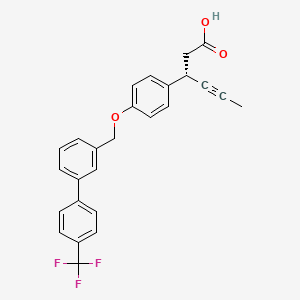
AMG-837
Übersicht
Beschreibung
Wissenschaftliche Forschungsanwendungen
AMG-837 has several scientific research applications, including:
Chemistry: The compound is used as a model compound to study the synthesis and reactivity of GPR40 agonists.
Biology: this compound is used to study the role of GPR40 in glucose metabolism and insulin secretion.
Wirkmechanismus
Target of Action
AMG-837, also known as (S)-3-(4-((4’-(Trifluoromethyl)-[1,1’-biphenyl]-3-yl)methoxy)phenyl)hex-4-ynoic acid, is a partial agonist of the GPR40 receptor , also known as FFAR1 . This receptor is primarily expressed in insulin-producing pancreatic β-cells and incretin-producing enteroendocrine cells of the small intestine .
Mode of Action
This compound interacts with the GPR40 receptor, leading to the activation of this receptor It has been found to increase glucose-stimulated insulin secretion (GSIS) in a glucose-dependent manner .
Biochemical Pathways
The activation of the GPR40 receptor by this compound leads to an increase in inositol phosphate accumulation and calcium flux . This results in the potentiation of glucose-stimulated insulin secretion, both in vitro and in vivo .
Pharmacokinetics
While specific ADME (Absorption, Distribution, Metabolism, and Excretion) properties of this compound are not readily available, it is noted that this compound is highly lipophilic (cLog P = 7.6) and likely to have significant CNS exposure . This suggests that the compound may be well-absorbed and distributed throughout the body, including the central nervous system.
Result of Action
The primary result of this compound’s action is the potentiation of glucose-stimulated insulin secretion . This leads to lowered glucose excursions and increased insulin secretion during glucose tolerance tests in both normal and Zucker fatty rats . The improvement in glucose excursions persists following daily dosing of this compound for 21 days in Zucker fatty rats .
Biochemische Analyse
Biochemical Properties
AMG-837 is a potent partial agonist on the GPR40 receptor . It stimulates inositol phosphate accumulation with an EC50 of 7.861.2 nM . This interaction with the GPR40 receptor is crucial for its role in biochemical reactions .
Cellular Effects
This compound potentiates glucose-stimulated insulin secretion in vitro and in vivo . This indicates that this compound has significant effects on cellular processes, particularly those related to glucose metabolism and insulin secretion .
Molecular Mechanism
The molecular mechanism of this compound involves its action as a GPR40 agonist . It binds to the GPR40 receptor, leading to the activation of inositol phosphate accumulation and Ca2+ flux assays . This binding interaction is key to its ability to enhance insulin secretion and lower glucose levels .
Temporal Effects in Laboratory Settings
In laboratory settings, the effects of this compound have been observed to persist over time . For instance, the improvement in glucose excursions persisted following daily dosing of this compound for 21-days in Zucker fatty rats .
Dosage Effects in Animal Models
In animal models, the effects of this compound vary with dosage . Acute administration of this compound lowered glucose excursions and increased glucose-stimulated insulin secretion during glucose tolerance tests in both normal and Zucker fatty rats .
Metabolic Pathways
This compound is involved in the metabolic pathway related to glucose metabolism and insulin secretion . It interacts with the GPR40 receptor, which plays a key role in these metabolic processes .
Vorbereitungsmethoden
Synthesewege und Reaktionsbedingungen
Die Synthese von AMG-837 beinhaltet eine vielseitige Methode für die Iridium-katalysierte asymmetrische Substitution racemischer Allylialkohole. Diese Methode veranschaulicht die Synthese von this compound, einem GPR40-Rezeptoragonisten . Die wichtigsten Schritte in der Synthese beinhalten die enantioselektive Alkynylierung von benzylischen C-H-Bindungen über einen kupferkatalysierten Radikalrelayprozess . Dieser Prozess ermöglicht einen einfachen Zugang zu strukturell diversen benzylischen Alkinen in guten Ausbeuten mit exzellenten Enantioselektivitäten .
