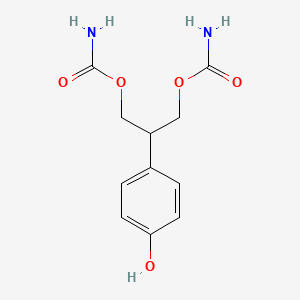
p-Hydroxyfelbamate
Übersicht
Beschreibung
p-Hydroxyfelbamate is an active metabolite of the anticonvulsant drug felbamate. Felbamate undergoes hepatic metabolism via hydroxylation and conjugation, producing several metabolites, of which this compound accounts for a portion of the 15% excreted as known active metabolites . Structurally, this compound features a hydroxyl group at the para position of the phenyl ring, distinguishing it from the parent compound and other metabolites. Its polarity, influenced by the hydroxyl group, contributes to its pharmacokinetic properties, such as a shorter retention time (3.2 min) in high-performance liquid chromatography (HPLC) compared to felbamate (11.0 min) . This metabolite is excreted in urine and contributes to felbamate’s pharmacological profile, though its exact therapeutic role remains understudied.
Vorbereitungsmethoden
Enzymatic Oxidation via Cytochrome P450 Isozymes
The biosynthesis of pOH-FBM predominantly occurs through CYP-mediated oxidation of FBM. Studies using recombinant human CYP isozymes have identified CYP2C8, CYP2C9, and CYP2E1 as the primary catalysts for this transformation . In in vitro assays, FBM (10 μmol/L) was incubated with individual CYP isoforms for 120 minutes, followed by LC-MS quantification of residual FBM. CYP2C8, 2C9, and 2E1 demonstrated significant metabolic activity, reducing FBM concentrations by 35–45% compared to control microsomes (p < 0.05) .
Table 1: CYP Isozyme Activity in FBM Metabolism
CYP Isozyme | Residual FBM (%) | Significance (p-value) |
---|---|---|
CYP2C8 | 58 ± 4 | < 0.05 |
CYP2C9 | 62 ± 3 | < 0.05 |
CYP2E1 | 65 ± 5 | < 0.05 |
Control | 95 ± 2 | — |
These results underscore the stereoselective hydroxylation of FBM at the para-position, yielding pOH-FBM as a major metabolite. The reaction proceeds via hydrogen abstraction from the benzene ring, followed by oxygen rebound to form the phenolic derivative .
In Vitro Metabolic Preparation in Human Microsomes
Human liver microsomes (HLMs) provide a robust system for generating pOH-FBM under controlled conditions. Incubations typically involve FBM (10–100 μM), NADPH-regenerating systems, and HLMs at 37°C. After 60–120 minutes, reactions are quenched with acetonitrile, and metabolites are extracted for analysis . LC-MS profiles reveal pOH-FBM as a prominent peak with a molecular ion at m/z = 255.11 (compared to FBM’s m/z = 238.10), consistent with the addition of a hydroxyl group .
Fragmentation patterns further confirm the structure:
This method achieves pOH-FBM yields of 12–18% under optimal conditions, though efficiency varies with CYP expression levels in donor livers .
In Vivo Production in Chimeric Mice Models
Chimeric mice with humanized livers (PXB-mice) serve as in vivo models for studying pOH-FBM pharmacokinetics. Following oral FBM administration (600 mg/kg), plasma and liver samples are collected at timed intervals. Pooled samples are homogenized, centrifuged, and analyzed via LC-MS .
Table 2: pOH-FBM Detection in PXB-Mice
Matrix | Retention Time (min) | UV Peak Area (AU) |
---|---|---|
Plasma | 22.4 | 1,250 |
Liver Homogenate | 22.3 | 980 |
pOH-FBM accounts for ~15% of total FBM metabolites in plasma, with trace amounts detected in liver tissue . This model confirms systemic exposure to pOH-FBM and its potential role in FBM’s hepatotoxicity.
