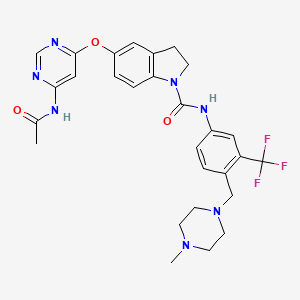
NVP-BBT594
Übersicht
Beschreibung
NVP-BBT594 ist ein potenter Inhibitor des Rezeptortyrosinkinase RET (REarranged during Transfection), der durch seinen Liganden, den glialen Neurotrophen Faktor, aktiviert wird. Die RET-Signalübertragung ist an verschiedenen Entwicklungsprozessen beteiligt, darunter Proliferation, Migration, Überleben und Differenzierung. This compound wird hauptsächlich in der Krebsforschung eingesetzt, da es die RET-Signalübertragung effektiv hemmen kann .
Wissenschaftliche Forschungsanwendungen
NVP-BBT594 hat verschiedene Anwendungen in der wissenschaftlichen Forschung, darunter:
Chemie: Als Werkzeugverbindung zur Untersuchung von RET-Signalwegen und deren Rolle in zellulären Prozessen.
Biologie: In der Forschung eingesetzt, um die Rolle von RET in Entwicklung und Krankheit zu verstehen.
Medizin: Auf seine potenziellen therapeutischen Anwendungen bei der Behandlung von Krebsarten untersucht, die eine abnorme RET-Signalübertragung aufweisen.
Industrie: Zur Entwicklung neuer Medikamente eingesetzt, die auf RET und verwandte Wege abzielen
Wirkmechanismus
This compound übt seine Wirkung aus, indem es die inaktive Konformation des Janus-Kinase-2 (JAK2)-Enzyms stabilisiert, was zu einer nicht phosphorylierten Aktivierungsschleife und einer stärkeren Hemmung führt. Dieser Mechanismus beinhaltet die Bindung an die ATP-Bindungstasche von JAK2, wodurch dessen Aktivierung und die anschließende Signalübertragung verhindert wird. Die Verbindung hemmt auch die RET-Signalübertragung, indem sie die Interaktion zwischen RET und seinem Liganden, dem glialen Neurotrophen Faktor, blockiert .
Wirkmechanismus
Target of Action
Nvp-bbt594, also known as BBT594, is a potent inhibitor of the receptor tyrosine kinase RET . RET is a crucial player in several cellular processes, including cell survival, proliferation, and differentiation . In addition to RET, this compound also targets Janus Kinase 2 (JAK2), a key player in cytokine receptor signaling .
Mode of Action
This compound acts as a type-II inhibitor, stabilizing the inactive conformation of its targets . For JAK2, this results in an unphosphorylated activation loop, likely achieving a stronger inhibition . The compound’s interaction with its targets leads to a blockade of their function, despite an increase in activation loop phosphorylation .
Biochemical Pathways
The primary biochemical pathway affected by this compound is the JAK-STAT signaling pathway . This pathway is crucial for transmitting information from chemical signals outside the cell, through the cell membrane, and into gene promoters on the DNA in the cell nucleus, causing DNA transcription and activity in the cell. By inhibiting JAK2, this compound disrupts this pathway, leading to a decrease in the proliferation of certain cell types .
Result of Action
This compound has been shown to block the enhancement of cell viability in certain cancer cell lines . It impairs GDNF-mediated RET downstream signaling and significantly enhances the antiproliferative effects of letrozole . This suggests that this compound could potentially be used in cancer treatment, particularly in cancers where the RET and JAK2 pathways play a significant role.
Action Environment
The action of this compound can be influenced by various environmental factors. For instance, the presence of certain hormones, such as estrogen, can affect the compound’s efficacy . Furthermore, the compound’s stability and action could potentially be affected by factors such as pH, temperature, and the presence of other substances in the body.
Biochemische Analyse
Biochemical Properties
Nvp-bbt594 plays a significant role in biochemical reactions. It interacts with enzymes and proteins, particularly the receptor tyrosine kinase RET . The nature of these interactions involves the suppression of GDNF-induced RET signaling, as assessed by RET, ERK1/2, AKT, and ER phosphorylation .
Cellular Effects
This compound has profound effects on various types of cells and cellular processes. It influences cell function by blocking the GDNF-mediated enhancement of MCF7-LTED cell viability in 2D culture and 3D colony formation . It also remarkably elevates the antiproliferative effects of letrozole in MCF7-2A cells .