Industrielle Produktionsmethoden
Die industrielle Produktion von this compound beinhaltet die Optimierung der Synthesewege, um eine hohe Ausbeute und Reinheit zu gewährleisten. Der Prozess umfasst die Verwendung von Hochdurchsatz-Screening-Treffern und Leadoptimierung, um die effizientesten Synthesewege zu identifizieren . Die Produktionsmethoden sind so konzipiert, dass sie skalierbar und kostengünstig sind, um sicherzustellen, dass die Verbindung in großen Mengen für klinische und kommerzielle Zwecke hergestellt werden kann .
Analyse Chemischer Reaktionen
Arten von Reaktionen
AMG-837 durchläuft verschiedene Arten von chemischen Reaktionen, darunter:
Oxidation: Die Verbindung kann oxidiert werden, um verschiedene Oxidationsprodukte zu bilden.
Reduktion: Reduktionsreaktionen können verwendet werden, um die funktionellen Gruppen in this compound zu modifizieren.
Substitution: Die Verbindung kann Substitutionsreaktionen eingehen, um bestimmte funktionelle Gruppen durch andere zu ersetzen.
Häufige Reagenzien und Bedingungen
Die häufigen Reagenzien und Bedingungen, die in den Reaktionen mit this compound verwendet werden, umfassen:
Oxidationsmittel: Wie Kaliumpermanganat oder Chromtrioxid.
Reduktionsmittel: Wie Lithiumaluminiumhydrid oder Natriumborhydrid.
Katalysatoren: Wie Palladium- oder Kupferkatalysatoren für Substitutionsreaktionen.
Hauptprodukte, die gebildet werden
Die Hauptprodukte, die aus den Reaktionen mit this compound gebildet werden, umfassen verschiedene Derivate, die die Kernstruktur der Verbindung beibehalten, während neue funktionelle Gruppen eingeführt werden. Diese Derivate können verwendet werden, um die Struktur-Aktivitäts-Beziehung zu untersuchen und die pharmakologischen Eigenschaften der Verbindung zu optimieren .
Wissenschaftliche Forschungsanwendungen
This compound hat mehrere wissenschaftliche Forschungsanwendungen, darunter:
Chemie: Die Verbindung wird als Modellverbindung verwendet, um die Synthese und Reaktivität von GPR40-Agonisten zu untersuchen.
Biologie: this compound wird verwendet, um die Rolle von GPR40 im Glukosestoffwechsel und der Insulinsekretion zu untersuchen.
Wirkmechanismus
This compound übt seine Wirkung aus, indem es als partieller Agonist für den GPR40-Rezeptor wirkt. Die Aktivierung von GPR40 durch this compound führt zur Stimulation der glukoseabhängigen Insulinsekretion aus pankreatischen Betazellen . Dieser Mechanismus beinhaltet die Bindung von this compound an den GPR40-Rezeptor, der eine Kaskade intrazellulärer Signalereignisse auslöst, einschließlich der Aktivierung der GTPγS-Bindung, der Ansammlung von Inositolphosphat und der Calciumfluss-Assays . Diese Ereignisse führen letztendlich zur Potenzierung der Insulinsekretion und zur Senkung des Glukosespiegels im Blut .
Vergleich Mit ähnlichen Verbindungen
AMG-837 wird mit anderen ähnlichen Verbindungen verglichen, wie z. B.:
Einzigartigkeit von this compound
This compound ist einzigartig in seiner Fähigkeit, als potenter partieller Agonist für den GPR40-Rezeptor zu wirken, was es ihm ermöglicht, die Insulinsekretion effektiv zu steigern und den Glukosespiegel zu senken. Seine hohe Zielselektivität und Potenz machen ihn zu einem vielversprechenden Kandidaten für die Behandlung von Typ-2-Diabetes .