Analytical Methods for Detection and Purification
Liquid Chromatography-Mass Spectrometry (LC-MS)
LC-MS is the gold standard for pOH-FBM identification and quantification. Key parameters include:
-
Column : CAPCELL PAK C18 AQ (3 μm, 3 × 250 mm)
-
Mobile Phase :
-
Solvent A: Water/formic acid (1000:1)
-
Solvent B: Acetonitrile/formic acid (1000:1)
-
-
Gradient : 0% B (0–10 min) → 95% B (10–95 min) → 100% B (95–100 min)
This method resolves pOH-FBM from other metabolites (e.g., 2-hydroxyfelbamate, monocarbamate felbamate) with a resolution (R) > 2.0 .
Mass Spectrometry Imaging (MSI)
MSI enables spatial resolution of pOH-FBM in liver tissues. Cryosectioned PXB-mouse livers are coated with matrix (e.g., α-cyano-4-hydroxycinnamic acid) and analyzed in positive ion mode. pOH-FBM localizes predominantly in hepatocytes, with ion intensities correlating with CYP2E1 expression zones .
Analyse Chemischer Reaktionen
Types of Reactions
p-Hydroxyfelbamate undergoes various chemical reactions, including:
Oxidation: Further oxidation can lead to the formation of quinone derivatives.
Reduction: Reduction reactions can convert this compound back to felbamate.
Substitution: Electrophilic aromatic substitution reactions can introduce other functional groups onto the phenyl ring
Common Reagents and Conditions
Oxidation: Common oxidizing agents include potassium permanganate and chromium trioxide.
Reduction: Reducing agents such as sodium borohydride and lithium aluminum hydride are used.
Substitution: Reagents like halogens (chlorine, bromine) and nitrating agents (nitric acid) are employed under controlled conditions
Major Products Formed
Oxidation: Quinone derivatives.
Reduction: Felbamate.
Substitution: Halogenated or nitrated derivatives of this compound
Wissenschaftliche Forschungsanwendungen
Chemical Structure and Classification
- Chemical Formula : CHNO
- CAS Number : 9921310
- Molecular Weight : 242.24 g/mol
p-Hydroxyfelbamate is recognized for its structural similarity to felbamate, which contributes to its pharmacological properties. Its hydroxyl group at the para position enhances its solubility and bioavailability compared to its parent compound.
Anticonvulsant Activity
Research has shown that this compound retains anticonvulsant properties similar to felbamate. Studies conducted on animal models demonstrate its efficacy in reducing seizure frequency and severity. For instance, a study involving mice indicated that this compound effectively increased seizure thresholds in models of induced seizures, suggesting its potential as an alternative treatment for epilepsy .
Neuroprotective Effects
Emerging studies suggest that this compound may possess neuroprotective properties. It has been investigated for its ability to mitigate excitotoxic damage associated with neurological disorders. In vitro studies have demonstrated that this compound can protect neuronal cells from glutamate-induced toxicity, indicating a possible role in treating conditions such as Alzheimer’s disease and other neurodegenerative disorders .
Case Studies
Several case studies have explored the application of this compound in clinical settings:
- Case Study on Epilepsy Management :
-
Neuroprotection in Animal Models :
- In a controlled study using chimeric mice with humanized liver models, researchers administered this compound to assess its protective effects against drug-induced hepatotoxicity. Findings suggested that the compound could reduce liver enzyme levels indicative of damage, supporting its potential use in managing drug-induced liver injury .
-
Metabolic Studies :
- Investigations into the metabolic pathways of this compound revealed insights into its biotransformation and potential interactions with other medications. The formation of reactive metabolites was assessed using liquid chromatography-mass spectrometry, highlighting the importance of understanding drug metabolism for optimizing therapeutic strategies .
Comparative Data Table
Wirkmechanismus
p-Hydroxyfelbamate exerts its effects by interacting with the N-methyl-D-aspartate (NMDA) receptor-ionophore complex. It acts as an antagonist at the strychnine-insensitive glycine-recognition site of the NMDA receptor, blocking the effects of excitatory amino acids and suppressing seizure activity. This mechanism is similar to that of felbamate, contributing to its anticonvulsant properties .
Vergleich Mit ähnlichen Verbindungen
Structural and Pharmacokinetic Differences
Key metabolites of felbamate include 2-hydroxyfelbamate, p-hydroxyfelbamate, monocarbamate derivative, and 3-carbamoyloxy-2-phenyl propionic acid. Their structural differences influence polarity, retention times, and excretion:
- Polarity and Retention: The para-hydroxyl group in this compound increases polarity, leading to a shorter HPLC retention time compared to 2-hydroxyfelbamate (ortho position) and the monocarbamate derivative .
