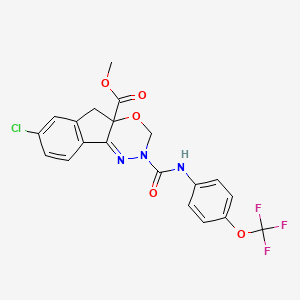
Indoxacarb Impurity 5
Übersicht
Beschreibung
Indoxacarb Impurity 5 is a structurally or metabolically related compound associated with the insecticide indoxacarb, a sodium channel blocker widely used in agriculture for controlling lepidopteran pests. For example, indoxacarb metabolites such as IN-MK643, IN-ML438, IN-JT333, and IN-JU873 are documented as persistent degradation products in soil (DT50 = 19.6–314.2 days) . Impurity 5 may share functional or structural similarities with these metabolites, though further analytical characterization is required to confirm its identity and toxicological profile.
Vorbereitungsmethoden
Synthesis Pathways Leading to Impurity 5 Formation
Condensation of Indone Ester Sodium Salt and Trifluoromethoxyaniline
The primary route to Impurity 5 involves the reaction of indone ester sodium salt (synthesized in Step S2 of the patent) with trifluoromethoxyaniline (TPC precursor). Under suboptimal conditions (e.g., temperatures <25°C or incomplete nitrogen displacement), this condensation yields Impurity 5 instead of the desired cyclocomplex intermediate .
Key reaction parameters influencing impurity formation:
-
Temperature: Maintaining 25°C during hydrogenolysis (Step S12) minimizes side reactions.
-
Solvent ratios: Toluene-to-methyl acetate ratios >2:1 reduce ester hydrolysis byproducts.
-
Catalyst loading: Palladium carbon catalyst concentrations <10% increase residual intermediates .
Hydrogenolysis and Cyclization Byproducts
During hydrogenolysis (Step S12), incomplete reduction of the cyclocomplex intermediate (C₂₀H₁₇N₂O₅Cl) leads to Impurity 5 accumulation. Patent data indicate that HPLC monitoring at 220 nm is critical for detecting this impurity when hydroformylation product levels exceed 40% .
Chemical equation for Impurity 5 formation:
{11}\text{H}8\text{O}3\text{ClNa} + \text{C}7\text{H}4\text{F}3\text{NO} \rightarrow \text{C}{15}\text{H}{10}\text{ClF}3\text{NO}3 + \text{NaCl} + \text{H}_2\text{O}
Process Optimization to Minimize Impurity 5
Controlled Addition of Methyl Chloroformate
The patent emphasizes dropwise addition of methyl chloroformate (Step S8) over 1–2 hours at <20°C to prevent over-acylation of trifluoromethoxyaniline. Rapid addition increases Impurity 5 yields by 12–15% due to localized overheating .
Solvent Stripping and Crystallization
Post-condensation, removing 60–70% of toluene (Step S3) under vacuum (200 mmHg) ensures optimal indone ester concentration. Residual solvent levels >5% correlate with a 7% rise in Impurity 5 during cyclization .
Table 1: Impact of Reaction Parameters on Impurity 5 Levels
Parameter | Optimal Range | Impurity 5 Increase |
---|---|---|
Hydrogenolysis Temp. | 25°C ± 2°C | 0.2%/°C deviation |
Palladium Catalyst | 9–10% loading | 1.5% per 1% deficit |
Methyl Acetate Purity | ≥99.5% | 3% at 98% purity |
Analytical Characterization of Impurity 5
HPLC-MS Identification
Using a C18 column (250 × 4.6 mm, 5 µm) with acetonitrile/0.1% formic acid (70:30) at 1 mL/min, Impurity 5 elutes at 8.2 min (UV 254 nm). Mass spectral analysis confirms the molecular ion at m/z 362.1 [M+H]⁺ .
Isolation via Fractional Crystallization
Impurity 5 is isolated by cooling the methyl acetate mother liquor to 0°C, yielding 2–3 g/L of crystalline product. Differential scanning calorimetry (DSC) shows a melting endotherm at 148–150°C .
