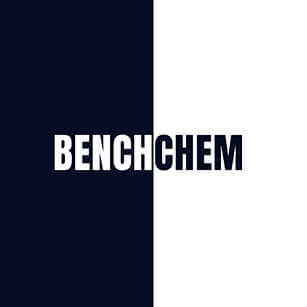
Pyridoxal-d5 5-Phosphat
- Klicken Sie auf QUICK INQUIRY, um ein Angebot von unserem Expertenteam zu erhalten.
- Mit qualitativ hochwertigen Produkten zu einem WETTBEWERBSFÄHIGEN Preis können Sie sich mehr auf Ihre Forschung konzentrieren.
Übersicht
Beschreibung
Pyridoxal-d5 5-Phosphate is a deuterated form of Pyridoxal 5-Phosphate, which is an active form of vitamin B6. This compound is crucial in various biochemical processes, particularly as a coenzyme in enzymatic reactions involving amino acids. The deuterated form, Pyridoxal-d5 5-Phosphate, is often used in research to study metabolic pathways and enzyme mechanisms due to its stability and traceability.
Wissenschaftliche Forschungsanwendungen
Biochemical Research
Pyridoxal 5'-phosphate is the active form of vitamin B6 and is essential for numerous enzymatic reactions, particularly those involving amino acid metabolism. The deuterated form, Pyridoxal-d5 5-Phosphate, is used in metabolic studies to trace the pathways of vitamin B6 and its derivatives within biological systems.
Case Study: Metabolic Pathway Analysis
A study utilized Pyridoxal-d5 5-Phosphate to investigate the metabolic pathways of vitamin B6 in human subjects. By tracking the incorporation of deuterium into metabolites, researchers were able to elucidate the conversion rates and identify bottlenecks in vitamin B6 metabolism, providing insights into deficiencies and potential therapeutic interventions .
Cancer Research
Recent studies have highlighted the protective role of pyridoxal 5'-phosphate against colorectal cancer. Research indicates that higher serum levels of pyridoxal 5'-phosphate correlate with reduced cancer risk and improved survival rates among patients.
Data Table: Colorectal Cancer Risk and Pyridoxal 5'-Phosphate Levels
Serum Level Quartile | Odds Ratio (OR) | Confidence Interval (CI) |
---|---|---|
Q1 (Lowest) | Reference | - |
Q2 | 0.51 | (0.40−0.66) |
Q3 | 0.26 | (0.20−0.33) |
Q4 (Highest) | 0.63 | (0.46−0.87) |
This table summarizes findings from a large-scale study involving over 2,500 participants, indicating that higher levels of pyridoxal 5'-phosphate are inversely associated with colorectal cancer risk .
Neurological Disorders
Pyridoxal 5'-phosphate has been investigated for its potential benefits in treating tardive dyskinesia, a movement disorder associated with long-term antipsychotic medication use.
Case Study: Treatment of Tardive Dyskinesia
A systematic review analyzed three randomized controlled trials involving patients with schizophrenia who experienced tardive dyskinesia. Results indicated that those treated with pyridoxal 5'-phosphate showed significant improvement in symptoms compared to placebo groups, as measured by the Extrapyramidal Symptoms Rating Scale .
Data Table: Efficacy of Pyridoxal 5'-Phosphate in Tardive Dyskinesia
Treatment Group | Mean Difference (MD) | Confidence Interval (CI) |
---|---|---|
Pyridoxal 5'-Phosphate | -4.07 | (-6.36 to -1.79) |
Placebo | Reference | - |
The evidence suggests that while the quality is low due to small sample sizes and short follow-up periods, pyridoxal 5'-phosphate may effectively reduce tardive dyskinesia symptoms .
Implications for Future Research
The diverse applications of Pyridoxal-d5 5-Phosphate emphasize its importance in both basic and clinical research. Future studies could focus on:
- The role of pyridoxal 5'-phosphate in other cancers beyond colorectal.
- Longitudinal studies assessing the long-term effects of pyridoxal 5'-phosphate supplementation in psychiatric disorders.