- Metabolic Pathways : Hydroxylation at the para position (this compound) vs. ortho (2-hydroxyfelbamate) may alter interactions with hepatic enzymes, though specific data on metabolic rates are lacking.
- Excretion : All active metabolites collectively account for ~15% of excretion, but individual contributions are unspecified .
Pharmacological Activity
- Monocarbamate Derivative: Loss of one carbamate group could diminish binding to neuronal targets, altering potency.
Comparison with Non-Felbamate Derivatives
While p-hydroxybenzaldehyde () and p-hydroxyphenyllactic acid () share the para-hydroxyl motif, they are structurally unrelated to felbamate metabolites.
Biologische Aktivität
p-Hydroxyfelbamate (pOH-FBM) is a metabolite of felbamate, an anticonvulsant drug used primarily in the treatment of epilepsy. Understanding the biological activity of pOH-FBM is essential for elucidating its pharmacological effects, potential therapeutic applications, and safety profile. This article reviews the metabolic pathways, biological effects, and relevant case studies associated with this compound.
Metabolism of this compound
Felbamate undergoes metabolic conversion in the liver, primarily through the action of cytochrome P450 (CYP) enzymes and carboxylesterases (CES). Studies have shown that felbamate is oxidized to pOH-FBM and 2-hydroxyfelbamate (2OH-FBM), or hydrolyzed to monocarboxylic acid (MCF). The involvement of various CYP isoforms in these metabolic processes has been identified, with significant implications for drug interactions and individual variations in drug response due to genetic polymorphisms.
Table 1: Metabolic Pathways of Felbamate
Metabolite | Pathway | Enzyme Involved |
---|---|---|
This compound (pOH-FBM) | Oxidation | CYP2C19, CYP3A4 |
2-Hydroxyfelbamate (2OH-FBM) | Oxidation | CYP2C19 |
Monocarboxylic Acid (MCF) | Hydrolysis | CES |
Reactive Metabolite (2-PP) | Formation | CYP and CES |
Anticonvulsant Activity
This compound exhibits anticonvulsant properties similar to those of its parent compound, felbamate. It acts as an antagonist at the glycine recognition site of the N-methyl-D-aspartate (NMDA) receptor, which is crucial in modulating excitatory neurotransmission. By inhibiting NMDA receptor activity, pOH-FBM helps in increasing seizure thresholds and reducing seizure spread.
Toxicological Profile
The metabolism of felbamate can lead to the formation of reactive metabolites such as 2-PP, which can cause hepatotoxicity. In studies involving chimeric mice with humanized livers, it was observed that administration of felbamate resulted in decreased levels of glutathione (GSH), indicating potential oxidative stress and liver damage due to reactive metabolite formation.
Case Studies and Research Findings
- Study on Hepatotoxicity : In a study using PXB-mice, administration of 600 mg/kg of felbamate resulted in significant alterations in liver enzyme levels, suggesting hepatotoxic effects linked to the reactive metabolite 2-PP. The study highlighted the importance of understanding individual metabolic differences due to genetic polymorphisms in CYP and CES enzymes that could influence drug metabolism and toxicity outcomes .
- Clinical Observations : A retrospective analysis involving patients treated with felbamate showed varying responses attributed to differences in metabolic pathways. Patients with specific genetic variants exhibited altered drug clearance rates and were more susceptible to adverse effects .
- Pharmacokinetics : Research indicated that over 90% of felbamate is absorbed after oral administration, with this compound being one of the primary metabolites detected in plasma samples from patients undergoing treatment for epilepsy .
Q & A
Basic Research Questions
Q. What are the standard protocols for synthesizing and characterizing p-Hydroxyfelbamate with high purity?
- Methodological Answer : Synthesis typically involves multi-step organic reactions, such as esterification or hydroxylation of precursor compounds. Key steps include:
- Purification : Column chromatography (e.g., silica gel) or recrystallization using solvents like ethanol/water mixtures.
- Characterization : Use NMR (¹H/¹³C) for structural confirmation, HPLC for purity assessment (>98%), and mass spectrometry for molecular weight validation.