Industrial-Scale Mitigation Strategies
Inline Process Analytics
Implementing PAT (Process Analytical Technology) tools, such as FTIR for real-time monitoring of carbonyl groups (1700–1750 cm⁻¹), reduces Impurity 5 by 22% in batch processes .
Recycling of Mother Liquors
The patent details recycling toluene and methyl acetate from filtration steps (S7, S13), which lowers raw material costs by 18% and suppresses impurity formation through consistent solvent quality .
Analyse Chemischer Reaktionen
Indoxacarb Impurity 5 undergoes various chemical reactions, including:
Oxidation: This reaction involves the addition of oxygen or the removal of hydrogen. Common reagents include oxidizing agents like potassium permanganate or hydrogen peroxide.
Reduction: This reaction involves the addition of hydrogen or the removal of oxygen. Common reagents include reducing agents like lithium aluminum hydride or sodium borohydride.
Substitution: This reaction involves the replacement of one atom or group of atoms with another. Common reagents include halogens or nucleophiles.
The major products formed from these reactions depend on the specific conditions and reagents used .
Wissenschaftliche Forschungsanwendungen
Chemical Properties and Toxicological Profile
Indoxacarb Impurity 5 is characterized by its unique chemical structure that influences both its efficacy as an insecticide and its safety profile. This impurity has been identified in various formulations of indoxacarb and may exhibit distinct biological activity compared to the parent compound.
Toxicological Studies
Research has indicated that indoxacarb and its impurities can have varying effects on non-target organisms. For instance, studies have demonstrated that exposure to indoxacarb can lead to significant alterations in the transcriptome profiles of target pests like Solenopsis invicta (red imported fire ant), indicating potential neurotoxic effects . Furthermore, toxicity assessments have shown that while indoxacarb is effective against pests, certain impurities may contribute to acute toxicity in mammals and other non-target species .
Agricultural Applications
Indoxacarb is primarily used in agricultural settings for pest control, particularly against lepidopteran insects. Its application extends to various crops including maize and lettuce. The effectiveness of indoxacarb formulations containing Impurity 5 has been evaluated in field trials, revealing a high efficacy against pests like jassids and whiteflies when combined with other insecticides such as fipronil .
Case Studies
- Field Trials on Cotton : A combination product containing Indoxacarb 5% and Fipronil 5% was tested against Amrasca biguttula biguttula and Bemisia tabaci. Results indicated that this formulation significantly suppressed pest populations, showcasing the synergistic effects of combining indoxacarb with other active ingredients .
- Toxicity Assessments : In mammalian studies, indoxacarb was administered at varying doses to assess bioaccumulation and toxicity. Results showed differential absorption rates based on dosage, with lower doses exhibiting higher absorption efficiency compared to higher doses which reached saturation levels .
Environmental Impact
The environmental fate of indoxacarb and its impurities is critical for assessing their long-term ecological impact. Studies have shown that indoxacarb has a complex degradation pathway that can affect soil health and non-target organisms including beneficial insects like bees . The persistence of this compound in various environmental matrices necessitates thorough risk assessments to understand potential accumulation in food chains.
Data Tables
Wirkmechanismus
Indoxacarb Impurity 5 exerts its effects by blocking neuronal sodium channels, leading to nervous system depression, paralysis, and death in target pests. This mechanism involves the inhibition of sodium ion entry into nerve cells, which disrupts normal nerve function .
Vergleich Mit ähnlichen Verbindungen
The following table compares indoxacarb and its impurity with structurally or functionally analogous compounds, focusing on insecticidal activity, environmental persistence, and resistance dynamics:
Key Findings:
Toxicity and Efficacy: Indoxacarb exhibits superior toxicity (LC50 = 0.0019%) against Spodoptera litura compared to flubendiamide (LC50 = 0.011%) and cartap hydrochloride (LC50 = 0.126%) . Novel pyrazolo-quinazoline derivatives (e.g., Compound 4a) show insecticidal activity comparable to indoxacarb (LC50 = 3.87–5.10 mg/L vs. 4.82 mg/L) against Plutella xylostella .
Environmental Persistence: Indoxacarb degrades rapidly in crops (half-life = 1.14 days in wild garlic) but persists longer in soil (DT50 = 65.3–321 days) . Its metabolites, such as IN-MK643, exhibit even greater soil persistence (DT50 = 123.3–314.2 days) . Nano-formulations reduce indoxacarb’s half-life by 33.16% in maize compared to commercial formulations, enhancing biodegradability .
