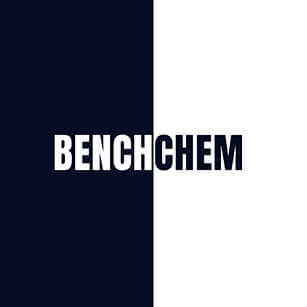
Clofentezine Metabolite 3
- Klicken Sie auf QUICK INQUIRY, um ein Angebot von unserem Expertenteam zu erhalten.
- Mit qualitativ hochwertigen Produkten zu einem WETTBEWERBSFÄHIGEN Preis können Sie sich mehr auf Ihre Forschung konzentrieren.
Übersicht
Beschreibung
Clofentezine Metabolite 3 is a derivative of clofentezine, a selective acaricide primarily used to control mite populations in agricultural settings. Clofentezine itself is known for its low aqueous solubility and moderate persistence in soil systems . The metabolite is formed through the biotransformation of clofentezine in various organisms and environmental conditions .
Vorbereitungsmethoden
Synthetic Routes and Reaction Conditions: The synthesis of Clofentezine Metabolite 3 typically involves the biotransformation of clofentezine in living organisms. This process can include hydroxylation, conjugation, and other metabolic pathways. Specific synthetic routes in a laboratory setting may involve controlled oxidation or reduction reactions to mimic these natural processes .
Industrial Production Methods: Industrial production of this compound is not commonly practiced due to its nature as a metabolite. Instead, it is often studied in the context of environmental fate and residue analysis following the application of clofentezine in agricultural settings .
Analyse Chemischer Reaktionen
Types of Reactions: Clofentezine Metabolite 3 can undergo various chemical reactions, including:
Oxidation: Introduction of hydroxyl groups.
Reduction: Conversion of ketones or aldehydes to alcohols.
Substitution: Replacement of functional groups with others, such as halogens.
Common Reagents and Conditions: Common reagents used in these reactions include oxidizing agents like potassium permanganate or hydrogen peroxide, and reducing agents like sodium borohydride. Reaction conditions often involve controlled temperatures and pH levels to ensure specificity and yield .
Major Products Formed: The major products formed from these reactions depend on the specific pathways and conditions used. For example, hydroxylation can lead to the formation of hydroxy-clofentezine derivatives, while reduction can yield alcohol derivatives .
Wissenschaftliche Forschungsanwendungen
Metabolism and Toxicology Studies
Absorption and Distribution:
Research indicates that after oral administration, a significant portion of clofentezine is excreted through feces (approximately 60-70% within 24 hours) with minimal unchanged substance found in urine . Studies on different species, including rats and baboons, show that the metabolite distribution varies, with higher concentrations found in adipose tissues, liver, and kidneys .
Toxicological Assessments:
Toxicity studies have indicated that Clofentezine Metabolite 3 exhibits low acute toxicity levels. Chronic exposure studies have shown no significant adverse effects on liver enzymes or overall health at lower doses, suggesting a favorable safety profile for agricultural use .
Agricultural Applications
This compound can be utilized effectively in integrated pest management (IPM) strategies due to its specific action against spider mites without affecting beneficial insects significantly. The following table summarizes its applications:
Application | Description |
---|---|
Pest Control | Effective against spider mites in crops such as apples, citrus, and vegetables. |
Resistance Management | Part of rotation strategies to manage resistance development in pest populations. |
Environmental Safety | Lower toxicity to non-target species compared to other pesticides. |
Efficacy in Crop Protection
A study conducted on apple orchards demonstrated that the application of clofentezine resulted in a significant reduction in spider mite populations while maintaining the population of predatory mites . This highlights its selective action and potential role in sustainable agriculture.
Impact on Non-target Species
Research assessing the impact of this compound on beneficial insects found minimal effects on lady beetles and lacewings, crucial for biological control . This finding supports its use as a safer alternative to broader-spectrum insecticides.
Environmental Fate and Residue Studies
Studies have shown that this compound has a relatively short half-life in soil and water environments, indicating low persistence and reduced risk of accumulation . Monitoring programs have been implemented to assess residue levels in crops post-application, confirming compliance with safety standards set by regulatory bodies.
Wirkmechanismus
The mechanism of action of Clofentezine Metabolite 3 involves its interaction with specific molecular targets in mites. It primarily acts as an ovicide, disrupting the development of mite eggs and early motile stages. The exact molecular pathways include inhibition of mitochondrial function and interference with cellular respiration .
Vergleich Mit ähnlichen Verbindungen
Similar Compounds:
Clofentezine: The parent compound, used as an acaricide.
3-Hydroxy-Clofentezine: Another metabolite formed through hydroxylation.
Mono-Chloro Sulfur-Containing Compound: A minor metabolite with distinct chemical properties.
Uniqueness: Clofentezine Metabolite 3 is unique due to its specific formation pathways and the distinct biological activity it exhibits compared to other metabolites. Its role in the environmental fate of clofentezine and its impact on non-target organisms make it a compound of significant interest in regulatory and environmental studies .