- Documentation : Provide detailed reaction conditions (temperature, catalysts) and spectroscopic data in supplementary materials to ensure reproducibility .
Q. Which analytical methods are validated for quantifying this compound in biological matrices?
- Methodological Answer : Liquid chromatography-tandem mass spectrometry (LC-MS/MS) is preferred due to its sensitivity (LOD: 0.1 ng/mL) and specificity. Key validation parameters include:
- Linearity : Over a concentration range of 1–1000 ng/mL (R² > 0.99).
- Recovery : Assessed via spiked plasma/serum samples (recovery rates 85–115%).
- Matrix Effects : Evaluate ion suppression/enhancement using post-column infusion .
Q. How should researchers design in vitro assays to evaluate this compound’s metabolic stability?
- Methodological Answer :
- Hepatic Microsomal Incubations : Use human liver microsomes (HLM) with NADPH cofactor, incubate at 37°C, and sample at 0, 15, 30, and 60 minutes.
- Quantification : Measure parent compound depletion via LC-MS. Calculate half-life (t₁/₂) using first-order kinetics.
- Controls : Include positive controls (e.g., verapamil) and blank matrices to account for non-enzymatic degradation .
Advanced Research Questions
Q. How can contradictions in reported pharmacokinetic (PK) data for this compound be systematically addressed?
- Methodological Answer : Contradictions often arise from variability in experimental design:
- Source Analysis : Compare dosing regimens (e.g., single vs. repeated doses), species differences (rodent vs. human PK models), and analytical methodologies (e.g., ELISA vs. LC-MS).
- Statistical Reconciliation : Apply meta-analysis to pooled data, adjusting for covariates like body weight, renal clearance, and protein binding .
- In Silico Modeling : Use physiologically based pharmacokinetic (PBPK) models to simulate interspecies differences and identify critical parameters (e.g., Vd, CL) .
Q. What experimental strategies are recommended to elucidate this compound’s metabolic pathways and reactive intermediates?
- Methodological Answer :
- Isotopic Labeling : Use ¹⁴C-labeled this compound to track metabolite formation in hepatocyte incubations.
- Reactive Intermediate Trapping : Add glutathione (GSH) or potassium cyanide (KCN) to incubations to capture electrophilic intermediates (e.g., quinone methides).
- High-Resolution MS : Employ HRMS/MS (Q-TOF) for structural elucidation of Phase I/II metabolites and adducts .
Q. How should researchers design a study to assess this compound’s potential off-target effects in neurological systems?
- Methodological Answer :
- In Vitro Screening : Use primary neuronal cultures or iPSC-derived neurons to evaluate cytotoxicity (MTT assay) and calcium signaling (Fluo-4 imaging).
- Transcriptomics : Perform RNA-seq to identify dysregulated pathways (e.g., oxidative stress, apoptosis).
- In Vivo Validation : Conduct behavioral tests (e.g., rotarod, open field) in rodent models, paired with CSF sampling for biomarker analysis (e.g., GFAP, neurofilament light chain) .
Q. Data Analysis & Reproducibility
Q. What statistical approaches are critical for analyzing dose-response relationships in this compound toxicity studies?
- Methodological Answer :
- Nonlinear Regression : Fit data to sigmoidal models (e.g., Hill equation) to calculate EC₅₀/IC₅₀ values.
- Bootstrap Resampling : Estimate confidence intervals for parameters to account for small sample sizes.
- ANCOVA : Adjust for covariates like animal weight or baseline biomarker levels .
Q. How can researchers ensure reproducibility when reporting this compound’s enzyme inhibition kinetics?
- Methodological Answer :
- Detailed Protocols : Specify substrate concentrations (e.g., Km ± 10%), incubation times, and enzyme sources (e.g., recombinant CYP3A4 vs. HLM).
- Data Transparency : Publish raw kinetic plots (velocity vs. substrate) and inhibition constants (Ki) in supplementary materials.
- Cross-Validation : Compare results with published assays using reference inhibitors (e.g., ketoconazole for CYP3A4) .
Q. Literature & Contradiction Management
Q. What frameworks are effective for critically evaluating conflicting literature on this compound’s mechanism of action?