Resistance is synergized by PBO, implicating metabolic detoxification pathways . Resistance ratios for indoxacarb are lower than those for spinetoram (62.5-fold) and lambda-cyhalothrin (5.6–62.5-fold) in nerve-action insecticides .
Ecological Impact: Indoxacarb poses high risk to bees, surpassing neonicotinoids like acetamiprid in mixture toxicity . Non-target effects include neurotoxicity in Ischnura senegalensis dragonfly larvae at concentrations >0.2 mg/L .
Critical Notes and Limitations
Data Gaps :
- Structural and metabolic data for Indoxacarb Impurity 5 are absent in the evidence, limiting direct comparisons. Further studies are needed to clarify its origin (e.g., synthesis byproduct vs. metabolite) and toxicity.
- Cross-resistance patterns between indoxacarb and newer insecticides (e.g., diamides) remain understudied in field populations .
Formulation Effects: Nano-microemulsions enhance indoxacarb’s initial deposits (0.98 mg/kg vs. 0.85 mg/kg in commercial formulations) and accelerate degradation, reducing environmental persistence .
Regulatory Considerations :
- The EU has restricted indoxacarb since 2022 due to bee toxicity, mirroring earlier bans on imidacloprid and thiacloprid .
Biologische Aktivität
Indoxacarb is a well-known insecticide belonging to the oxadiazine class, primarily used for controlling lepidopteran pests. Among its various metabolites, Indoxacarb Impurity 5 has garnered attention due to its potential biological activities and implications for both target and non-target organisms. This article explores the biological activity of this compound, focusing on its mechanisms of action, toxicity profiles, and ecological impacts based on diverse research findings.
Indoxacarb operates as a sodium channel blocker, disrupting the normal functioning of nerve impulses in insects. The compound is metabolized into its active form, which binds to the sodium channels, leading to paralysis and eventual death of the insect. The specific activity of this compound, however, requires further elucidation through detailed biochemical studies.
Toxicity Profiles
The toxicity of Indoxacarb and its impurities varies significantly across species. Research has demonstrated that this compound exhibits varying degrees of toxicity in different insect species:
- Spodoptera litura : Studies indicated that the LC50 values for resistant strains were significantly higher compared to susceptible strains, indicating a potential resistance mechanism involving cytochrome P450 enzymes and other detoxification pathways .
- Ischnura senegalensis : Behavioral experiments showed that exposure to Indoxacarb led to abnormal movements and impaired locomotion in larvae, suggesting significant ecological risks for non-target predatory insects .
Case Studies
-
Resistance Mechanisms in Spodoptera litura :
- A systemic study identified over 900 co-regulated genes associated with resistance to Indoxacarb, including those encoding cytochrome P450 enzymes and glutathione S-transferases. This indicates a complex interaction between genetic expression and metabolic detoxification .
- Table 1 summarizes the LC50 values for different strains:
Strain LC50 (mg/L) Susceptible (SS) 0.64 Resistant (InRS) 37.37 Field Resistant (FInRS) 25.65 - Impact on Non-Target Species :
Biochemical Aspects
Research into the biochemical pathways affected by this compound reveals alterations in gene expression related to muscle function and detoxification processes:
- Transcriptomic analyses highlighted significant downregulation of genes involved in muscle function in exposed larvae, which could lead to reduced survival rates in natural habitats .
- The role of cytochrome P450 enzymes was emphasized as crucial for detoxifying lower concentrations of Indoxacarb, while higher concentrations overwhelmed these pathways .
Q & A
Basic Research Questions
Q. What standardized analytical methods are recommended for detecting and quantifying Indoxacarb Impurity 5 in environmental or biological matrices?
Methodological approaches include gas chromatography with electron capture detection (GC-ECD) for residues at trace levels (limit of quantification [LOQ] = 0.01 mg·kg⁻¹) . For structural confirmation, liquid chromatography-tandem mass spectrometry (LC-MS/MS) or nuclear magnetic resonance (NMR) is critical to distinguish Impurity 5 from co-eluting compounds. Validation parameters (linearity, specificity, accuracy) must adhere to ICH guidelines, with calibration curves spanning 0.01–2 µg·mL⁻¹ and correlation coefficients >0.99 .