Molecular Mechanism
The mechanism of action of this compound is quite complex. It exerts its effects at the molecular level by stabilizing the inactive conformation of JAK2, which results in an unphosphorylated activation loop, likely achieving a stronger inhibition . This binding interaction with biomolecules leads to changes in gene expression and enzyme inhibition or activation .
Temporal Effects in Laboratory Settings
Over time in laboratory settings, this compound continues to block the GDNF-mediated enhancement of MCF7-LTED cell viability in 2D culture and 3D colony formation
Vorbereitungsmethoden
Synthesewege und Reaktionsbedingungen
Die Synthese von NVP-BBT594 umfasst mehrere Schritte, darunter die Bildung von Schlüsselzwischenprodukten und deren anschließende Reaktionen unter spezifischen Bedingungen. Der detaillierte Syntheseweg und die Reaktionsbedingungen sind urheberrechtlich geschützt und werden nicht öffentlich bekannt gegeben.
Industrielle Produktionsmethoden
Die industrielle Produktion von this compound folgt ähnlichen Synthesewegen, jedoch in größerem Maßstab. Der Prozess beinhaltet die Optimierung der Reaktionsbedingungen, um eine hohe Ausbeute und Reinheit zu gewährleisten. Die Verbindung wird typischerweise in fester Form hergestellt und kann zur weiteren Verwendung in Lösungsmitteln wie Dimethylsulfoxid gelöst werden .
Analyse Chemischer Reaktionen
Arten von Reaktionen
NVP-BBT594 unterliegt verschiedenen chemischen Reaktionen, darunter:
Oxidation: Die Verbindung kann unter bestimmten Bedingungen oxidiert werden, um oxidierte Derivate zu bilden.
Reduktion: Reduktionsreaktionen können this compound in reduzierte Formen mit unterschiedlichen chemischen Eigenschaften umwandeln.
Substitution: Die Verbindung kann Substitutionsreaktionen eingehen, bei denen funktionelle Gruppen durch andere Gruppen ersetzt werden.
Häufige Reagenzien und Bedingungen
Oxidation: Häufige Oxidationsmittel sind Wasserstoffperoxid und Kaliumpermanganat.
Reduktion: Reduktionsmittel wie Natriumborhydrid und Lithiumaluminiumhydrid werden verwendet.
Substitution: Verschiedene Nukleophile und Elektrophile können unter kontrollierten Bedingungen verwendet werden, um Substitutionsreaktionen zu erreichen.
Hauptprodukte
Die Hauptprodukte, die aus diesen Reaktionen gebildet werden, hängen von den verwendeten Reagenzien und Bedingungen ab. Beispielsweise kann Oxidation zu oxidierten Derivaten mit veränderter biologischer Aktivität führen, während Reduktion Verbindungen mit unterschiedlichen pharmakologischen Eigenschaften erzeugen kann .
Vergleich Mit ähnlichen Verbindungen
Ähnliche Verbindungen
NVP-CHZ868: Ein weiterer JAK2-Inhibitor vom Typ II mit ähnlicher Bindungsart und Hemmwirkung.
Sunitinib: Ein Multi-Target-Rezeptortyrosinkinase-Inhibitor mit Aktivität gegen RET.
Cabozantinib: Ein Inhibitor mehrerer Rezeptortyrosinkinasen, einschließlich RET.
Einzigartigkeit
NVP-BBT594 ist aufgrund seiner hohen Spezifität und Potenz bei der Hemmung der RET-Signalübertragung einzigartig. Im Vergleich zu anderen ähnlichen Verbindungen zeigt es eine stärkere Hemmung von RET und verwandten Wegen, was es zu einem wertvollen Werkzeug in der Krebsforschung und Medikamentenentwicklung macht .
Biologische Aktivität
BBT594, also known as NVP-BBT594, is a type II kinase inhibitor primarily developed to target the T315I mutant of BCR-ABL. Its biological activity extends beyond BCR-ABL inhibition to include significant effects on the Janus kinase 2 (JAK2) signaling pathway, which is crucial in various hematological malignancies. This article details the biological activity of BBT594, focusing on its mechanisms of action, efficacy in preclinical studies, and potential clinical applications.
BBT594 functions by binding to the inactive conformation of JAK2, a mechanism that stabilizes the kinase and prevents its activation. This binding occurs at an allosteric site adjacent to the ATP-binding pocket, leading to reduced phosphorylation of key residues in JAK2 and downstream signaling molecules such as STAT5. The inhibition of JAK2 activity is particularly relevant in conditions where JAK2 mutations drive malignancies, such as acute lymphoblastic leukemia (ALL) and myeloproliferative neoplasms (MPNs).