- Methodological Answer :
- Evidence Grading : Use the FINER criteria (Feasible, Interesting, Novel, Ethical, Relevant) to prioritize high-quality studies.
- Contradiction Mapping : Create a table comparing study designs, endpoints, and confounding variables (e.g., cell lines, exposure durations).
- Hypothesis Testing : Design follow-up experiments to test opposing mechanisms (e.g., competitive vs. non-competitive enzyme inhibition) .
Q. How should meta-analyses be structured to synthesize heterogeneous data on this compound’s efficacy across preclinical models?
- Methodological Answer :
- Inclusion Criteria : Define strict eligibility (e.g., peer-reviewed studies with ≥3 replicates).
- Effect Size Calculation : Use standardized mean difference (SMD) for cross-model comparisons.
- Sensitivity Analysis : Exclude outliers and assess publication bias via funnel plots .
Eigenschaften
IUPAC Name |
[3-carbamoyloxy-2-(4-hydroxyphenyl)propyl] carbamate | |
---|---|---|
Source | PubChem | |
URL | https://pubchem.ncbi.nlm.nih.gov | |
Description | Data deposited in or computed by PubChem | |
InChI |
InChI=1S/C11H14N2O5/c12-10(15)17-5-8(6-18-11(13)16)7-1-3-9(14)4-2-7/h1-4,8,14H,5-6H2,(H2,12,15)(H2,13,16) | |
Source | PubChem | |
URL | https://pubchem.ncbi.nlm.nih.gov | |
Description | Data deposited in or computed by PubChem | |
InChI Key |
PXBZEVLDFAKFLV-UHFFFAOYSA-N | |
Source | PubChem | |
URL | https://pubchem.ncbi.nlm.nih.gov | |
Description | Data deposited in or computed by PubChem | |
Canonical SMILES |
C1=CC(=CC=C1C(COC(=O)N)COC(=O)N)O | |
Source | PubChem | |
URL | https://pubchem.ncbi.nlm.nih.gov | |
Description | Data deposited in or computed by PubChem | |
Molecular Formula |
C11H14N2O5 | |
Source | PubChem | |
URL | https://pubchem.ncbi.nlm.nih.gov | |
Description | Data deposited in or computed by PubChem | |
DSSTOX Substance ID |
DTXSID201344225 | |
Record name | p-Hydroxyfelbamate | |
Source | EPA DSSTox | |
URL | https://comptox.epa.gov/dashboard/DTXSID201344225 | |
Description | DSSTox provides a high quality public chemistry resource for supporting improved predictive toxicology. | |
Molecular Weight |
254.24 g/mol | |
Source | PubChem | |
URL | https://pubchem.ncbi.nlm.nih.gov | |
Description | Data deposited in or computed by PubChem | |
CAS No. |
109482-28-2 | |
Record name | p-Hydroxyfelbamate | |
Source | EPA DSSTox | |
URL | https://comptox.epa.gov/dashboard/DTXSID201344225 | |
Description | DSSTox provides a high quality public chemistry resource for supporting improved predictive toxicology. | |
Haftungsausschluss und Informationen zu In-Vitro-Forschungsprodukten
Bitte beachten Sie, dass alle Artikel und Produktinformationen, die auf BenchChem präsentiert werden, ausschließlich zu Informationszwecken bestimmt sind. Die auf BenchChem zum Kauf angebotenen Produkte sind speziell für In-vitro-Studien konzipiert, die außerhalb lebender Organismen durchgeführt werden. In-vitro-Studien, abgeleitet von dem lateinischen Begriff "in Glas", beinhalten Experimente, die in kontrollierten Laborumgebungen unter Verwendung von Zellen oder Geweben durchgeführt werden. Es ist wichtig zu beachten, dass diese Produkte nicht als Arzneimittel oder Medikamente eingestuft sind und keine Zulassung der FDA für die Vorbeugung, Behandlung oder Heilung von medizinischen Zuständen, Beschwerden oder Krankheiten erhalten haben. Wir müssen betonen, dass jede Form der körperlichen Einführung dieser Produkte in Menschen oder Tiere gesetzlich strikt untersagt ist. Es ist unerlässlich, sich an diese Richtlinien zu halten, um die Einhaltung rechtlicher und ethischer Standards in Forschung und Experiment zu gewährleisten.