- Investigating the biochemical mechanisms underlying its protective effects against oxidative stress and inflammation.
Wirkmechanismus
1. Target of Action: Pyridoxal-d5 5-Phosphate (PLP) primarily targets enzymes involved in amino acid metabolism. It acts as a coenzyme for various enzymatic reactions, including transamination, decarboxylation, and racemization. These enzymes play crucial roles in synthesizing neurotransmitters, hemoglobin, and other essential biomolecules .
2. Mode of Action: PLP forms a Schiff base with the amino group of amino acids, facilitating the transfer of amino groups in transamination reactions. This interaction converts PLP into pyridoxamine phosphate (PMP), which then participates in further reactions. The reversible nature of this interaction allows PLP to act as a versatile coenzyme in multiple biochemical processes .
3. Biochemical Pathways: PLP is involved in several key biochemical pathways:
Pharmacokinetics:
5. Result of Action: At the molecular level, PLP’s action results in the efficient metabolism of amino acids and the synthesis of vital biomolecules. At the cellular level, this translates to proper cellular function, neurotransmission, and oxygen transport. Deficiency in PLP can lead to various disorders, including anemia, neurological issues, and impaired immune function.
6. Action Environment: Environmental factors such as pH, temperature, and the presence of other cofactors can influence PLP’s efficacy and stability. For instance, extreme pH levels can denature the enzymes PLP interacts with, reducing its effectiveness. Similarly, high temperatures can lead to the degradation of PLP, while the presence of other cofactors can enhance its activity by stabilizing enzyme structures.
Pyridoxal-d5 5-Phosphate is a vital coenzyme with a broad range of biochemical roles, making it essential for maintaining various physiological functions.
: DrugBank : DrugBank : Nutrition Reviews : Springer : DrugBank : Springer
Biochemische Analyse
Biochemical Properties
Pyridoxal-d5 5-Phosphate: participates in several biochemical reactions, including transamination, α-decarboxylation, β-decarboxylation, β-elimination, γ-elimination, racemization, and aldol reactions . It acts as a coenzyme in all transamination reactions and some decarboxylation and deamination reactions of amino acids . The aldehyde group of Pyridoxal-d5 5-Phosphate forms a Schiff base (internal aldimine) with a specific lysine group of the aminotransferase enzyme .
Cellular Effects
Pyridoxal-d5 5-Phosphate: influences cell function by impacting cell signaling pathways, gene expression, and cellular metabolism . It plays a role in the synthesis of neurotransmitters, hemoglobin, and nucleic acids . It also participates in the conversion of tryptophan to niacin .
Molecular Mechanism
The molecular mechanism of Pyridoxal-d5 5-Phosphate involves binding interactions with biomolecules, enzyme inhibition or activation, and changes in gene expression . The aldimine formed between Pyridoxal-d5 5-Phosphate and the enzyme is a key intermediate in these reactions .
Temporal Effects in Laboratory Settings
In laboratory settings, the effects of Pyridoxal-d5 5-Phosphate can change over time. It is stable under physiological conditions, but can degrade under certain conditions . Long-term effects on cellular function have been observed in in vitro or in vivo studies .
Dosage Effects in Animal Models
The effects of Pyridoxal-d5 5-Phosphate can vary with different dosages in animal models . High doses can lead to neurotoxicity, while deficiency can cause dermatitis, microcytic anemia, and neurological symptoms .
Metabolic Pathways
Pyridoxal-d5 5-Phosphate: is involved in various metabolic pathways, interacting with enzymes and cofactors . It plays a role in the metabolism of all amino acids, glucose, lipids, and nucleic acids .
Transport and Distribution
Pyridoxal-d5 5-Phosphate: is transported and distributed within cells and tissues . It is taken up by cells via facilitated diffusion and active transport .
Subcellular Localization
Pyridoxal-d5 5-Phosphate: is localized in the cytoplasm, mitochondria, and nucleus . It is directed to specific compartments or organelles based on its role in various biochemical reactions .
