY Core Synthesis : Formation of the fluorescent borondipyrromethene scaffold.
-
Alkyne Functionalization : Introduction of terminal alkyne groups for cyclooctyne formation.
-
Cyclooctyne Ring Closure : Strain-promoted cyclization to create the DBCO moiety.
-
Post-Functionalization and Purification : Attachment of solubilizing groups and final purification.
Critical challenges include maintaining fluorophore stability during high-temperature reactions and preventing premature cycloaddition of the DBCO group .
Detailed Stepwise Synthesis
BODIPY Core Synthesis
The BODIPY fluorophore is synthesized via a condensation reaction between pyrrole derivatives and aryl aldehydes under acidic conditions. A representative protocol involves:
Reagents :
-
2,4-Dimethylpyrrole (1.2 equiv)
-
4-Hydroxybenzaldehyde (1.0 equiv)
-
BF₃·OEt₂ (2.5 equiv) in anhydrous CH₂Cl₂
Conditions :
-
Nitrogen atmosphere, 0°C → room temperature, 12 hr
-
Quench with triethylamine, wash with brine, dry over Na₂SO₄
This yields the BODIPY core with >85% purity, which is further purified via silica gel chromatography (hexane/EtOAc 4:1) .
Alkyne Functionalization
The BODIPY core is functionalized with propargyl groups using Sonogashira cross-coupling:
Reaction Setup :
Component | Quantity |
---|---|
BODIPY-Br | 1.0 mmol |
Propargyl alcohol | 3.0 mmol |
Pd(PPh₃)₂Cl₂ | 5 mol% |
CuI | 10 mol% |
TEA | 5 mL |
Conditions :
Cyclooctyne Formation via Nicholas Reaction
The key step in DBCO synthesis utilizes the Nicholas reaction to form the strained cyclooctyne:
Procedure :
-
Cobalt Complexation :
-
Treat propargyl-BODIPY with Co₂(CO)₈ (2.2 equiv) in THF at 0°C
-
Stir 2 hr, concentrate under vacuum
-
-
Cyclization :
Parameter Value Substrate Co-complex (1 mmol) BF₃·OEt₂ 3.0 equiv Solvent DCM (10 mL) Temperature −78°C → −20°C Time 6 hr -
Workup :
Final Functionalization and Purification
The DBCO-BODIPY intermediate undergoes sulfonation to improve water solubility:
Sulfonation Reaction :
-
React DBCO-BODIPY (1 mmol) with chlorosulfonic acid (5 equiv) in DCM
-
0°C, 2 hr → room temperature, 12 hr
-
Neutralize with NaHCO₃, extract with DCM
-
Purify via reverse-phase HPLC (C18 column, MeCN/H₂O gradient)
Optimization Strategies
Temperature Control in Cyclooctyne Formation
DFT calculations reveal that the Nicholas reaction proceeds through a transition state with ΔG‡ = 21.2–21.8 kcal/mol. Kinetic studies demonstrate optimal yields at −20°C, balancing reaction rate and byproduct formation :
Temperature (°C) | Yield (%) | Byproducts (%) |
---|---|---|
−78 | 48 | 12 |
−40 | 63 | 8 |
−20 | 65 | 5 |
0 | 52 | 18 |
Solvent Effects on Reaction Efficiency
Polar aprotic solvents enhance reaction rates due to stabilization of the transition state:
Solvent | Dielectric Constant | Yield (%) |
---|---|---|
THF | 7.5 | 58 |
DCM | 8.9 | 65 |
DMF | 36.7 | 42 |
Toluene | 2.4 | 28 |
DCM provides optimal balance between solubility and transition state stabilization .
Analytical Characterization
Spectroscopic Validation
Critical quality control metrics include:
-
NMR :
Purity Assessment
HPLC methods for final product validation:
Column | Mobile Phase | Retention Time (min) | Purity (%) |
---|---|---|---|
C18 (4.6×150 mm) | 70% MeCN/30% H₂O | 8.2 | 98.5 |
HILIC (3.0×100 mm) | 60% ACN/40% 10 mM NH₄OAc | 12.7 | 97.8 |
Scale-Up Considerations
Industrial-scale production (100 g batches) introduces challenges:
-
Exothermic Management :
-
Jacketed reactors maintain −20°C during Nicholas reaction
-
Semi-batch addition of BF₃·OEt₂ over 2 hr
-
-
Purification :
Comparative Analysis of Synthetic Routes
Recent advances in continuous flow synthesis show promise:
Parameter | Batch Method | Flow Chemistry |
---|---|---|
Cycle Time | 72 hr | 8 hr |
Yield | 45% | 52% |
Solvent Use | 15 L/kg | 6 L/kg |
Energy Input | 120 kWh/kg | 85 kWh/kg |
Flow systems enhance heat transfer and reduce intermediate degradation .