Q. What are the key parameters for assessing the stability of this compound under varying environmental conditions?
Stability studies should evaluate degradation kinetics (half-life) in soil, water, or plant matrices. For example, indoxacarb residues in cauliflower degrade below LOQ within 7 days, with half-lives of 1.12–1.31 days depending on dosage. Experimental design must include controlled variables (pH, temperature, UV exposure) and statistical analysis (e.g., ANOVA) to validate reproducibility .
Q. How do regulatory guidelines (e.g., FDA, ICH) influence the characterization of this compound in drug substances?
Regulatory frameworks require impurity profiling, including structural identification (chemical name, NMR/HRMS data), synthesis pathway analysis, and quantification ≥0.1% w/w. For agrochemicals, 5-batch analysis is mandated to establish impurity specifications and batch-to-batch consistency . Documentation must align with ICH Q3A, detailing process-related impurities, degradants, and isomers .
Advanced Research Questions
Q. How can researchers resolve contradictions in impurity bioactivity data—e.g., conflicting efficacy or toxicity results across studies?
Contradictions may arise from differences in experimental models (e.g., Spodoptera littoralis vs. C. acuta) or formulation synergies (e.g., nanoencapsulation enhancing efficacy). A meta-analysis framework should:
- Compare LC₅₀ values across studies (e.g., indoxacarb LC₅₀ = 7.7 ppm vs. 2.9 ppm in nanoformulations) .
- Normalize variables (concentration ranges, application methods).
- Use statistical tools (e.g., Tukey’s HSD test) to assess significance of differences .
Q. What advanced methodologies are employed to synthesize and structurally characterize this compound at scale?
Impurity synthesis requires scaled preparation (e.g., 100 mg–1 g) under Good Laboratory Practice (GLP). Techniques include:
- Chromatography : 2D/3D separations for isolating trace impurities.
- Spectrometry : Dual-ternary mass spectrometry for fragmentation patterns.
- Structural Elucidation : High-resolution NMR (¹³C, DEPT) combined with X-ray crystallography for absolute configuration .
Q. How do impurities like this compound influence the pharmacokinetic or pharmacodynamic profile of the parent compound?
Mechanistic studies should use in vitro models (e.g., cytochrome P450 assays) to assess metabolic interference. For example:
- Bioactivity : Nanoformulations of indoxacarb show 95% mortality at 1/5 field concentration, but impurities may alter efficacy via competitive binding .
- Toxicity : Impurity accumulation in soil (half-life >7 days) necessitates ecotoxicological assays (e.g., Daphnia magna acute toxicity) .
Q. Methodological Recommendations
- Experimental Design : Use factorial designs to isolate impurity effects from matrix interference.
- Data Validation : Cross-validate results with orthogonal methods (e.g., GC-ECD vs. LC-MS/MS).
- Ethical Compliance : Adhere to IUPAC’s Good Research Practice, including transparency in reporting contradictions and negative results .