Vorbereitungsmethoden
Synthetic Routes and Reaction Conditions: The synthesis of Pyridoxal-d5 5-Phosphate typically involves the deuteration of Pyridoxal 5-Phosphate. One common method includes the reaction of Pyridoxal with deuterated reagents to introduce deuterium atoms. The process involves several steps:
Formation of Pyridoxal Schiff Base: Pyridoxal reacts with phenetidine to form a Schiff base.
Dissolution in Ionic Liquid: The Schiff base is dissolved in an ionic liquid, which acts as a solvent.
Addition of Polyphosphoric Acid: Polyphosphoric acid is added to the solution, and the mixture is stirred until the reaction is complete.
Hydrolysis and Purification: The resulting Schiff base is hydrolyzed and purified to obtain Pyridoxal-d5 5-Phosphate.
Industrial Production Methods: Industrial production of Pyridoxal-d5 5-Phosphate follows similar synthetic routes but on a larger scale. The use of ionic liquids and polyphosphoric acid ensures high yield and efficiency. The process is designed to be economical and environmentally friendly, utilizing green solvents and minimizing waste.
Analyse Chemischer Reaktionen
Types of Reactions: Pyridoxal-d5 5-Phosphate undergoes various chemical reactions, including:
Transamination: It acts as a coenzyme in the transfer of amino groups between amino acids and keto acids.
Decarboxylation: It facilitates the removal of carboxyl groups from amino acids.
Deamination: It assists in the removal of amino groups from amino acids.
Common Reagents and Conditions:
Transamination: Requires amino acids and keto acids, typically under physiological conditions.
Decarboxylation: Often involves enzymes like decarboxylases and occurs under mild conditions.
Deamination: Utilizes deaminases and occurs in aqueous solutions at neutral pH.
Major Products:
Transamination: Produces new amino acids and keto acids.
Decarboxylation: Results in the formation of amines and carbon dioxide.
Deamination: Leads to the production of keto acids and ammonia.
Vergleich Mit ähnlichen Verbindungen
Pyridoxal 5-Phosphate: The non-deuterated form, widely used in biochemical studies.
Pyridoxine 5-Phosphate: Another form of vitamin B6, involved in similar biochemical processes.
Pyridoxamine 5-Phosphate: A related compound with distinct enzymatic roles.
Uniqueness: Pyridoxal-d5 5-Phosphate is unique due to its deuterium content, which provides stability and allows for precise tracking in metabolic studies. This makes it particularly valuable in research settings where detailed analysis of metabolic pathways is required .
Biologische Aktivität
Pyridoxal-d5 5'-phosphate (PLP-d5) is a deuterated form of pyridoxal 5'-phosphate (PLP), the active coenzyme form of vitamin B6. This compound plays a crucial role in various biochemical processes, particularly in enzymatic reactions involving amino acids. The biological activity of PLP-d5 is closely related to its ability to participate in diverse metabolic pathways, including neurotransmitter synthesis, amino acid metabolism, and the modulation of physiological processes such as blood pressure regulation.
1. Enzymatic Functions
PLP-d5 acts as a coenzyme for over 160 enzyme-catalyzed reactions, primarily those involving amino acids. Key enzymatic activities include:
- Decarboxylation : PLP-d5 facilitates the conversion of amino acids into neurotransmitters. For instance, it is essential for the conversion of L-tryptophan to serotonin and L-histidine to histamine .
- Transamination : It aids in the transfer of amino groups between amino acids and α-keto acids, playing a vital role in amino acid metabolism .
- Racemization : PLP-d5 is involved in converting L-amino acids to their D-forms, which is crucial for various biological functions .
2. Mechanistic Insights
The mechanism of action for PLP-d5 involves the formation of a Schiff base with the substrate amino acid, which enhances its electrophilicity and facilitates subsequent catalytic steps. This process is essential for stabilizing reaction intermediates, allowing for efficient enzymatic reactions .