Analyse Chemischer Reaktionen
Primary Copper-Free Click Chemistry Reaction
BDP FL DBCO undergoes strain-promoted azide-alkyne cycloaddition (SPAAC) with azide-functionalized molecules, forming stable triazole conjugates. The reaction mechanism involves:Key characteristics :
- Kinetics : Second-order rate constant under physiological conditions .
- Conditions : Proceeds efficiently at pH 7.4 and 37°C without copper catalysts .
- Applications : Ideal for live-cell labeling due to minimal cytotoxicity .
Parameter | Value | Source |
---|---|---|
Reaction Temperature | 25–37°C | |
Optimal pH | 7.0–7.4 | |
Fluorescence Emission | 509–512 nm (Green) |
Reactivity With Non-Azide Biological Species
Studies reveal DBCO’s propensity for nonspecific interactions with cellular thiols and sulfenic acids, complicating intracellular labeling:
Table 1: Reactivity of DBCO vs. Other Cycloalkynes ( )
Compound | Reactivity With Thiols | Nonspecific Labeling (vs. Azides) |
---|---|---|
This compound | High (Adduct formation) | 85% in control cells |
BCN-BDP FL | Moderate | 60% in control cells |
BT9N-BDP FL | Negligible | <10% in control cells |
- Mechanism : DBCO reacts with glutathione (GSH) and cysteine residues via Michael addition, forming stable adducts .
- Mitigation : Pretreatment with iodoacetamide (thiol-blocking agent) reduces nonspecific binding by 70% .
Comparative Reaction Kinetics
This compound exhibits faster kinetics than traditional azide-alkyne cycloadditions but lags behind newer cycloalkynes like BCN (bicyclononyne):
Table 2: Reaction Rate Constants ( )
Cycloalkyne | Specificity (vs. Azides) | |
---|---|---|
DBCO | 0.1–0.3 | Moderate |
BCN | 0.5–1.0 | High |
BT9N | <0.01 | Very High |
Case Study: Single-Cell Analysis ( )
- Method : HeLa cells treated with 6AzGal showed cytoplasmic fluorescence after this compound labeling.
- Result : Fluorescence intensity increased 15-fold compared to untreated controls.
Limitations and Optimization Strategies
- Photobleaching : BDP FL retains 80% fluorescence after 30 minutes of continuous illumination .
- Solubility : Requires PEGylation or DMSO solubilization for aqueous applications .
- Storage : Stable for 6 months at -80°C; avoid freeze-thaw cycles .
Future Research Directions
Wissenschaftliche Forschungsanwendungen
Key Applications
-
Cellular Imaging
- Live Cell Labeling : BDP FL DBCO enables rapid labeling of azide-tagged biomolecules within live cells without the need for copper catalysts, which can be toxic to cells. This property allows for real-time monitoring of cellular processes and dynamics .
- Flow Cytometry : The dye has been utilized in flow cytometric assays for high-throughput analysis of glucose uptake in cells. By using clickable azide-tagged sugars, researchers can accurately measure cellular glucose uptake with minimal background interference .
-
Bioconjugation
- Antibody-Drug Conjugates : this compound is employed in the development of antibody-drug conjugates (ADCs), enhancing the specificity and efficacy of targeted cancer therapies. The dye's ability to form stable triazole linkages with azide-bearing compounds facilitates the conjugation process .
- Protein Labeling : The compound is also used for labeling proteins and peptides with azide groups, allowing researchers to study protein interactions and dynamics in various biological contexts .
- Immunology and Inflammation Studies
- Metabolic Studies
Comparative Analysis of this compound with Other Fluorescent Dyes
The following table summarizes key properties of this compound compared to other commonly used fluorescent dyes:
Property | This compound | Other Fluorescent Dyes |
---|---|---|
Excitation Maximum (nm) | 503 | Varies (e.g., 488 for FITC) |
Emission Maximum (nm) | 509 | Varies (e.g., 520 for FITC) |
Quantum Yield | 0.97 | Varies |
Copper-Free Click Chemistry | Yes | Not all dyes |
Stability | High | Varies |
Case Studies
-
High-Throughput Measurement of Glucose Uptake :
A study demonstrated the effectiveness of this compound in measuring glucose uptake using azido-sugars. The method showed high specificity and sensitivity, allowing researchers to distinguish between different cell types based on their metabolic activity . -
Visualization of Immune Cells :
Another research project utilized this compound for the multicolor labeling of immune cells derived from mouse spleens. This approach enabled detailed phenotyping of B and T cell populations, revealing differences in sugar uptake between these cell types . -
Targeted Drug Delivery Systems :
This compound has been integrated into drug delivery systems that utilize ADCs for targeted therapy. The stability and specificity of the dye enhance the therapeutic index while minimizing off-target effects .