In Vitro Studies
BBT594 has demonstrated potent anti-proliferative effects in various cell lines harboring JAK2 mutations. For instance, in studies involving Ph-like B-ALL cell lines such as MHH-CALL-4 and MUTZ-5, BBT594 exhibited an IC50 value of approximately 0.1 μM, indicating strong potency against these cells. The combination of BBT594 with other inhibitors like AZD2014 showed enhanced efficacy, achieving synergistic effects characterized by Combination Indices (CIs) less than 1.0 across multiple assays (Table 1) .
Table 1: Combination Index (CI) Values for BBT594 with AZD2014
Cell Line | CI Value (BBT594 + AZD2014) |
---|---|
MHH-CALL-4 | 0.5 ± 0.02 |
MUTZ-5 | 0.7 ± 0.11 |
REH | Not significant |
In Vivo Studies
In vivo experiments using patient-derived xenograft (PDX) models have further validated the efficacy of BBT594. Treatment with BBT594 alone or in combination with AZD2014 resulted in significant reductions in spleen size and bone marrow infiltration by leukemic cells . These findings suggest that BBT594 not only inhibits tumor cell proliferation but also impacts tumor microenvironment dynamics.
Resistance Mechanisms
Despite its efficacy, resistance to BBT594 can develop, particularly through mutations in the JAK2 gene itself. For example, the L884P mutation has been shown to reduce the binding affinity of BBT594 to JAK2, leading to diminished therapeutic effects . Understanding these resistance mechanisms is crucial for developing strategies to overcome them and enhance treatment outcomes.
Clinical Implications
The promising results from preclinical studies position BBT594 as a potential candidate for clinical trials targeting JAK2-dependent malignancies. Its unique mechanism of action and ability to inhibit both wild-type and mutant forms of JAK2 could provide a therapeutic advantage over existing treatments.
Eigenschaften
IUPAC Name |
5-(6-acetamidopyrimidin-4-yl)oxy-N-[4-[(4-methylpiperazin-1-yl)methyl]-3-(trifluoromethyl)phenyl]-2,3-dihydroindole-1-carboxamide | |
---|---|---|
Source | PubChem | |
URL | https://pubchem.ncbi.nlm.nih.gov | |
Description | Data deposited in or computed by PubChem | |
InChI |
InChI=1S/C28H30F3N7O3/c1-18(39)34-25-15-26(33-17-32-25)41-22-5-6-24-19(13-22)7-8-38(24)27(40)35-21-4-3-20(23(14-21)28(29,30)31)16-37-11-9-36(2)10-12-37/h3-6,13-15,17H,7-12,16H2,1-2H3,(H,35,40)(H,32,33,34,39) | |
Source | PubChem | |
URL | https://pubchem.ncbi.nlm.nih.gov | |
Description | Data deposited in or computed by PubChem | |
InChI Key |
VQLNKQZLPGLOSI-UHFFFAOYSA-N | |
Source | PubChem | |
URL | https://pubchem.ncbi.nlm.nih.gov | |
Description | Data deposited in or computed by PubChem | |
Canonical SMILES |
CC(=O)NC1=CC(=NC=N1)OC2=CC3=C(C=C2)N(CC3)C(=O)NC4=CC(=C(C=C4)CN5CCN(CC5)C)C(F)(F)F | |
Source | PubChem | |
URL | https://pubchem.ncbi.nlm.nih.gov | |
Description | Data deposited in or computed by PubChem | |
Molecular Formula |
C28H30F3N7O3 | |
Source | PubChem | |
URL | https://pubchem.ncbi.nlm.nih.gov | |
Description | Data deposited in or computed by PubChem | |
Molecular Weight |
569.6 g/mol | |
Source | PubChem | |
URL | https://pubchem.ncbi.nlm.nih.gov | |
Description | Data deposited in or computed by PubChem | |
Retrosynthesis Analysis
AI-Powered Synthesis Planning: Our tool employs the Template_relevance Pistachio, Template_relevance Bkms_metabolic, Template_relevance Pistachio_ringbreaker, Template_relevance Reaxys, Template_relevance Reaxys_biocatalysis model, leveraging a vast database of chemical reactions to predict feasible synthetic routes.
One-Step Synthesis Focus: Specifically designed for one-step synthesis, it provides concise and direct routes for your target compounds, streamlining the synthesis process.