Biologische Aktivität
Clofentezine Metabolite 3 is a significant derivative of clofentezine, an acaricide predominantly used in agriculture to manage mite populations. Understanding the biological activity of this metabolite is crucial for assessing its environmental impact, efficacy, and potential health risks. This article compiles findings from various studies, including metabolic pathways, mechanisms of action, and toxicological assessments.
1. Overview of Clofentezine and Its Metabolite
Clofentezine is primarily recognized for its selective action against mites, functioning mainly as an ovicide. Its metabolites, particularly this compound, are formed through biotransformation processes within organisms exposed to the parent compound. These transformations typically involve hydroxylation and conjugation reactions facilitated by cytochrome P450 enzymes .
2. Metabolic Pathways
The metabolism of clofentezine has been extensively studied, revealing multiple pathways leading to various metabolites:
- Hydroxylation : This is a major pathway where hydroxyl groups are introduced at positions 3, 4, or 5 on the phenyl ring of clofentezine.
- Conjugation : Hydroxylated metabolites may undergo further conjugation, resulting in more polar compounds that are excreted via urine or feces .
Table 1: Major Metabolites of Clofentezine
Metabolite | Structure | Formation Pathway |
---|---|---|
Clofentezine | C₁₄H₁₁ClN₂O | Parent compound |
Clofentezine Metabolite 1 | C₁₄H₁₂ClN₂O₂ | Hydroxylation |
This compound | C₁₄H₁₂ClN₂O₂ | Hydroxylation and conjugation |
This compound primarily exhibits its biological activity through the following mechanisms:
- Ovicide Activity : It disrupts the development of mite eggs and early motile stages by inhibiting mitochondrial function.
- Cellular Respiration Interference : The metabolite affects cellular respiration pathways in mites, leading to reduced viability .
4. Toxicological Assessments
Toxicological studies have evaluated the safety profile of Clofentezine and its metabolites in various animal models:
- Acute Toxicity : Studies indicate low acute toxicity levels in mammals when exposed to clofentezine at recommended agricultural doses.
- Chronic Exposure : Long-term studies have shown reversible hepatotoxic effects at high concentrations but no significant impacts on mortality or major organ function at lower doses .
Case Study: Lactating Cow Experiment
In a study involving lactating cows administered with radiolabeled clofentezine, residues were analyzed to understand metabolic patterns:
- Dosing : Cows received oral doses of 2.2 mg/kg body weight per day for three days.
- Findings : Residues peaked in milk at 0.18 mg equiv/L. Analysis revealed that clofentezine was the major component found in tissues such as liver (33% residue), with hydroxylated derivatives also present .
5. Environmental Impact and Residue Analysis
Research has focused on the environmental fate of clofentezine and its metabolites:
- Soil Mobility : Studies indicate that metabolites tend to be less mobile than the parent compound, suggesting a lower risk of groundwater contamination.
- Residue Persistence : Residues from agricultural applications have been detected in various crops, necessitating monitoring for food safety compliance .
Table 2: Residue Levels in Various Tissues
Tissue Type | Percentage Residue (%) |
---|---|
Milk | 0.18 |
Liver | 33 |
Fat | 70 |
Muscle | 34 |
Eggs (developing) | 32 |
6. Conclusion
This compound plays a crucial role in understanding the biological activity and environmental implications of clofentezine usage in agriculture. Its mechanisms as an ovicide highlight its effectiveness against mite populations while also raising considerations regarding potential residues in food products and environmental systems. Ongoing research is essential to fully elucidate its metabolic pathways and long-term effects on health and ecosystems.
Eigenschaften
CAS-Nummer |
107573-63-7 |
---|---|
Molekularformel |
C15H11ClN4OS |
Molekulargewicht |
330.8 |
Reinheit |
> 95% |
Menge |
Milligrams-Grams |
Herkunft des Produkts |
United States |
Haftungsausschluss und Informationen zu In-Vitro-Forschungsprodukten
Bitte beachten Sie, dass alle Artikel und Produktinformationen, die auf BenchChem präsentiert werden, ausschließlich zu Informationszwecken bestimmt sind. Die auf BenchChem zum Kauf angebotenen Produkte sind speziell für In-vitro-Studien konzipiert, die außerhalb lebender Organismen durchgeführt werden. In-vitro-Studien, abgeleitet von dem lateinischen Begriff "in Glas", beinhalten Experimente, die in kontrollierten Laborumgebungen unter Verwendung von Zellen oder Geweben durchgeführt werden. Es ist wichtig zu beachten, dass diese Produkte nicht als Arzneimittel oder Medikamente eingestuft sind und keine Zulassung der FDA für die Vorbeugung, Behandlung oder Heilung von medizinischen Zuständen, Beschwerden oder Krankheiten erhalten haben. Wir müssen betonen, dass jede Form der körperlichen Einführung dieser Produkte in Menschen oder Tiere gesetzlich strikt untersagt ist. Es ist unerlässlich, sich an diese Richtlinien zu halten, um die Einhaltung rechtlicher und ethischer Standards in Forschung und Experiment zu gewährleisten.