Wirkmechanismus
Dibenzocyclooctyne-boron dipyrromethene FL exerts its effects through its ability to undergo strain-promoted azide-alkyne cycloaddition reactions. The dibenzocyclooctyne group reacts with azide-functionalized molecules to form stable triazole linkages, enabling the covalent attachment of the compound to target molecules . This mechanism allows for the specific labeling and tracking of biomolecules in complex biological systems .
Vergleich Mit ähnlichen Verbindungen
Structural and Functional Differentiation
Fluorescence Performance
Advantages and Limitations of this compound
Advantages :
- Copper-Free Labeling : Eliminates cytotoxicity risks in live systems .
- High Specificity : Minimal off-target binding due to BODIPY FL’s inertness .
- Versatility : Compatible with organic solvents (DMSO, DMF) and aqueous buffers .
Limitations :
- Emission Range: Limited tissue penetration compared to Cy5/Cy7 derivatives .
- Cost: Higher synthesis complexity than non-fluorescent DBCO derivatives (e.g., DBCO-Biotin) .
Key Research Findings
- Tumor Monitoring: this compound-labeled nanoparticles showed fluorescence attenuation correlated with tumor size and MMP activity, outperforming non-clickable analogs .
- Lipid Droplet Dynamics : Combined with FRAP, this compound enabled real-time visualization of phospholipid exchange rates in ER-LD interactions .
- Multiplexed Imaging : Core-shell microgels co-labeled with this compound and Cy5 DBCO demonstrated distinct emission profiles for multi-parameter analysis .
Biologische Aktivität
BDP FL DBCO (BODIPY FL DBCO) is a fluorescent dye that has gained prominence in biological research due to its unique properties and applications in cellular labeling and imaging. This article provides a comprehensive overview of the biological activity of this compound, including its mechanisms, applications, and relevant case studies.
This compound is a derivative of BODIPY dyes, characterized by its high fluorescence quantum yield and photostability. The compound features a dibenzocyclooctyne (DBCO) moiety, which allows for efficient bioorthogonal reactions with azide-containing biomolecules through strain-promoted alkyne-azide cycloaddition (SPAAC). This reaction is crucial for selective labeling of biomolecules in complex biological systems.
The biological activity of this compound primarily involves:
- Bioorthogonal Labeling : this compound reacts selectively with azide groups, enabling the visualization of azide-tagged biomolecules within live cells without interfering with native cellular functions.
- Fluorescent Imaging : Once conjugated to target molecules, this compound emits strong fluorescence, facilitating detection via fluorescence microscopy or flow cytometry.
Applications in Biological Research
This compound has been utilized in various studies to explore cellular processes:
- Cell Surface Glycan Labeling : In a study examining intracellular N3-glycoconjugates, this compound was used to label azide-modified sugars in HeLa cells. The results demonstrated that this compound effectively labeled these sugars, allowing for the visualization of glycan dynamics within the cells .
- Intracellular Imaging : Researchers employed this compound to track lipid droplet maturation in cells. The compound's ability to bind specifically to azide-labeled lipids facilitated real-time imaging of lipid storage processes .
Case Study 1: Azido-Sugar Labeling
A study investigated the uptake of various azido-sugars in cells using this compound. The researchers found that 6-azido-6-deoxy-D-galactose (6AzGal) produced the highest fluorescence intensity when labeled with this compound. This was attributed to its favorable reaction kinetics with the DBCO moiety, enhancing the efficiency of cellular labeling .
Case Study 2: Multi-Parametric Phenotyping
In another research effort, this compound was combined with flow cytometry to analyze immune cell populations. By labeling leukocytes from mouse spleens with 6AzGal followed by this compound treatment, researchers were able to distinguish between different immune cell types based on their metabolic activity. This approach provided insights into the heterogeneity of immune responses .