Accurate Predictions: Utilizing the extensive PISTACHIO, BKMS_METABOLIC, PISTACHIO_RINGBREAKER, REAXYS, REAXYS_BIOCATALYSIS database, our tool offers high-accuracy predictions, reflecting the latest in chemical research and data.
Strategy Settings
Precursor scoring | Relevance Heuristic |
---|---|
Min. plausibility | 0.01 |
Model | Template_relevance |
Template Set | Pistachio/Bkms_metabolic/Pistachio_ringbreaker/Reaxys/Reaxys_biocatalysis |
Top-N result to add to graph | 6 |
Feasible Synthetic Routes
Q1: What is NVP-BBT594 and how does it work?
A1: this compound is a potent and selective type II inhibitor of Janus kinase 2 (JAK2). [, , , , , ] Unlike type I JAK2 inhibitors, which bind to the active conformation of the kinase, this compound targets the inactive conformation. [, , , ] This unique mechanism of action enables it to overcome resistance conferred by JAK2 heterodimerization, a mechanism by which type I inhibitors lose efficacy. [, , ] this compound effectively inhibits JAK/STAT signaling, a pathway often dysregulated in certain cancers like B-cell acute lymphoblastic leukemia (B-ALL). [, , , ]
Q2: Which cancers show sensitivity to this compound?
A2: Research has primarily focused on this compound's efficacy against hematological malignancies, specifically B-ALL subtypes characterized by CRLF2 rearrangements or JAK2 mutations. [, , , ] Preclinical studies demonstrate its effectiveness in inhibiting the growth of both CRLF2-rearranged and JAK2 mutation-positive B-ALL cells in vitro and in vivo. [, , , ] Additionally, this compound shows promise in estrogen receptor-positive (ER+) breast cancer models where GDNF-RET signaling contributes to resistance to aromatase inhibitors. [, ]
Q3: How does the efficacy of this compound compare to type I JAK2 inhibitors?
A3: this compound demonstrates superior efficacy compared to type I JAK2 inhibitors in certain contexts. In B-ALL cell lines with specific JAK2 mutations, like R683G, this compound exhibits increased potency and effectively inhibits JAK2 and STAT5 phosphorylation, unlike some type I inhibitors. [, ] Additionally, it effectively inhibits the growth of B-ALL cells harboring the JAK2 Y931C mutation, which confers resistance to several type I inhibitors. [, ]
Q4: Are there any resistance mechanisms to this compound?
A4: Yes, a mutation in JAK2, L884P, has been identified as a mechanism of resistance specific to this compound in the context of CRLF2/JAK2 R683G B-ALL. [] This mutation is suggested to alter the binding pocket for type II inhibitors, rendering them ineffective. [] Interestingly, this mutation does not confer resistance to type I JAK2 inhibitors. []
Q5: Are there any potential strategies to improve the efficacy of this compound?
A5: Research suggests combining this compound with other targeted therapies, such as mTOR inhibitors, could enhance its anti-leukemic effects. [, ] Preclinical data indicate that combining this compound with the mTOR kinase inhibitor AZD2014 induces synergistic cell death in JAK2-driven Ph-like ALL cells. [, ] Furthermore, combining this compound with dexamethasone, a chemotherapy agent, demonstrated significantly prolonged survival compared to monotherapy in a murine B-ALL model. []
Haftungsausschluss und Informationen zu In-Vitro-Forschungsprodukten
Bitte beachten Sie, dass alle Artikel und Produktinformationen, die auf BenchChem präsentiert werden, ausschließlich zu Informationszwecken bestimmt sind. Die auf BenchChem zum Kauf angebotenen Produkte sind speziell für In-vitro-Studien konzipiert, die außerhalb lebender Organismen durchgeführt werden. In-vitro-Studien, abgeleitet von dem lateinischen Begriff "in Glas", beinhalten Experimente, die in kontrollierten Laborumgebungen unter Verwendung von Zellen oder Geweben durchgeführt werden. Es ist wichtig zu beachten, dass diese Produkte nicht als Arzneimittel oder Medikamente eingestuft sind und keine Zulassung der FDA für die Vorbeugung, Behandlung oder Heilung von medizinischen Zuständen, Beschwerden oder Krankheiten erhalten haben. Wir müssen betonen, dass jede Form der körperlichen Einführung dieser Produkte in Menschen oder Tiere gesetzlich strikt untersagt ist. Es ist unerlässlich, sich an diese Richtlinien zu halten, um die Einhaltung rechtlicher und ethischer Standards in Forschung und Experiment zu gewährleisten.