Biologische Aktivität
(S)-3-(4-((4'-(Trifluoromethyl)-[1,1'-biphenyl]-3-yl)methoxy)phenyl)hex-4-ynoic acid is a synthetic compound notable for its potential biological activities. This article reviews the existing literature on its biological properties, including its pharmacological effects, mechanisms of action, and relevant case studies.
Chemical Structure and Properties
The compound features a complex structure characterized by a trifluoromethyl group attached to a biphenyl moiety and a hex-4-ynoic acid backbone. Its molecular formula is , indicating significant lipophilicity due to the presence of fluorinated groups which can enhance membrane permeability.
Biological Activity Overview
Research has indicated that this compound exhibits various biological activities, including:
- Anticancer Properties : Preliminary studies suggest that (S)-3-(4-((4'-(trifluoromethyl)-[1,1'-biphenyl]-3-yl)methoxy)phenyl)hex-4-ynoic acid may inhibit the proliferation of certain cancer cell lines. The mechanism appears to involve the modulation of signaling pathways related to cell growth and apoptosis.
- Anti-inflammatory Effects : The compound has been evaluated for its potential to reduce inflammation in vitro. It seems to inhibit the production of pro-inflammatory cytokines, suggesting a role in managing inflammatory diseases.
The exact mechanisms by which (S)-3-(4-((4'-(trifluoromethyl)-[1,1'-biphenyl]-3-yl)methoxy)phenyl)hex-4-ynoic acid exerts its effects are still under investigation. However, several hypotheses include:
- Inhibition of Enzyme Activity : The compound may act as an inhibitor of specific enzymes involved in cancer progression and inflammatory responses.
- Receptor Modulation : There is evidence that it may interact with various cellular receptors, influencing downstream signaling pathways critical for cell survival and proliferation.
Research Findings
Recent studies have provided insights into the biological activity of this compound:
Table 1: Summary of Biological Activities
Activity Type | Effect Observed | Reference |
---|---|---|
Anticancer | Inhibition of cell proliferation | |
Anti-inflammatory | Reduction in cytokine production | |
Enzyme Inhibition | Potential inhibition of key enzymes |
Case Studies
Case Study 1: Anticancer Activity
A study conducted on breast cancer cell lines demonstrated that treatment with (S)-3-(4-((4'-(trifluoromethyl)-[1,1'-biphenyl]-3-yl)methoxy)phenyl)hex-4-ynoic acid resulted in a significant reduction in cell viability. The IC50 value was determined to be approximately 15 µM, indicating potent activity against these cells.
Case Study 2: Anti-inflammatory Effects
In a model of acute inflammation induced by lipopolysaccharide (LPS), administration of the compound led to a marked decrease in inflammatory markers such as TNF-alpha and IL-6. This suggests potential therapeutic applications in conditions like rheumatoid arthritis or other inflammatory diseases.
Eigenschaften
IUPAC Name |
(3S)-3-[4-[[3-[4-(trifluoromethyl)phenyl]phenyl]methoxy]phenyl]hex-4-ynoic acid | |
---|---|---|
Source | PubChem | |
URL | https://pubchem.ncbi.nlm.nih.gov | |
Description | Data deposited in or computed by PubChem | |
InChI |
InChI=1S/C26H21F3O3/c1-2-4-21(16-25(30)31)20-9-13-24(14-10-20)32-17-18-5-3-6-22(15-18)19-7-11-23(12-8-19)26(27,28)29/h3,5-15,21H,16-17H2,1H3,(H,30,31)/t21-/m0/s1 | |
Source | PubChem | |
URL | https://pubchem.ncbi.nlm.nih.gov | |
Description | Data deposited in or computed by PubChem | |
InChI Key |
ZOPNBMMVVZRSGH-NRFANRHFSA-N | |
Source | PubChem | |
URL | https://pubchem.ncbi.nlm.nih.gov | |
Description | Data deposited in or computed by PubChem | |
Canonical SMILES |
CC#CC(CC(=O)O)C1=CC=C(C=C1)OCC2=CC(=CC=C2)C3=CC=C(C=C3)C(F)(F)F | |