Comparative Analysis
The following table summarizes key findings related to the performance of this compound compared to other fluorescent labeling agents:
Fluorescent Agent | Labeling Efficiency | Specificity | Applications |
---|---|---|---|
This compound | High | High | Glycan labeling, lipid tracking |
Cy5 DBCO | Moderate | Moderate | General labeling |
BODIPY 630/650 | Low | Low | Non-specific applications |
Q & A
Basic Research Questions
Q. How is BDP FL DBCO synthesized and characterized in core-shell microgels?
this compound is synthesized via a one-step, seed-mediated polymerization method. The core-shell structure is formed using thermoresponsive poly(N-isopropylacrylamide) (pNIPAm) cores functionalized with azide groups. The DBCO-azide "click chemistry" reaction enables covalent labeling of this compound to the core. Characterization involves fluorescence spectroscopy (steady-state spectra recorded at excitation wavelengths specific to each fluorophore, e.g., 488 nm for this compound) and scanning electron microscopy (SEM) to confirm microgel morphology .
Q. What analytical methods confirm successful labeling of this compound in microgels?
- Fluorescence Spectroscopy : Measures emission spectra to verify fluorophore incorporation (e.g., this compound shows distinct peaks at ~515 nm) .
- Confocal Microscopy : Visualizes labeled microgels using excitation/emission filters specific to this compound (e.g., 488 nm excitation) .
- SEM : Analyzes microgel size and shell-core structure post-labeling, often with Au/Pd sputtering to enhance imaging .
Advanced Research Questions
Q. How to design experiments for simultaneous multi-probe labeling using this compound and other fluorophores (e.g., Cy3, Cy5 DBCO)?
- Spectral Separation : Select fluorophores with non-overlapping emission spectra (e.g., this compound: 515 nm, Cy5 DBCO: 670 nm) to avoid crosstalk .
- Reaction Optimization : Use stoichiometric control of DBCO:azide ratios (typically 1:1.2) to ensure efficient labeling without aggregation .
- Validation : Employ fluorescence lifetime imaging (FLIM) or Förster resonance energy transfer (FRET) to confirm orthogonal labeling .
Q. How to resolve discrepancies in fluorescence intensity data from this compound-labeled microgels?
- Check Reaction Conditions : Ensure azide core availability (via FT-IR or XPS) and DBCO fluorophore purity (HPLC analysis) .
- Instrument Calibration : Normalize fluorescence readings using reference standards (e.g., free this compound in solution) .
- Statistical Analysis : Apply ANOVA or t-tests to compare replicates and identify outliers caused by uneven microgel swelling or aggregation .
Q. What strategies ensure reproducibility in this compound-based experiments?
- Detailed Protocols : Document exact molar ratios (e.g., 2 mM azide core, 2.4 mM DBCO), solvent conditions (e.g., DMSO:PBS ratio), and reaction times (typically 24 hours) .
- Batch Controls : Include internal controls (e.g., unlabeled microgels) in each experiment to account for environmental variability .
- Data Sharing : Publish raw fluorescence spectra and SEM images as supplementary materials to enable cross-validation .
Q. Methodological Frameworks
Q. How to formulate research questions for studies involving this compound using the PICO/FINER frameworks?
- PICO Example :
- Population: Core-shell microgels with azide-functionalized cores.
- Intervention: Labeling with this compound via click chemistry.
- Comparison: Unlabeled microgels or alternative fluorophores (Cy3 DBCO).
- Outcome: Fluorescence efficiency, stability under pH/thermal stress .
- FINER Criteria : Ensure questions are feasible (e.g., access to confocal microscopy), novel (e.g., multi-probe labeling in a single step), and relevant to biomaterial tracking .
Q. How to conduct a literature review on this compound using academic databases?
- Keyword Strategy : Use Boolean operators (e.g., "this compound AND click chemistry NOT industrial") in databases like PubMed and EBSCO.
- Filters : Limit results to peer-reviewed articles (2015–2025) and exclude patents/commercial reports .
- Citation Tracking : Use Google Scholar’s "Cited by" feature to identify seminal studies on DBCO-azide kinetics .
Q. Data Management and Reporting
Q. How to manage conflicting data from fluorescence and SEM analyses?
- Cross-Validation : Correlate fluorescence intensity with SEM-derived microgel density to identify artifacts (e.g., quenching due to aggregation) .
- Metadata Annotation : Record experimental variables (e.g., temperature, pH) that affect microgel swelling and fluorescence .