Table 1: Key Enzymatic Reactions Involving PLP-d5
Enzyme Type | Substrate | Product | Reaction Type |
---|---|---|---|
Aromatic L-amino acid decarboxylase | L-Tryptophan | Serotonin | Decarboxylation |
L-Histidine decarboxylase | L-Histidine | Histamine | Decarboxylation |
Glutamic acid decarboxylase | Glutamate | GABA | Decarboxylation |
Transaminases | Various amino acids | Corresponding α-keto acids | Transamination |
3. Physiological Implications
Recent studies have highlighted the potential therapeutic applications of PLP-d5 in managing various health conditions:
- Hypertension : Research indicates that PLP can induce post-translational modifications of angiotensin II (Ang II), leading to decreased blood pressure in hypertensive models. This suggests that PLP-d5 might serve as a cost-effective treatment for hypertension by modulating vascular smooth muscle cell responses .
- Neurological Disorders : Given its role in neurotransmitter synthesis, adequate levels of PLP are essential for neurological health. Deficiencies can lead to conditions such as seizures and mood disorders due to impaired GABA and serotonin production .
Case Study 1: Hypertensive Rat Model
In a study involving spontaneously hypertensive rats (SHR), administration of PLP resulted in significant reductions in blood pressure compared to control groups receiving only Ang II. The study demonstrated that PLP modifies Ang II into a less potent form (Ang P), which shows lower binding affinity to angiotensin receptors and reduced calcium ion entry into vascular smooth muscle cells .
Case Study 2: Genetic Disorders
Patients with inherited metabolic disorders related to PLP-dependent enzymes have shown improvement with pyridoxine supplementation. For example, individuals with AADC deficiency exhibited enhanced enzyme activity and improved clinical outcomes when treated with PLP or its precursors .
Eigenschaften
CAS-Nummer |
1246818-16-5 |
---|---|
Molekularformel |
C8H5D5NO6P |
Molekulargewicht |
252.17 |
Aussehen |
Light Yellow Solid |
melting_point |
>127°C (dec.) |
Reinheit |
> 95% |
Menge |
Milligrams-Grams |
Verwandte CAS-Nummern |
54-47-7 (unlabelled) |
Synonyme |
3-Hydroxy-2-methyl-5-[(phosphonooxy)methyl]-4-pyridinecarboxaldehyde-d5; Pyridoxal-d5 Phosphate; Pyridoxal-d5 5-(Dihydrogen Phosphate); 2-Methyl-3-hydroxy-4-formyl-5-pyridyl-methylphosphoric Acid-d5; 3-Hydroxy-5-(hydroxymethyl)-2-methylisonicotinaldehyde-d5 5-Phosphate; Apolon B6-d5; Biosechs-d5; Codecarboxylase-d5; Coenzyme B6-d5; Hairoxal-d5; Hexermin P-d5; Hi-Pyridoxin-d5; Hiadelon-d5; NSC 82388-d5; PLP-d5; Phosphopyridoxal-d5; Piodel-d5; Pydoxal-d5; Pyridoxal-d5 Monophosphate; Vitazechs-d5 |
Tag |
Pyridoxal |
Herkunft des Produkts |
United States |
Haftungsausschluss und Informationen zu In-Vitro-Forschungsprodukten
Bitte beachten Sie, dass alle Artikel und Produktinformationen, die auf BenchChem präsentiert werden, ausschließlich zu Informationszwecken bestimmt sind. Die auf BenchChem zum Kauf angebotenen Produkte sind speziell für In-vitro-Studien konzipiert, die außerhalb lebender Organismen durchgeführt werden. In-vitro-Studien, abgeleitet von dem lateinischen Begriff "in Glas", beinhalten Experimente, die in kontrollierten Laborumgebungen unter Verwendung von Zellen oder Geweben durchgeführt werden. Es ist wichtig zu beachten, dass diese Produkte nicht als Arzneimittel oder Medikamente eingestuft sind und keine Zulassung der FDA für die Vorbeugung, Behandlung oder Heilung von medizinischen Zuständen, Beschwerden oder Krankheiten erhalten haben. Wir müssen betonen, dass jede Form der körperlichen Einführung dieser Produkte in Menschen oder Tiere gesetzlich strikt untersagt ist. Es ist unerlässlich, sich an diese Richtlinien zu halten, um die Einhaltung rechtlicher und ethischer Standards in Forschung und Experiment zu gewährleisten.