Source | PubChem | |
URL | https://pubchem.ncbi.nlm.nih.gov | |
Description | Data deposited in or computed by PubChem | |
Isomeric SMILES |
CC#C[C@@H](CC(=O)O)C1=CC=C(C=C1)OCC2=CC(=CC=C2)C3=CC=C(C=C3)C(F)(F)F | |
Source | PubChem | |
URL | https://pubchem.ncbi.nlm.nih.gov | |
Description | Data deposited in or computed by PubChem | |
Molecular Formula |
C26H21F3O3 | |
Source | PubChem | |
URL | https://pubchem.ncbi.nlm.nih.gov | |
Description | Data deposited in or computed by PubChem | |
DSSTOX Substance ID |
DTXSID00676764 | |
Record name | (3S)-3-(4-{[4'-(Trifluoromethyl)[biphenyl]-3-yl]methoxy}phenyl)-4-hexynoic acid | |
Source | EPA DSSTox | |
URL | https://comptox.epa.gov/dashboard/DTXSID00676764 | |
Description | DSSTox provides a high quality public chemistry resource for supporting improved predictive toxicology. | |
Molecular Weight |
438.4 g/mol | |
Source | PubChem | |
URL | https://pubchem.ncbi.nlm.nih.gov | |
Description | Data deposited in or computed by PubChem | |
CAS No. |
865231-46-5 | |
Record name | AMG-837 | |
Source | ChemIDplus | |
URL | https://pubchem.ncbi.nlm.nih.gov/substance/?source=chemidplus&sourceid=0865231465 | |
Description | ChemIDplus is a free, web search system that provides access to the structure and nomenclature authority files used for the identification of chemical substances cited in National Library of Medicine (NLM) databases, including the TOXNET system. | |
Record name | (3S)-3-(4-{[4'-(Trifluoromethyl)[biphenyl]-3-yl]methoxy}phenyl)-4-hexynoic acid | |
Source | EPA DSSTox | |
URL | https://comptox.epa.gov/dashboard/DTXSID00676764 | |
Description | DSSTox provides a high quality public chemistry resource for supporting improved predictive toxicology. | |
Record name | AMG-837 | |
Source | FDA Global Substance Registration System (GSRS) | |
URL | https://gsrs.ncats.nih.gov/ginas/app/beta/substances/NE6U6R66U4 | |
Description | The FDA Global Substance Registration System (GSRS) enables the efficient and accurate exchange of information on what substances are in regulated products. Instead of relying on names, which vary across regulatory domains, countries, and regions, the GSRS knowledge base makes it possible for substances to be defined by standardized, scientific descriptions. | |
Explanation | Unless otherwise noted, the contents of the FDA website (www.fda.gov), both text and graphics, are not copyrighted. They are in the public domain and may be republished, reprinted and otherwise used freely by anyone without the need to obtain permission from FDA. Credit to the U.S. Food and Drug Administration as the source is appreciated but not required. | |
Haftungsausschluss und Informationen zu In-Vitro-Forschungsprodukten
Bitte beachten Sie, dass alle Artikel und Produktinformationen, die auf BenchChem präsentiert werden, ausschließlich zu Informationszwecken bestimmt sind. Die auf BenchChem zum Kauf angebotenen Produkte sind speziell für In-vitro-Studien konzipiert, die außerhalb lebender Organismen durchgeführt werden. In-vitro-Studien, abgeleitet von dem lateinischen Begriff "in Glas", beinhalten Experimente, die in kontrollierten Laborumgebungen unter Verwendung von Zellen oder Geweben durchgeführt werden. Es ist wichtig zu beachten, dass diese Produkte nicht als Arzneimittel oder Medikamente eingestuft sind und keine Zulassung der FDA für die Vorbeugung, Behandlung oder Heilung von medizinischen Zuständen, Beschwerden oder Krankheiten erhalten haben. Wir müssen betonen, dass jede Form der körperlichen Einführung dieser Produkte in Menschen oder Tiere gesetzlich strikt untersagt ist. Es ist unerlässlich, sich an diese Richtlinien zu halten, um die Einhaltung rechtlicher und ethischer Standards in Forschung und Experiment zu gewährleisten.