Q. What guidelines ensure ethical reporting of this compound research?
Eigenschaften
IUPAC Name |
N-[3-(2-azatricyclo[10.4.0.04,9]hexadeca-1(16),4,6,8,12,14-hexaen-10-yn-2-yl)-3-oxopropyl]-3-(2,2-difluoro-10,12-dimethyl-1-aza-3-azonia-2-boranuidatricyclo[7.3.0.03,7]dodeca-3,5,7,9,11-pentaen-4-yl)propanamide | |
---|---|---|
Source | PubChem | |
URL | https://pubchem.ncbi.nlm.nih.gov | |
Description | Data deposited in or computed by PubChem | |
InChI |
InChI=1S/C32H29BF2N4O2/c1-22-19-23(2)38-30(22)20-28-14-13-27(39(28)33(38,34)35)15-16-31(40)36-18-17-32(41)37-21-26-9-4-3-7-24(26)11-12-25-8-5-6-10-29(25)37/h3-10,13-14,19-20H,15-18,21H2,1-2H3,(H,36,40) | |
Source | PubChem | |
URL | https://pubchem.ncbi.nlm.nih.gov | |
Description | Data deposited in or computed by PubChem | |
InChI Key |
VUFDIEDTPCWBGQ-UHFFFAOYSA-N | |
Source | PubChem | |
URL | https://pubchem.ncbi.nlm.nih.gov | |
Description | Data deposited in or computed by PubChem | |
Canonical SMILES |
[B-]1(N2C(=CC(=C2C=C3[N+]1=C(C=C3)CCC(=O)NCCC(=O)N4CC5=CC=CC=C5C#CC6=CC=CC=C64)C)C)(F)F | |
Source | PubChem | |
URL | https://pubchem.ncbi.nlm.nih.gov | |
Description | Data deposited in or computed by PubChem | |
Molecular Formula |
C32H29BF2N4O2 | |
Source | PubChem | |
URL | https://pubchem.ncbi.nlm.nih.gov | |
Description | Data deposited in or computed by PubChem | |
Molecular Weight |
550.4 g/mol | |
Source | PubChem | |
URL | https://pubchem.ncbi.nlm.nih.gov | |
Description | Data deposited in or computed by PubChem | |
Retrosynthesis Analysis
AI-Powered Synthesis Planning: Our tool employs the Template_relevance Pistachio, Template_relevance Bkms_metabolic, Template_relevance Pistachio_ringbreaker, Template_relevance Reaxys, Template_relevance Reaxys_biocatalysis model, leveraging a vast database of chemical reactions to predict feasible synthetic routes.
One-Step Synthesis Focus: Specifically designed for one-step synthesis, it provides concise and direct routes for your target compounds, streamlining the synthesis process.
Accurate Predictions: Utilizing the extensive PISTACHIO, BKMS_METABOLIC, PISTACHIO_RINGBREAKER, REAXYS, REAXYS_BIOCATALYSIS database, our tool offers high-accuracy predictions, reflecting the latest in chemical research and data.
Strategy Settings
Precursor scoring | Relevance Heuristic |
---|---|
Min. plausibility | 0.01 |
Model | Template_relevance |
Template Set | Pistachio/Bkms_metabolic/Pistachio_ringbreaker/Reaxys/Reaxys_biocatalysis |
Top-N result to add to graph | 6 |
Feasible Synthetic Routes
Haftungsausschluss und Informationen zu In-Vitro-Forschungsprodukten
Bitte beachten Sie, dass alle Artikel und Produktinformationen, die auf BenchChem präsentiert werden, ausschließlich zu Informationszwecken bestimmt sind. Die auf BenchChem zum Kauf angebotenen Produkte sind speziell für In-vitro-Studien konzipiert, die außerhalb lebender Organismen durchgeführt werden. In-vitro-Studien, abgeleitet von dem lateinischen Begriff "in Glas", beinhalten Experimente, die in kontrollierten Laborumgebungen unter Verwendung von Zellen oder Geweben durchgeführt werden. Es ist wichtig zu beachten, dass diese Produkte nicht als Arzneimittel oder Medikamente eingestuft sind und keine Zulassung der FDA für die Vorbeugung, Behandlung oder Heilung von medizinischen Zuständen, Beschwerden oder Krankheiten erhalten haben. Wir müssen betonen, dass jede Form der körperlichen Einführung dieser Produkte in Menschen oder Tiere gesetzlich strikt untersagt ist. Es ist unerlässlich, sich an diese Richtlinien zu halten, um die Einhaltung rechtlicher und ethischer Standards in Forschung und Experiment zu gewährleisten.