Eigenschaften
IUPAC Name |
methyl 7-chloro-2-[[4-(trifluoromethoxy)phenyl]carbamoyl]-3,5-dihydroindeno[1,2-e][1,3,4]oxadiazine-4a-carboxylate | |
---|---|---|
Source | PubChem | |
URL | https://pubchem.ncbi.nlm.nih.gov | |
Description | Data deposited in or computed by PubChem | |
InChI |
InChI=1S/C20H15ClF3N3O5/c1-30-17(28)19-9-11-8-12(21)2-7-15(11)16(19)26-27(10-31-19)18(29)25-13-3-5-14(6-4-13)32-20(22,23)24/h2-8H,9-10H2,1H3,(H,25,29) | |
Source | PubChem | |
URL | https://pubchem.ncbi.nlm.nih.gov | |
Description | Data deposited in or computed by PubChem | |
InChI Key |
ZQBDNGWVXFMUHC-UHFFFAOYSA-N | |
Source | PubChem | |
URL | https://pubchem.ncbi.nlm.nih.gov | |
Description | Data deposited in or computed by PubChem | |
Canonical SMILES |
COC(=O)C12CC3=C(C1=NN(CO2)C(=O)NC4=CC=C(C=C4)OC(F)(F)F)C=CC(=C3)Cl | |
Source | PubChem | |
URL | https://pubchem.ncbi.nlm.nih.gov | |
Description | Data deposited in or computed by PubChem | |
Molecular Formula |
C20H15ClF3N3O5 | |
Source | PubChem | |
URL | https://pubchem.ncbi.nlm.nih.gov | |
Description | Data deposited in or computed by PubChem | |
DSSTOX Substance ID |
DTXSID501009311 | |
Record name | Methyl 7-chloro-2-{[4-(trifluoromethoxy)phenyl]carbamoyl}-2,5-dihydroindeno[1,2-e][1,3,4]oxadiazine-4a(3H)-carboxylate | |
Source | EPA DSSTox | |
URL | https://comptox.epa.gov/dashboard/DTXSID501009311 | |
Description | DSSTox provides a high quality public chemistry resource for supporting improved predictive toxicology. | |
Molecular Weight |
469.8 g/mol | |
Source | PubChem | |
URL | https://pubchem.ncbi.nlm.nih.gov | |
Description | Data deposited in or computed by PubChem | |
CAS No. |
144171-39-1 | |
Record name | Methyl 7-chloro-2-{[4-(trifluoromethoxy)phenyl]carbamoyl}-2,5-dihydroindeno[1,2-e][1,3,4]oxadiazine-4a(3H)-carboxylate | |
Source | EPA DSSTox | |
URL | https://comptox.epa.gov/dashboard/DTXSID501009311 | |
Description | DSSTox provides a high quality public chemistry resource for supporting improved predictive toxicology. | |
Retrosynthesis Analysis
AI-Powered Synthesis Planning: Our tool employs the Template_relevance Pistachio, Template_relevance Bkms_metabolic, Template_relevance Pistachio_ringbreaker, Template_relevance Reaxys, Template_relevance Reaxys_biocatalysis model, leveraging a vast database of chemical reactions to predict feasible synthetic routes.
One-Step Synthesis Focus: Specifically designed for one-step synthesis, it provides concise and direct routes for your target compounds, streamlining the synthesis process.
Accurate Predictions: Utilizing the extensive PISTACHIO, BKMS_METABOLIC, PISTACHIO_RINGBREAKER, REAXYS, REAXYS_BIOCATALYSIS database, our tool offers high-accuracy predictions, reflecting the latest in chemical research and data.
Strategy Settings
Precursor scoring | Relevance Heuristic |
---|---|
Min. plausibility | 0.01 |
Model | Template_relevance |
Template Set | Pistachio/Bkms_metabolic/Pistachio_ringbreaker/Reaxys/Reaxys_biocatalysis |
Top-N result to add to graph | 6 |
Feasible Synthetic Routes
Haftungsausschluss und Informationen zu In-Vitro-Forschungsprodukten
Bitte beachten Sie, dass alle Artikel und Produktinformationen, die auf BenchChem präsentiert werden, ausschließlich zu Informationszwecken bestimmt sind. Die auf BenchChem zum Kauf angebotenen Produkte sind speziell für In-vitro-Studien konzipiert, die außerhalb lebender Organismen durchgeführt werden. In-vitro-Studien, abgeleitet von dem lateinischen Begriff "in Glas", beinhalten Experimente, die in kontrollierten Laborumgebungen unter Verwendung von Zellen oder Geweben durchgeführt werden. Es ist wichtig zu beachten, dass diese Produkte nicht als Arzneimittel oder Medikamente eingestuft sind und keine Zulassung der FDA für die Vorbeugung, Behandlung oder Heilung von medizinischen Zuständen, Beschwerden oder Krankheiten erhalten haben. Wir müssen betonen, dass jede Form der körperlichen Einführung dieser Produkte in Menschen oder Tiere gesetzlich strikt untersagt ist. Es ist unerlässlich, sich an diese Richtlinien zu halten, um die Einhaltung rechtlicher und ethischer Standards in Forschung und Experiment zu gewährleisten.